Missile Fins 3D Printed with High-Strength Materials
목차
Introduction: The Critical Role of Missile Fins in Aerospace & Defense
Missiles, whether deployed for tactical advantage on the battlefield, strategic deterrence, or even peaceful space exploration launch systems, represent the pinnacle of aerospace engineering. Within these complex systems, every component plays a vital role, often operating under extreme conditions of speed, temperature, and stress. Among the most crucial external components are the missile fins. These aerodynamic surfaces, typically located at the aft section of the missile body, are fundamental to ensuring stability, maneuverability, and overall mission success. Their primary function is to interact with the airflow around the missile, generating aerodynamic forces that control the vehicle’s trajectory and orientation. Without precisely designed and manufactured fins, a missile would be uncontrollable, unable to maintain its intended flight path or accurately engage its target.
The importance of missile fins stems from several key aerodynamic principles:
- 안정성: Fins provide passive aerodynamic stability, much like the feathers on an arrow. They help to keep the missile aligned with the direction of flight, counteracting disturbances caused by atmospheric conditions, thrust vector misalignments, or slight asymmetries in the missile body. This stability is crucial for predictable flight and accurate guidance. The center of pressure (the point where the aerodynamic forces effectively act) must be located behind the center of gravity for the missile to be inherently stable. Fins achieve this by adding significant surface area at the rear.
- 제어: In many missile designs, fins are actively controlled, meaning they can be moved or actuated during flight. By changing the angle of the fins relative to the airflow (deflection), the missile’s control system can generate specific aerodynamic forces and moments. These forces are used to steer the missile, correct its trajectory, execute maneuvers, and maintain roll stability. The responsiveness and precision of these control surfaces directly impact the missile’s agility and its ability to intercept targets or follow complex flight paths.
- Maneuverability: Especially for air-to-air or surface-to-air missiles designed to intercept fast-moving, agile targets, high maneuverability is essential. Fins enable rapid changes in direction by generating large control forces. The size, shape (planform), and deflection capability of the fins are critical design parameters that determine the maximum g-loading (acceleration) the missile can withstand during maneuvers.
The operational environment for missile fins is incredibly demanding. They are subjected to:
- Extreme Aerodynamic Loads: At supersonic and hypersonic speeds (Mach 5 and above), the pressure and shear forces acting on the fins can be immense. The structure must withstand these loads without failure or excessive deformation.
- Aerodynamic Heating: Air friction at high speeds generates significant heat, particularly along the leading edges of the fins. Materials must retain their strength and structural integrity at elevated temperatures, sometimes exceeding hundreds or even thousands of degrees Celsius, depending on the speed and altitude regime.
- Vibration and Flutter: The interaction between aerodynamic forces, structural elasticity, and inertia can lead to aeroelastic phenomena like flutter – a potentially catastrophic self-excited vibration. Fin design must carefully consider stiffness and damping characteristics to avoid such issues.
- Impact and Erosion: Depending on the flight profile, fins might be exposed to rain, hail, dust, or debris, requiring resistance to erosion and impact damage.
Given these stringent requirements, the design, material selection, and manufacturing process for missile fins are critical considerations for aerospace and defense organizations. Traditionally, fins have been manufactured using subtractive methods like CNC machining from billet materials (e.g., high-strength steels, titanium alloys, or aluminum alloys) or, in some cases, through casting or forging followed by machining. While effective, these traditional methods can face limitations, especially when dealing with increasingly complex geometries driven by advanced aerodynamic requirements, the need for lightweighting to improve range and payload capacity, or the desire for rapid iteration during development and testing phases. The quest for higher performance, faster development cycles, and potentially lower costs for specialized, low-volume components has paved the way for exploring advanced manufacturing techniques, with metal additive manufacturing (AM), or 3D 프린팅, emerging as a powerful alternative for producing these critical aerospace components. The ability to build complex shapes layer-by-layer directly from digital designs offers unique advantages that align perfectly with the evolving demands of modern missile systems. Companies specializing in 금속 3D 프린팅 are increasingly becoming key partners for defense contractors and aerospace OEMs looking to leverage this technology for demanding applications like missile fins.
Applications: Where are Advanced Missile Fins Deployed?
The utility and adaptability of missile fins, enabled by advanced design and manufacturing, mean they are integral components across a wide spectrum of aerospace and defense systems. Their specific design – shape, size, actuation mechanism (fixed or movable), and material composition – varies significantly depending on the mission profile, speed regime, and operational requirements of the platform. Understanding these diverse applications highlights the critical need for manufacturing processes that can deliver high-performance, reliable, and often customized fin solutions.
Key Application Areas:
- Tactical Missiles: This broad category includes air-to-air, surface-to-air, air-to-surface, and anti-ship missiles.
- Air-to-Air Missiles (AAMs): Designed for high agility to intercept maneuvering aircraft. Fins must enable rapid, high-g turns. Examples include Sidewinder (AIM-9X) or AMRAAM (AIM-120). Materials need high strength-to-weight ratios and the ability to withstand significant aerodynamic loads. Control fins are typically actuated for precise maneuvering.
- Surface-to-Air Missiles (SAMs): Used to defend against aircraft and incoming missiles. Often larger than AAMs, they require robust fins for stability during boost phase and maneuverability during intercept. Examples: Patriot (MIM-104), Standard Missile (SM-6). Fins may experience high heating during ascent through dense atmosphere.
- Air-to-Surface Missiles (ASMs) / Guided Bombs: Focus on precision strikes against ground or sea targets. While high-g maneuvering might be less critical than for AAMs, fins ensure accurate guidance to the target. Stability during release and flight is key. Examples: Maverick (AGM-65), Hellfire (AGM-114), JDAM (Joint Direct Attack Munition) tail kits.
- Anti-Ship Missiles (AShMs): Often fly low (“sea-skimming”) profiles. Fins must provide stability and control in dense air close to the water surface and potentially execute terminal maneuvers. Examples: Harpoon (AGM-84), Exocet.
- Strategic Missiles: This category includes Intercontinental Ballistic Missiles (ICBMs) and Submarine-Launched Ballistic Missiles (SLBMs).
- While the primary flight phase is ballistic (outside the atmosphere), fins or other aerodynamic control surfaces might be used during the boost phase for initial stabilization and trajectory shaping within the atmosphere. Materials must withstand extreme temperatures and stresses during launch and ascent. Fins on re-entry vehicles (RVs) might also be used for maneuvering within the atmosphere (MaRV – Maneuverable Re-entry Vehicle).
- Hypersonic Vehicles: Operating at speeds exceeding Mach 5, these systems (including hypersonic glide vehicles and cruise missiles) present extreme challenges for aerodynamic surfaces.
- Fins or functionally similar control surfaces must withstand incredibly high temperatures (requiring advanced materials like ceramic matrix composites or refractory metals in some cases) and extreme aerodynamic pressures. Precise control at these speeds is exceptionally difficult, placing immense demands on the design and manufacturing of control surfaces. Metal AM offers possibilities for creating complex internal cooling channels or using novel high-temperature alloys.
- Unmanned Aerial Vehicles (UAVs) / Drones: While not “missiles” in the traditional sense, many larger or high-performance UAVs utilize fin-like control surfaces on their tails (vertical and horizontal stabilizers) for stability and control, operating under similar aerodynamic principles. Additionally, munitions deployed 에서 UAVs often rely on fins for guidance. Metal AM can be beneficial for creating lightweight, structurally efficient control surfaces for long-endurance or high-performance UAVs.
- Guided Munitions and Rockets: This includes smaller guided rockets (e.g., APKWS), mortar rounds with guidance kits, and specialized artillery shells.
- Fins provide the necessary stability and control to guide these munitions accurately to their targets, significantly increasing effectiveness over unguided counterparts. Cost-effective manufacturing, even for complex fin designs (like wrap-around or pop-out fins), is crucial for these often high-volume applications, presenting opportunities for optimized AM processes.
Common Threads Across Applications:
- Need for Precision: Regardless of the type, accuracy is paramount. Fins must be manufactured to tight tolerances to ensure predictable aerodynamic performance and reliable control system interaction.
- 머티리얼 퍼포먼스: The chosen material must meet the specific demands of the flight environment – strength, stiffness, temperature resistance, fatigue life, and sometimes radar transparency or low observability characteristics.
- 복잡한 지오메트리: Modern aerodynamic designs often call for non-linear shapes, variable thickness, and potentially integrated features (like actuation mechanisms or sensors) that can be challenging or expensive to produce conventionally.
- Weight Optimization: Reducing weight is almost always a goal in aerospace to improve range, payload, speed, or maneuverability. Fins contribute to overall vehicle mass, making lightweighting efforts valuable.
The diverse and demanding nature of these applications underscores why aerospace and defense manufacturers, prime contractors, and aerospace material distributors are increasingly turning to advanced manufacturing techniques like metal 3D printing. The ability to produce highly complex, lightweight, high-strength fins using specialized materials like Ti-6Al-4V 또는 스칼말로이® directly addresses the evolving needs of these critical systems.
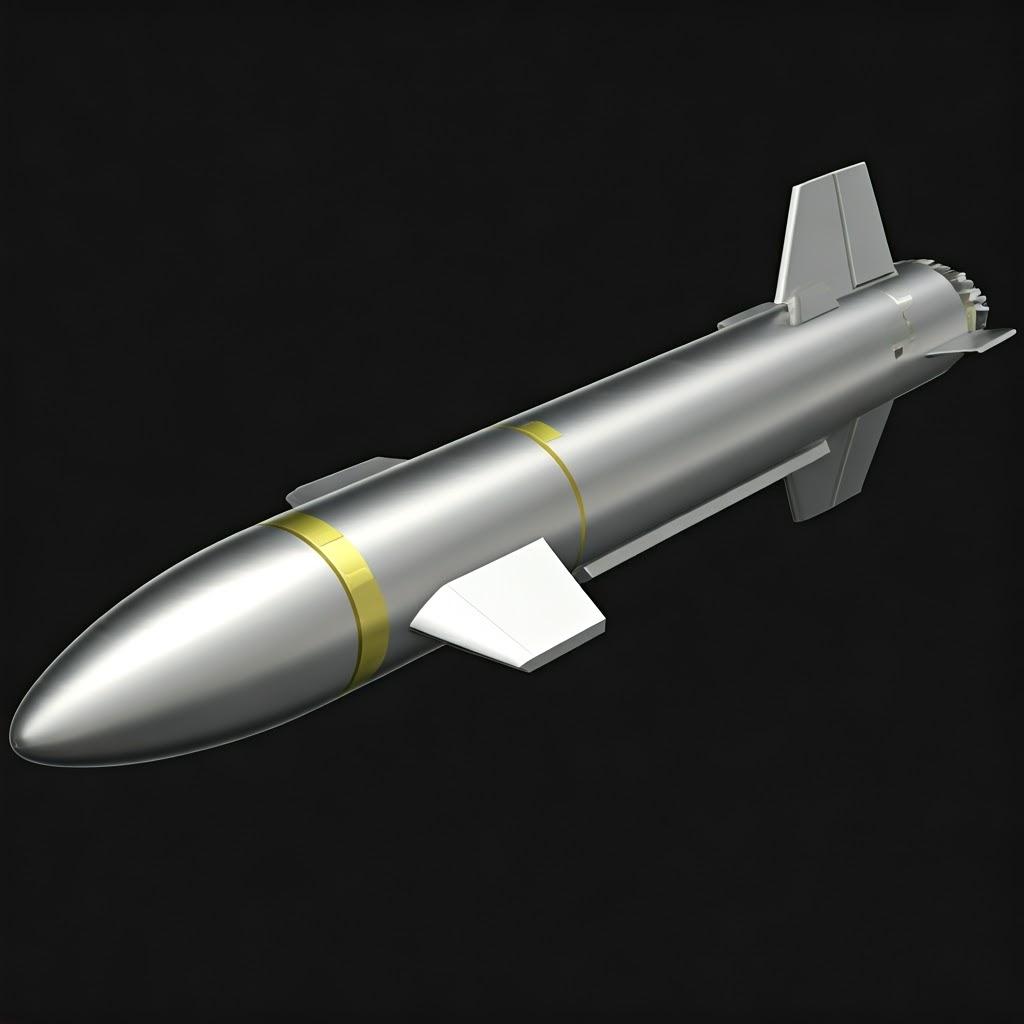
Why Metal 3D Printing for Missile Fin Production?
While traditional manufacturing methods like CNC machining, casting, and forging have long served the aerospace and defense industries well, metal additive manufacturing (AM), commonly known as metal 3D printing, presents a compelling suite of advantages specifically suited for producing complex, high-performance components like missile fins. The shift towards AM is driven by the need for enhanced performance, faster development cycles, supply chain flexibility, and the ability to realize designs previously considered unmanufacturable. For procurement managers and engineers evaluating production methods for critical defense hardware, understanding these benefits is crucial.
Key Advantages of Metal AM for Missile Fins:
- Geometric Complexity & Design Freedom:
- 도전: Modern aerodynamic requirements often lead to highly complex fin shapes (e.g., non-planar forms, optimized airfoils, blended root sections) that are difficult, time-consuming, or extremely expensive to achieve with subtractive machining (which involves cutting material away from a solid block).
- AM Solution: AM builds parts layer by layer directly from a 3D CAD model. This additive approach inherently allows for the creation of intricate internal and external features with relative ease. Designers can incorporate:
- Topology Optimized Shapes: Algorithms can determine the most efficient material distribution to withstand specific loads, resulting in organic-looking, lightweight yet strong structures impossible to machine conventionally.
- Internal Lattice Structures: For lightweighting while maintaining stiffness, complex internal lattices can be integrated within the fin’s volume.
- Conformal Cooling Channels (for Hypersonics): In ultra-high-speed applications, internal channels conforming to the fin’s shape could be printed for active cooling systems, a feat nearly impossible with traditional methods.
- 파트 통합: Features that might previously have required multiple machined parts and subsequent assembly (e.g., mounting brackets, actuator interfaces) can potentially be integrated directly into the 3D printed fin, reducing part count, assembly time, and potential failure points.
- 경량화:
- 도전: Every gram saved on a missile translates to potential gains in range, payload capacity, or maneuverability. Fins, while needing to be strong, contribute to the overall mass.
- AM Solution: The design freedom offered by AM enables significant weight reduction without compromising structural integrity. This is achieved through:
- 토폴로지 최적화: Placing material only where it’s structurally needed.
- 격자 구조: Replacing solid sections with strong, low-density lattices.
- Use of High Specific Strength Materials: AM processes excel with materials like Titanium alloys (Ti-6Al-4V) and specialized Aluminum alloys (Scalmalloy®), which offer excellent strength-to-weight ratios.
- 신속한 프로토타이핑 및 반복:
- 도전: Developing and testing new missile designs involves multiple iterations. Creating tooling (molds, dies) or complex machining setups for each prototype fin design using traditional methods is slow and costly.
- AM Solution: AM is a tool-less process. A new design can be sent directly to the printer, allowing engineers to produce functional prototypes in days rather than weeks or months. This accelerates the design-build-test cycle significantly, enabling faster innovation and optimization of aerodynamic performance. Design modifications can be implemented quickly by simply updating the CAD file.
- Material Efficiency (Reduced Waste):
- 도전: Subtractive manufacturing, especially for complex shapes from expensive materials like titanium, can generate substantial material waste (buy-to-fly ratio). Machining might start with a large billet and remove up to 80-90% of the material.
- AM Solution: AM uses material primarily where it’s needed in the final part. While some support structures are required and some powder is unfused, the overall material utilization is generally much higher compared to machining complex parts from billet, especially for expensive aerospace-grade powders. This is particularly relevant for wholesale metal powders procurement, where minimizing waste translates to cost savings.
- Low-Volume Production & On-Demand Manufacturing:
- 도전: Missile programs often involve relatively low production volumes compared to consumer goods. Setting up traditional production lines with dedicated tooling can be uneconomical for small batches or spare parts.
- AM Solution: AM is highly suitable for low-to-medium volume production runs without the high upfront costs associated with tooling. It also enables on-demand manufacturing, allowing organizations to print fins or spare parts as needed, reducing inventory requirements and improving supply chain resilience. A digital inventory of fin designs can be maintained and printed anywhere with the right equipment and certified processes.
- Tailored Material Properties:
- 도전: Achieving specific microstructures and material properties uniformly across a complex geometry can be challenging with traditional methods.
- AM Solution: AM processes, like Selective Laser Melting (SLM) or Electron Beam Melting (EBM), involve rapid melting and solidification. This can create unique microstructures, sometimes offering superior properties compared to wrought or cast equivalents, although post-processing like heat treatment is often required to optimize them. Companies like Met3dp, with expertise in both advanced powder development and printing processes, can help tailor material properties for specific application needs. Their focus on R&D allows for the exploration of novel alloys and processing parameters to push performance boundaries.
- Supply Chain Simplification & Security:
- 도전: Traditional aerospace supply chains can be complex and geographically dispersed, potentially posing risks (disruption, lead times, security).
- AM Solution: The ability to print parts closer to the point of need or assembly can simplify logistics. Furthermore, maintaining digital files instead of physical tooling enhances design security. Manufacturing can potentially be distributed across certified facilities, increasing redundancy.
While AM offers these compelling advantages, it’s important to note that it’s not a universal replacement for traditional methods. Factors like surface finish requirements (AM parts often need post-machining for critical surfaces), internal stress management, the need for rigorous quality control and certification (especially for flight-critical parts), and cost-effectiveness at very high volumes must be carefully considered. However, for components like missile fins, where complex geometry, lightweighting, rapid iteration, and high-performance materials are paramount, metal 3D printing provides capabilities that significantly advance design possibilities and manufacturing efficiency. Collaborating with experienced AM service providers who understand the nuances of aerospace applications is key to successfully leveraging this transformative technology.
High-Strength Materials for Demanding Flight Conditions: Ti-6Al-4V and Scalmalloy®
The extreme operating environment of missile fins – encompassing high aerodynamic loads, potential G-forces during maneuvers, significant vibrations, and often elevated temperatures due to aerodynamic heating – necessitates the use of materials that offer exceptional performance characteristics. The choice of material is a critical design decision, directly impacting the fin’s structural integrity, weight, durability, and ultimately, the missile’s overall effectiveness. Metal additive manufacturing processes have matured to reliably process high-performance alloys well-suited for these demanding aerospace and defense applications. Among the leading candidates for 3D printed missile fins are Titanium Grade 5 (Ti-6Al-4V) and Scalmalloy®, an advanced aluminum-magnesium-scandium alloy.
Understanding the properties and benefits of these materials is crucial for engineers designing the components and for procurement managers sourcing the materials or manufacturing services. Reputable 금속 분말 공급업체 like Met3dp play a vital role by providing high-quality, consistent powders optimized for AM processes. Met3dp, utilizing advanced techniques like gas atomization and Plasma Rotating Electrode Process (PREP), ensures its metallic powders, including Titanium alloys, possess the high sphericity and flowability required for producing dense, high-quality parts.
1. Titanium Grade 5 (Ti-6Al-4V): The Aerospace Workhorse
Ti-6Al-4V (also known as Ti64 or Grade 5 Titanium) is arguably the most widely used titanium alloy, accounting for more than 50% of all titanium tonnage worldwide. Its popularity stems from an excellent combination of properties that make it ideal for aerospace structures, including missile fins.
- Key Properties & Benefits:
- 높은 중량 대비 강도 비율: Titanium is about 40% lighter than steel but offers comparable or even superior strength in many conditions. This is critical for missile fins, where minimizing weight directly benefits performance metrics like range and maneuverability.
- 뛰어난 내식성: Ti-6Al-4V exhibits outstanding resistance to corrosion in a wide range of environments, including saltwater and various industrial chemicals. This ensures durability and longevity, even for missiles potentially stored for long periods or operated in marine environments.
- Good High-Temperature Performance: While not a refractory metal, Ti-6Al-4V retains significant strength at moderately elevated temperatures, typically up to around 315°C (600°F), and can withstand short excursions to higher temperatures. This is sufficient for many tactical missile applications operating at supersonic speeds. For hypersonic applications requiring resistance to much higher temperatures, other materials (like nickel superalloys or potentially refractory metals processed via AM) would be considered.
- High Fatigue Strength: Missile fins are subjected to cyclic loading due to vibration and maneuvering. Ti-6Al-4V possesses excellent fatigue resistance, ensuring reliability over the operational life of the component.
- 생체 적합성: While not relevant for missile fins, its biocompatibility makes it a standard in medical implants, highlighting its inertness.
- Weldability & Fabricability: It can be processed using various methods, including AM (SLM, EBM, DED).
- AM Considerations for Ti-6Al-4V:
- Successfully printing Ti-6Al-4V requires careful control of the build environment (typically inert gas like Argon) to prevent oxygen pickup, which can embrittle the material.
- As-built parts often have an acicular (needle-like) martensitic microstructure due to rapid cooling. Post-processing heat treatments (like annealing or stress relief, often followed by Hot Isostatic Pressing – HIP) are typically required to achieve the desired microstructure (usually a duplex alpha-beta structure) for optimal ductility, fracture toughness, and fatigue properties, meeting stringent aerospace specifications. HIPing also helps close any residual internal porosity.
- Support structures are necessary during the build process and require careful removal.
- Procurement: 고품질 소싱 wholesale titanium powder specifically characterized for AM processes (particle size distribution, morphology, flowability, chemistry) is critical. Working with established suppliers like Met3dp, who manufacture powders using advanced atomization technologies, ensures consistency and traceability crucial for aerospace applications.
Table: Typical Properties of AM Ti-6Al-4V (Post-Processed)
속성 | Typical Value (Metric) | Typical Value (Imperial) | Significance for Missile Fins |
---|---|---|---|
밀도 | ~4.43g/cm³ | ~0.160 lb/in³ | Low density contributes to lightweighting. |
궁극의 인장 강도 | 950 – 1100 MPa | 138 – 160 ksi | High strength to withstand aerodynamic and maneuver loads. |
항복 강도(0.2%) | 850 - 1000 MPa | 123 – 145 ksi | Indicates resistance to permanent deformation. |
휴식 시 신장 | 10 – 18% | 10 – 18% | Ductility, ability to deform before fracture (post-HIP/HT). |
탄성 계수 | ~114 GPa | ~16.5 Msi | Stiffness, resistance to bending/flexing under load. |
최대 서비스 온도 | ~315 – 400°C (application dep.) | ~600 – 750°F (application dep.) | Ability to retain strength at elevated temperatures. |
피로 강도 | 높음 | 높음 | Resistance to failure under cyclic loading (vibration, maneuvers). |
시트로 내보내기
2. Scalmalloy®: High-Performance Aluminum Alloy
Scalmalloy® is a patented high-performance aluminum-magnesium-scandium (Al-Mg-Sc) alloy specifically developed for additive manufacturing by APWORKS, a subsidiary of Airbus. It offers properties that bridge the gap between traditional high-strength aluminum alloys and titanium, making it an attractive option for applications demanding lightweighting and high strength, potentially at a lower cost point than titanium.
- Key Properties & Benefits:
- Very High Specific Strength: Scalmalloy® boasts a specific strength (strength divided by density) significantly higher than traditional aerospace aluminum alloys (like 7075 or 2024) and comparable to, or even exceeding, that of Ti-6Al-4V in some conditions. This makes it extremely attractive for lightweight structural components like fins.
- Excellent Ductility and Toughness: Unlike some very high-strength aluminum alloys which can be brittle, Scalmalloy® maintains good ductility and fracture toughness, even at cryogenic temperatures.
- 우수한 내식성: Offers good general corrosion resistance.
- Excellent Weldability (in AM context): Developed specifically for the SLM process, it exhibits excellent processability and resistance to hot cracking during printing.
- Microstructural Stability: The addition of Scandium helps create fine grain structures that are stable even after heat treatment, contributing to its high strength.
- AM Considerations for Scalmalloy®:
- Typically processed using Selective Laser Melting (SLM).
- Requires a specific heat treatment post-printing to achieve its optimal mechanical properties (solution heat treatment followed by artificial aging).
- Like titanium, support structures are needed and must be removed.
- While strong, its maximum service temperature is generally lower than Ti-6Al-4V, making it more suitable for applications without extreme aerodynamic heating (e.g., subsonic or low-supersonic missiles, UAV control surfaces).
- Procurement: As a specialized, patented alloy, Scalmalloy® powder is available from licensed suppliers. Organizations looking for high-performance aluminum options should inquire about its availability and processing capabilities from their chosen AM service provider or explore options with aluminum alloy distributors familiar with advanced AM materials.
Table: Typical Properties of AM Scalmalloy® (Post-Processed)
속성 | Typical Value (Metric) | Typical Value (Imperial) | Significance for Missile Fins |
---|---|---|---|
밀도 | ~2.67 g/cm³ | ~0.096 lb/in³ | Extremely low density, excellent for aggressive lightweighting. |
궁극의 인장 강도 | ~520 MPa | ~75 ksi | Very high strength for an aluminum alloy. |
항복 강도(0.2%) | ~480 MPa | ~70 ksi | High resistance to permanent deformation. |
휴식 시 신장 | ~13% | ~13% | Good ductility, preventing brittle fracture. |
탄성 계수 | ~70 GPa | ~10 Msi | Typical aluminum stiffness. |
최대 서비스 온도 | ~150-200°C (guide) | ~300-390°F (guide) | Lower than Ti-6Al-4V, suitable for less demanding thermal environments. |
피로 강도 | 우수 | 우수 | Superior fatigue performance compared to traditional Al alloys. |
시트로 내보내기
Choosing Between Ti-6Al-4V and Scalmalloy®:
The decision between these two high-performance materials often depends on the specific requirements of the missile fin application:
- Choose Ti-6Al-4V when:
- Higher operating temperatures are expected (supersonic flight).
- Maximum absolute strength and stiffness are prioritized over absolute minimum weight.
- Proven track record in demanding aerospace applications is essential.
- Choose Scalmalloy® when:
- Aggressive lightweighting is the primary goal.
- Operating temperatures are moderate (subsonic, low supersonic).
- A balance of high strength, excellent ductility, and fatigue life is needed at a lower density than titanium.
- Cost optimization compared to titanium is a factor (material cost is generally lower, though processing complexities exist).
Both Ti-6Al-4V and Scalmalloy® represent significant advancements in materials technology, particularly when combined with the geometric freedom of metal additive manufacturing. By carefully selecting the appropriate material and partnering with experienced manufacturers like Met3dp, who possess deep knowledge in both powder production and 고급 인쇄 방법, engineers can design and produce missile fins that meet the increasingly stringent performance demands of modern aerospace and defense systems. The availability of high-quality, certified 항공우주 등급 소재 in powder form is the foundation upon which the success of AM in these critical applications is built.
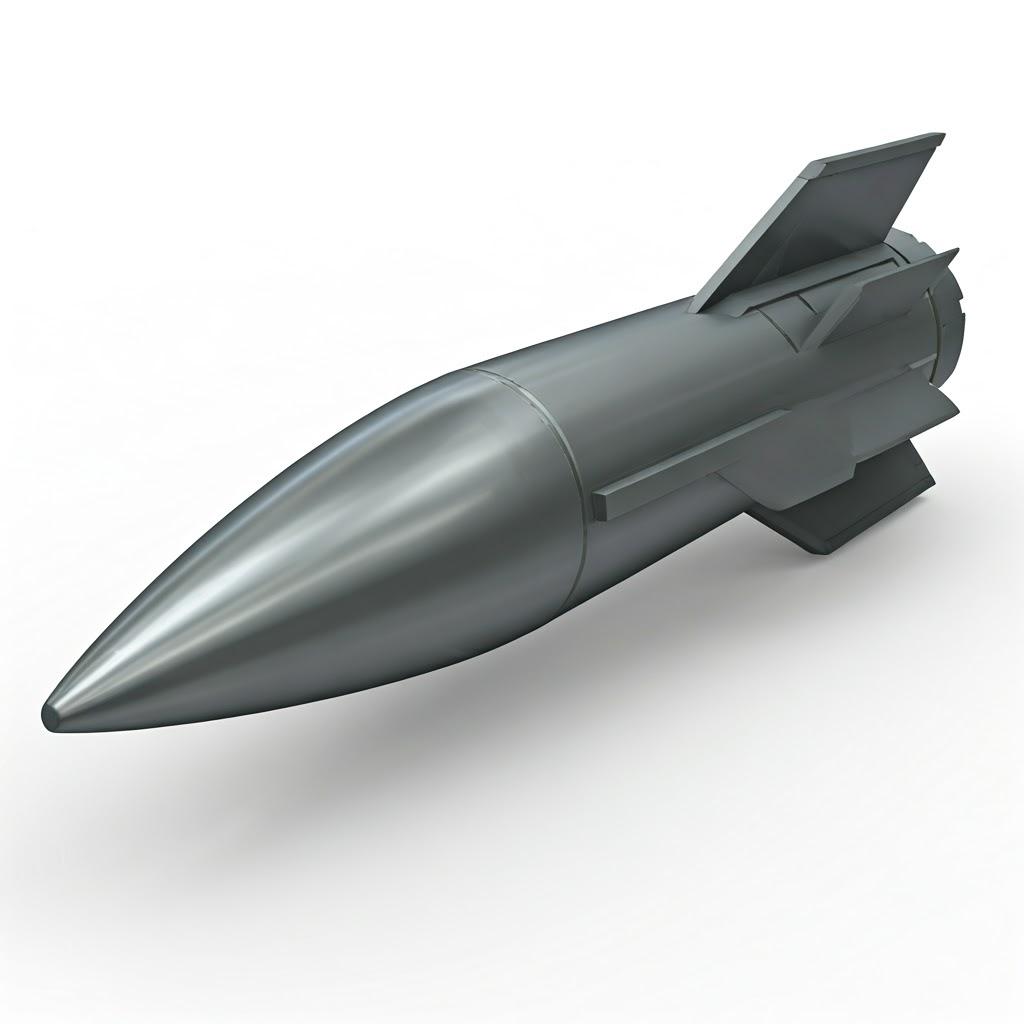
Optimizing Missile Fin Design for Additive Manufacturing (DfAM)
Simply taking a design originally intended for CNC machining or casting and sending it to a metal 3D printer rarely unlocks the full potential of additive manufacturing. To truly harness the benefits of AM – particularly lightweighting, performance enhancement, and cost-effectiveness for complex components like missile fins – engineers must embrace Design for Additive Manufacturing (DfAM). DfAM is not just a software tool; it’s a methodology, a shift in thinking that considers the unique capabilities and constraints of layer-by-layer fabrication right from the conceptual design stage. Failing to apply DfAM principles can lead to suboptimal results, including unnecessarily heavy parts, longer print times, increased support structure requirements (adding cost and post-processing effort), and potential build failures. For critical aerospace components like missile fins, optimizing the design for the chosen AM process and material is paramount.
Key DfAM Principles for 3D Printed Missile Fins:
- 토폴로지 최적화:
- 개념: This is perhaps the most powerful DfAM tool for aerospace components. Topology optimization software uses Finite Element Analysis (FEA) algorithms to determine the most efficient material distribution within a defined design space, subject to specified loads, constraints, and performance targets (e.g., stiffness).
- Application for Fins: Starting with a basic fin envelope and defining the load cases (aerodynamic pressure, maneuver loads, vibration), the software iteratively removes material from low-stress areas, leaving behind an often organic-looking, highly efficient load-bearing structure. This can result in significant weight savings (often 20-50% or more compared to traditional designs) while meeting or exceeding structural requirements. The resulting complex geometries are often ideally suited for AM production.
- 고려 사항: Optimized designs must still be manufacturable. Minimum wall thicknesses, overhang angles, and feature resolution need to be considered. Often, some smoothing or geometry reconstruction is needed after optimization.
- Lattice Structures and Infill:
- 개념: Instead of solid material, internal volumes can be filled with engineered lattice structures (e.g., gyroids, honeycombs, truss-like structures). These structures offer high strength and stiffness relative to their density.
- Application for Fins: For thicker fin sections or internal ribbing, solid material can be replaced with lightweight lattices. This reduces mass and material consumption while maintaining structural integrity. Different lattice types can be chosen based on whether load requirements are directional or multi-axial. This technique can be combined with topology optimization for further weight reduction.
- 고려 사항: Powder removal from complex internal lattices can be challenging and must be planned for (e.g., incorporating drainage holes). Lattice density and cell size impact both mechanical properties and printability.
- Support Structure Strategy and Orientation:
- 개념: Most powder bed fusion AM processes require support structures for overhanging features (typically angles below 45 degrees from the horizontal) and to anchor the part to the build plate, managing thermal stresses. Supports consume material, add print time, require removal in post-processing (which can affect surface finish), and can be difficult to remove from internal channels.
- Application for Fins: The orientation in which the fin is printed significantly impacts support needs. Designers should:
- Orient for Self-Support: If possible, orient the fin to minimize steep overhangs. Often, printing fins vertically or at a slight angle can reduce reliance on supports for the main airfoil surfaces.
- Design for Support Removal: Ensure accessibility for removing supports, especially if complex internal features exist. Avoid designs where supports are trapped internally.
- Consider Sacrificial Features: Sometimes, adding small features specifically designed to be machined away later can provide necessary support during printing without compromising the final geometry.
- Expert Input: Collaborating with an experienced AM service provider like Met3dp during the design phase can provide valuable insights into optimal orientation and support strategies, leveraging their deep understanding of process limitations and best practices.
- Minimum Feature Size and Wall Thickness:
- 개념: Every AM machine and material combination has limitations on the smallest features (e.g., pins, holes) and thinnest walls it can reliably produce with the required accuracy.
- Application for Fins: Thin trailing edges, sharp leading edges, or small internal channels need to be designed with the specific process capabilities in mind. For instance, Laser Powder Bed Fusion (L-PBF or SLM) can typically achieve finer features than Electron Beam Melting (EBM). Aiming for wall thicknesses below the process capability (e.g., <0.4-0.5 mm for some L-PBF systems) can lead to incomplete formation or poor dimensional accuracy.
- Met3dp’s Capabilities: Utilizing industry-leading equipment, Met3dp can achieve high accuracy and resolution, but designers should still adhere to practical minimums for robustness and manufacturability. Consulting their design guidelines is recommended.
- Thermal Management Considerations:
- 개념: The rapid heating and cooling inherent in metal AM can induce residual stresses and potential distortion (warping). Design choices can influence the thermal behavior during the build.
- Application for Fins:
- Avoid Large, Solid Sections: These can accumulate significant thermal stress. Incorporating lattices or internal voids can help.
- Smooth Transitions: Sharp changes in cross-section can create stress concentrations. Using fillets and smooth transitions is beneficial.
- Symmetric Design (where possible): Symmetric parts tend to distribute thermal stresses more evenly.
- Simulation: Thermal simulation tools can predict potential stress hotspots and distortion, allowing designers to modify the geometry or build strategy proactively.
- 파트 통합:
- 개념: AM allows complex assemblies to be redesigned and printed as a single, integrated component.
- Application for Fins: While a single fin is often one piece, consider its attachment points or associated mechanisms. Could brackets, small fairings, or parts of an actuation linkage be integrated directly into the fin’s root section? This reduces part count, eliminates fasteners or joints (potential failure points), simplifies assembly, and can further reduce weight.
- 트레이드 오프: Consider inspectability and repairability. A consolidated part might be harder to inspect internally or impossible to repair if one section is damaged.
- Designing for Post-Processing:
- 개념: AM parts, especially for aerospace, almost always require post-processing (heat treatment, support removal, machining, surface finishing). Design choices should facilitate these steps.
- Application for Fins:
- Machining Allowances: If critical surfaces (e.g., mounting interfaces, hinge lines) require tight tolerances or specific finishes achievable only by machining, add extra material (machining stock) to those areas in the AM design.
- Access for Support Removal: As mentioned, ensure supports are accessible.
- Fixturing Points: Consider adding temporary features that can aid in holding the part securely during post-machining or inspection, which can be removed later.
Leveraging DfAM Expertise:
Successfully implementing DfAM requires a combination of software tools, engineering knowledge, and practical manufacturing experience. Companies venturing into AM for critical components like missile fins often benefit from partnering with specialized service providers. Met3dp, for example, offers more than just printing; their team possesses expertise in additive manufacturing solutions, including DfAM consultation. They can assist clients in evaluating existing designs for AM suitability, identifying opportunities for optimization (lightweighting, consolidation), and ensuring the final design is truly manufacturable, leveraging the full potential of their advanced metal 3D printing equipment and processes. By incorporating DfAM principles, manufacturers can produce missile fins that are lighter, stronger, potentially more aerodynamically efficient, and delivered faster than previously possible.
Achieving Precision: Tolerance, Surface Finish, and Dimensional Accuracy in 3D Printed Fins
For aerodynamic components like missile fins, precision is non-negotiable. The fin’s shape directly influences airflow, stability, and control effectiveness. Mounting points must align perfectly with the missile body or actuation mechanisms. Therefore, understanding and achieving the required dimensional accuracy, tolerances, and surface finish are critical aspects of using metal additive manufacturing for these applications. While AM offers incredible geometric freedom, it inherently differs from the precision standards often associated with multi-axis CNC machining directly from the outset. However, through careful process control, appropriate material selection, and planned post-processing, AM can meet the stringent requirements of aerospace components.
Dimensional Accuracy and Tolerances:
- 정의: Dimensional accuracy refers to how closely the printed part conforms to the nominal dimensions specified in the CAD model. Tolerance defines the permissible range of variation for a given dimension.
- Typical AM Capabilities: The achievable accuracy in metal AM depends heavily on several factors:
- AM 프로세스: Laser Powder Bed Fusion (L-PBF/SLM) generally offers higher accuracy and finer feature resolution than Electron Beam Melting (EBM), which tends to build faster but with slightly looser tolerances and rougher surfaces. Directed Energy Deposition (DED) typically has lower accuracy than PBF methods.
- 머신 보정: Well-maintained and precisely calibrated machines are essential. Thermal drift, laser/beam alignment, and powder layer consistency all impact accuracy.
- Part Size and Geometry: Larger parts generally exhibit greater absolute deviation due to accumulated thermal effects and potential distortion. Complex geometries with internal features or thin walls can be more challenging to control dimensionally.
- Material: Different materials have varying shrinkage rates and thermal conductivity, affecting final dimensions.
- Build Orientation and Supports: How the part is oriented and supported influences thermal behavior and potential distortion, impacting accuracy.
- 후처리: Heat treatments (stress relief, HIP) can cause slight dimensional changes that must be accounted for. Finish machining is often used to achieve the tightest tolerances on critical features.
- General Tolerance Ranges (Powder Bed Fusion):
- As-Built: Typical tolerances for well-controlled L-PBF processes might be in the range of ±0.1 mm to ±0.2 mm (±0.004″ to ±0.008″) for smaller dimensions, potentially increasing to ±0.5 mm (±0.020″) or more for larger dimensions or less controlled processes. EBM tolerances are generally looser.
- With Post-Machining: For critical interfaces, mounting holes, or aerodynamic surfaces requiring very tight tolerances (e.g., ±0.025 mm / ±0.001″ or better), post-print CNC machining is typically employed. DfAM principles should incorporate machining stock in these areas.
표면 마감(거칠기):
- 정의: Surface finish, often quantified by the arithmetic average roughness (Ra), describes the texture of the part’s surface. Smooth surfaces are generally preferred for external aerodynamic applications to minimize drag, while specific roughness might be desired for bonding or coating adhesion.
- Typical AM Surface Roughness:
- As-Built: Metal AM parts inherently have a rougher surface finish than machined parts. This is due to the partially melted powder particles adhering to the surface and the layer-by-layer build process creating slight stepping effects, especially on curved or angled surfaces.
- L-PBF: Typical Ra values range from 6 µm to 20 µm (240 µin to 790 µin), depending on material, parameters, and surface orientation (upward-facing surfaces tend to be smoother than downward-facing or vertical walls).
- EBM: Generally produces rougher surfaces than L-PBF, often in the Ra 20 µm to 35 µm (790 µin to 1380 µin) range.
- Impact of Orientation: Surfaces parallel to the build plate tend to be rougher than vertical walls. Angled surfaces show layer stepping effects.
- As-Built: Metal AM parts inherently have a rougher surface finish than machined parts. This is due to the partially melted powder particles adhering to the surface and the layer-by-layer build process creating slight stepping effects, especially on curved or angled surfaces.
- 표면 마감 개선:
- 프로세스 매개변수 최적화: Fine-tuning laser/beam parameters can slightly improve finish, but significant improvements require secondary operations.
- 연마제 블라스팅(비드/모래 블라스팅): Provides a more uniform, matte finish, often reducing Ra slightly but not achieving a polished look.
- 텀블링/진동 마감: Can smooth surfaces and deburr edges, particularly for smaller parts.
- Chemical Polishing/Etching: Can achieve very smooth surfaces but requires careful control and material compatibility.
- CNC 가공: The most reliable way to achieve very smooth finishes (Ra < 1.6 µm / 63 µin or better) on specific surfaces.
- 수동 연마: Labor-intensive but capable of achieving mirror finishes if required.
Table: Surface Finish Comparison (Typical Ra Values)
제조 프로세스 | Typical Ra (µm) | Typical Ra (µin) | 참고 |
---|---|---|---|
Metal L-PBF (As-Built) | 6 – 20 | 240 – 790 | Varies with orientation, material, parameters |
Metal EBM (As-Built) | 20 – 35 | 790 – 1380 | Generally rougher than L-PBF |
Abrasive Blasting (Post-AM) | 5 – 15 | 200 – 590 | Creates uniform matte finish |
Standard CNC Machining | 1.6 – 6.3 | 63 – 250 | Good general-purpose finish |
정밀 CNC 가공 | 0.4 – 1.6 | 16 – 63 | Tighter tolerances, smoother surfaces |
Grinding / Polishing | < 0.4 | < 16 | Very smooth, often mirror-like finishes |
시트로 내보내기
Quality Control and Metrology:
Achieving and verifying the required precision for aerospace components like missile fins demands rigorous quality control and metrology procedures.
- 진행 중인 모니터링: Advanced AM systems incorporate sensors to monitor aspects like melt pool temperature, layer uniformity, and atmospheric conditions, providing real-time feedback for quality assurance.
- 비파괴 검사(NDT):
- Computed Tomography (CT Scanning): Essential for inspecting internal features, detecting voids or porosity, and verifying complex internal geometries (like lattices or cooling channels) without destroying the part.
- Fluorescent Penetrant Inspection (FPI): Used to detect surface-breaking cracks.
- Ultrasonic Testing (UT): Can detect subsurface flaws.
- Dimensional Metrology:
- Coordinate Measuring Machines (CMMs): Provide high-accuracy point measurements to verify critical dimensions and tolerances against the CAD model.
- 3D Laser Scanning / Structured Light Scanning: Capture dense point clouds of the entire part surface, allowing comparison to the CAD model (geometric dimensioning and tolerancing – GD&T analysis) and verification of complex curvatures and overall form.
- Material Property Testing: Destructive testing of sample coupons printed alongside the main parts is often required to verify tensile strength, ductility, hardness, and microstructure, ensuring they meet material specifications.
Met3dp’s Commitment to Precision:
Achieving aerospace-level precision requires not only advanced equipment but also robust processes and expertise. Met3dp utilizes industry-leading printing systems known for their accuracy and reliability. Their comprehensive approach includes stringent process controls, meticulous machine calibration, and thorough quality inspection protocols, incorporating various NDT and metrology techniques. This commitment ensures that components like missile fins manufactured by Met3dp meet the demanding dimensional accuracy, tolerance, and surface finish requirements critical for mission success in the aerospace and defense sectors. Partnering with a provider that prioritizes and demonstrates this level of 정밀 제조 capability is essential for critical hardware.
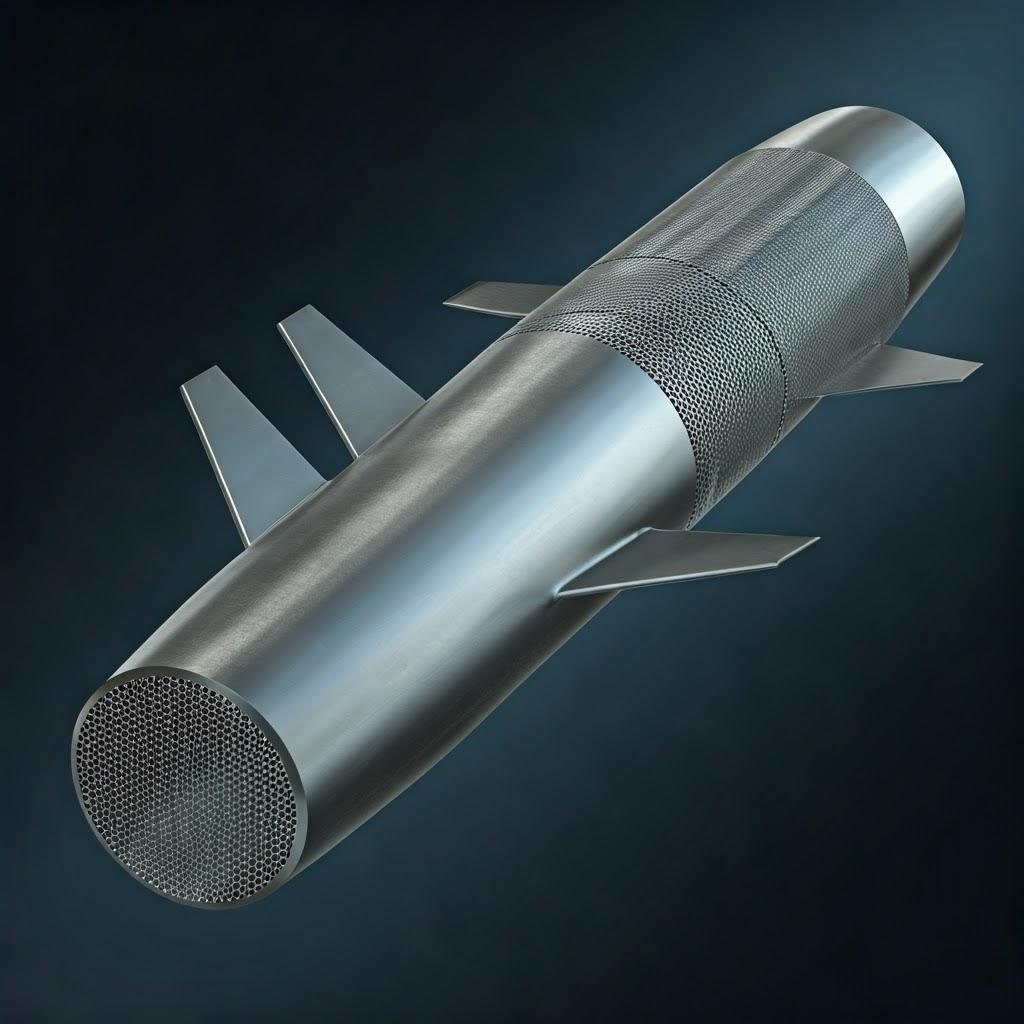
Essential Post-Processing Steps for 3D Printed Missile Fins
A common misconception about metal additive manufacturing is that parts come out of the printer ready to use. In reality, especially for high-performance applications like missile fins, the printing process is just one step in the overall manufacturing workflow. Post-processing encompasses a series of essential treatments and finishing operations required to transform the as-built part into a functional, reliable component meeting all engineering specifications. These steps are crucial for relieving internal stresses, removing temporary structures, achieving desired material properties, ensuring dimensional accuracy, and obtaining the required surface finish. Neglecting or improperly executing post-processing can compromise the part’s performance and integrity.
Common Post-Processing Workflow for AM Missile Fins:
- 스트레스 해소:
- 목적: The rapid heating and cooling cycles during the layer-by-layer fusion process induce significant residual stresses within the metal part. These stresses can cause distortion (warping) upon removal from the build plate or during subsequent machining, and can negatively impact fatigue life and dimensional stability.
- 방법: A thermal treatment (heat treatment) performed 전에 removing the part from the build plate. The part, still attached to the plate, is heated to a specific temperature (below the material’s critical transformation point, e.g., 590-700°C for Ti-6Al-4V depending on the desired outcome), held for a period, and then slowly cooled. This allows the internal stresses to relax without causing significant distortion.
- Importance: This is often a mandatory first step for high-precision metal AM parts to ensure dimensional stability during subsequent operations.
- 빌드 플레이트에서 파트 제거:
- 목적: To separate the printed fin(s) and their support structures from the base plate they were built upon.
- 방법:
- 와이어 EDM(방전 가공): A precise method often used for titanium and other tough alloys. It provides a clean cut with minimal mechanical stress.
- 밴드 톱질: A faster but less precise method, suitable if the base requires subsequent machining anyway.
- 가공: Milling the part off the plate.
- 지원 구조 제거:
- 목적: To remove the temporary supports required during the build process for overhangs and thermal management.
- 방법:
- 수동 제거: Supports are often designed with weakened interfaces and can sometimes be broken off by hand or with simple tools (pliers, cutters). This is common for accessible supports.
- Machining (Milling, Grinding): Used to remove support interface points precisely and smooth the “witness marks” left on the part surface. Essential for aerodynamic surfaces.
- Wire EDM: Can be used to access and cut away supports in difficult-to-reach areas.
- Abrasive Blasting: Can sometimes help weaken or remove finer support structures.
- 도전 과제: Support removal can be labor-intensive and time-consuming, especially for complex geometries or internal supports. Damage to the part surface can occur if not done carefully. DfAM plays a crucial role in minimizing support needs and ensuring accessibility.
- Heat Treatment (Solution Annealing, Aging, HIP):
- 목적: To homogenize the microstructure, refine grain structure, improve ductility and fracture toughness, enhance fatigue life, and achieve the final desired mechanical properties as per material specifications (e.g., AMS standards for aerospace). As-built AM microstructures are often non-ideal due to rapid solidification.
- 방법:
- Annealing/Solution Treatment: Heating to a higher temperature (e.g., above the beta transus for Ti-6Al-4V, or ~500°C+ for Scalmalloy) followed by controlled cooling (quenching or air cooling).
- 노화: A lower temperature heat treatment following solution treatment (particularly for alloys like Scalmalloy® or precipitation-hardening steels) to precipitate strengthening phases.
- 열간 등방성 프레싱(HIP): This is a critical step for many aerospace AM components, especially titanium. The part is subjected to high temperature (just below the melting point) and high isostatic pressure (using an inert gas like Argon) simultaneously in a specialized vessel. HIP effectively closes any residual internal porosity (micro-voids) that might remain after printing, significantly improving fatigue properties, ductility, and overall structural integrity. It also acts as a stress relief and annealing treatment.
- Specification Driven: The exact heat treatment cycles (temperatures, times, cooling rates, atmosphere) are dictated by the material specification and the desired final properties.
- Finish Machining:
- 목적: To achieve tight tolerances on critical dimensions, improve surface finish on specific features, and create precise mating surfaces or interfaces.
- Areas Typically Machined:
- Mounting interfaces (e.g., bolt holes, mating flanges at the fin root).
- Aerodynamically critical surfaces (leading/trailing edges, specific airfoil sections if required finish is very high).
- Hinge lines or actuator attachment points.
- 방법: Typically 3-axis or 5-axis CNC milling, turning, or grinding. Requires careful fixturing of the AM part. DfAM should account for material stock addition in areas designated for machining.
- 표면 마감:
- 목적: To achieve the final desired surface texture for aerodynamic performance, paint/coating adhesion, or aesthetic requirements.
- Methods (beyond machining):
- Abrasive Blasting (Bead, Sand, Grit): Creates a uniform matte finish, removes discoloration from heat treatment, and can provide a good surface profile for coating adhesion. Different media provide different levels of roughness.
- 텀블링/진동 마감: Uses abrasive media in a rotating or vibrating drum to smooth surfaces, deburr edges, and provide a consistent finish, especially effective for batches of smaller parts.
- Electropolishing/Chemical Milling: Can produce very smooth, bright finishes on certain alloys by electrochemically removing material.
- Manual Polishing/Lapping: For achieving very high finishes (e.g., mirror polish) on specific areas, though labor-intensive.
- Coating Application (Optional):
- 목적: To provide enhanced properties like thermal protection (thermal barrier coatings for high-speed applications), corrosion resistance, wear resistance, or specific electromagnetic (low observable) characteristics.
- 방법: Painting, powder coating, anodizing (for aluminum/titanium), plasma spray coatings, PVD/CVD coatings, etc., depending on the requirement. Surface preparation (cleaning, blasting) is critical for coating adhesion.
- Final Inspection and Cleaning:
- 목적: To verify that all post-processing steps have been completed correctly, the part meets all dimensional and surface finish specifications, and it is clean and free from contaminants before assembly or delivery.
- 방법: Final dimensional inspection (CMM, scanning), visual inspection, NDT if required post-machining/heat treatment, and cleaning according to specifications.
Integrated Approach:
Effective post-processing requires careful planning and integration into the overall manufacturing strategy. It is not an afterthought. The choice of AM process, material, and DfAM decisions directly impacts the type and extent of post-processing required. Partnering with a full-service provider like Met3dp, who understands the intricacies of both the printing process and the necessary downstream parts finishing services, ensures a streamlined workflow and a final component that meets the rigorous demands of aerospace applications. Their expertise covers the entire chain, from powder to finished part, guaranteeing quality and performance. Procurement managers should ensure potential suppliers have validated post-processing capabilities and quality controls in place for the specific materials and requirements involved.
Overcoming Common Challenges in Metal AM for Missile Fins
While metal additive manufacturing offers transformative potential for producing complex components like missile fins, it is not without its challenges. Successfully implementing AM for critical aerospace hardware requires a deep understanding of the potential pitfalls and the strategies to mitigate them. These challenges often relate to the fundamental physics of rapidly melting and solidifying metal powder in a layer-wise fashion, as well as the complexities of managing the overall process chain. Addressing these proactively is key to achieving repeatable, high-quality results that meet stringent aerospace standards.
Key Challenges and Mitigation Strategies:
- Residual Stress and Distortion:
- 도전: The intense, localized heating from the laser or electron beam, followed by rapid cooling, creates steep thermal gradients within the part during the build. This leads to the buildup of internal residual stresses. If these stresses exceed the material’s yield strength at elevated temperature, or upon cooling and removal from the build plate, they can cause the part to distort, warp, or even crack. Missile fins, often having thin sections and asymmetric geometries, can be susceptible.
- 완화 전략:
- Optimized Build Orientation: Printing the fin in an orientation that minimizes large, flat surfaces parallel to the build plate and reduces overhangs can help manage thermal gradients.
- Intelligent Support Structures: Supports do more than just hold up overhangs; they act as heat sinks and anchor the part firmly to the rigid build plate, counteracting shrinkage forces. Support design (density, placement, type) is critical for stress management. Experienced providers use simulation tools to optimize support strategies.
- 프로세스 매개변수 최적화: Adjusting parameters like laser power, scan speed, layer thickness, and scan strategy (e.g., using an island scanning pattern) can influence the thermal history and reduce stress accumulation.
- Platform Heating (EBM & some L-PBF): Maintaining the build platform and surrounding powder at an elevated temperature (e.g., several hundred degrees Celsius) reduces the thermal gradients during processing, significantly lowering residual stress. This is a key advantage of EBM and some advanced L-PBF systems.
- 스트레스 완화 열처리: Performing an appropriate thermal stress relief cycle 전에 removing the part from the build plate is the most common and effective way to relax accumulated stresses.
- 다공성:
- 도전: Small voids or pores can become trapped within the solidified material. Porosity acts as a stress concentrator and can significantly degrade mechanical properties, particularly fatigue life, which is critical for missile fins subjected to vibration and maneuvering loads. Porosity can arise from:
- 가스 다공성: Gases (like Argon from the build chamber or dissolved gases in the powder) trapped within the melt pool during solidification.
- 융합 부족 다공성: Insufficient energy input or improper beam overlap leads to incomplete melting between powder particles or successive layers, leaving voids.
- 키홀 다공성: Excessive energy density can cause deep, unstable melt pools (keyholing) that collapse and trap vapor/gas.
- 완화 전략:
- High-Quality Powder: Using metal powders with low internal porosity, controlled particle size distribution, good flowability, and low absorbed gas content is fundamental. Met3dp’s advanced powder manufacturing techniques (Gas Atomization, PREP) are designed to produce such high-quality powders.
- Optimized Process Parameters: Developing robust parameter sets (laser/beam power, speed, focus, layer thickness, hatch spacing) specific to the material and machine is crucial to ensure complete melting and stable melt pool behavior. This often requires extensive development and qualification.
- Proper Machine Setup and Maintenance: Ensuring correct gas flow dynamics in the build chamber, proper beam calibration, and clean optics/electron gun components.
- 열간 등방성 프레싱(HIP): As mentioned in post-processing, HIP is highly effective at closing internal pores (except those open to the surface) through high temperature and pressure, significantly improving material density and mechanical properties. It is standard practice for critical Ti-6Al-4V AM components.
- 도전: Small voids or pores can become trapped within the solidified material. Porosity acts as a stress concentrator and can significantly degrade mechanical properties, particularly fatigue life, which is critical for missile fins subjected to vibration and maneuvering loads. Porosity can arise from:
- Powder Removal from Internal Features:
- 도전: If the fin design incorporates complex internal channels (e.g., for lightweighting lattices, potential cooling passages), removing all the unfused powder after the build can be extremely difficult. Trapped powder adds weight and can be detrimental if it comes loose during operation.
- 완화 전략:
- DfAM for Powder Removal: Designing internal channels with sufficient diameter, smooth pathways, and strategically placed drain/access holes specifically for powder evacuation. Avoid complex, dead-end cavities.
- 빌드 오리엔테이션: Orienting the part to facilitate powder drainage via gravity during or after the build.
- 포스트 프로세싱 기법: Using compressed air, vibration tables, ultrasonic cleaning baths, and sometimes specialized flushing rigs to dislodge and remove trapped powder.
- CT Scanning: Used for verification to ensure complete powder removal from critical internal passages.
- Surface Finish and Feature Resolution:
- 도전: As-built AM surfaces are inherently rougher than machined surfaces, and achieving very fine details or sharp edges can be limited by the process physics (melt pool size, powder particle size). This can impact aerodynamic performance or fitment.
- 완화 전략:
- Process Selection: L-PBF generally offers better surface finish and feature resolution than EBM.
- 매개변수 최적화: Fine-tuning parameters can marginally improve finish.
- DfAM: Avoiding features below the reliable resolution limit of the chosen process. Designing slightly thicker trailing edges that can be machined sharp if needed.
- Targeted Post-Processing: Employing machining, polishing, or other surface finishing techniques specifically on critical aerodynamic surfaces or interfaces where smoothness and precision are required.
- Quality Assurance and Repeatability:
- 도전: Ensuring that every printed fin meets the exact same stringent quality standards (dimensional accuracy, material properties, defect levels) consistently across different builds and machines is a major requirement for aerospace. The process has many variables that need tight control.
- 완화 전략:
- Robust Quality Management System (QMS): Implementing aerospace-certified QMS (like AS9100) covering all aspects from powder procurement and handling to process control, post-processing, inspection, and traceability. Met3dp operates under such rigorous quality systems.
- 프로세스 모니터링: Utilizing in-situ monitoring tools (melt pool monitoring, thermal imaging, layer imaging) to detect potential anomalies during the build.
- Standardized Procedures: Having clearly defined and validated procedures for machine setup, calibration, operation, post-processing, and inspection.
- Material Property Verification: Consistent testing of witness coupons printed with each build to verify mechanical properties.
- Comprehensive NDT and Metrology: Rigorous inspection of final parts using CT scanning, CMM, 3D scanning, etc.
- Process Validation & Qualification: Thoroughly validating the entire manufacturing process (including specific parts, materials, machines, and post-processing steps) according to aerospace requirements.
Overcoming these challenges requires significant investment in technology, process development, quality systems, and expertise. This is why partnering with an established metal AM provider like Met3dp, with a proven track record in demanding industries and a deep understanding of materials science, process physics, and aerospace quality standards, is often the most effective approach for organizations seeking to leverage AM for critical components like missile fins. Their focus on providing comprehensive 첨가 제조 솔루션 helps mitigate risks and ensures the delivery of high-performance, reliable parts.
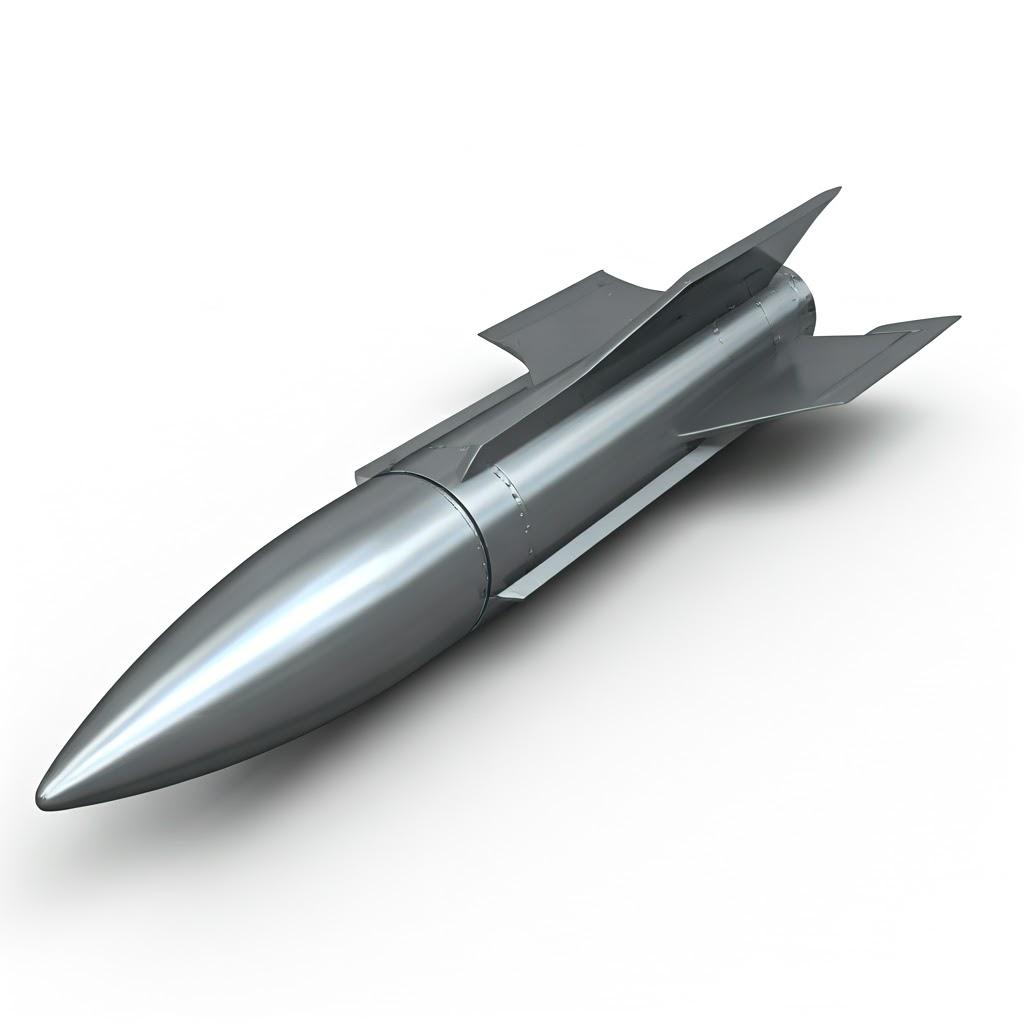
Selecting Your Strategic Partner: Choosing a Metal 3D Printing Service Provider
The success of incorporating 3D printed missile fins into your aerospace or defense program hinges significantly on the capabilities, reliability, and expertise of your chosen manufacturing partner. Selecting the right metal additive manufacturing (AM) service provider, often referred to as an AM service bureau or contract manufacturer, goes beyond simply finding a company with a 3D printer. For flight-critical components like missile fins, the selection process demands rigorous evaluation against stringent criteria encompassing technical proficiency, quality assurance, security, and industry-specific certifications. This partner will be integral to your supply chain, impacting quality, lead times, cost, and potentially even design optimization.
Key Criteria for Evaluating Metal AM Service Providers for Missile Fin Production:
- Aerospace & Defense Certifications:
- AS9100: This is the cornerstone quality management system standard for the aviation, space, and defense industries. Certification to AS9100 (or equivalent standards like EN9100) demonstrates a provider’s commitment to rigorous quality processes, traceability, risk management, and continuous improvement tailored to aerospace requirements. It is often a non-negotiable requirement for Tier 1 and Tier 2 suppliers.
- ISO 9001: A fundamental quality management standard, often a prerequisite for AS9100.
- NADCAP (National Aerospace and Defense Contractors Accreditation Program): While AS9100 covers the overall QMS, NADCAP provides specific accreditation for special processes like heat treating, materials testing, welding, and increasingly, additive manufacturing itself. NADCAP accreditation for relevant processes provides an additional layer of assurance.
- ITAR Registration (if applicable): For projects involving U.S. defense articles or technical data covered by the International Traffic in Arms Regulations, the provider must be ITAR registered and have robust procedures in place to handle controlled information and hardware securely. Similar export control regulations exist in other regions (e.g., BAFA in Germany, Export Controls UK).
- Proven Experience and Expertise:
- Material Specialization: Does the provider have documented experience successfully printing the specific high-strength materials required (e.g., Ti-6Al-4V, Scalmalloy®)? Ask for case studies, material datasheets derived from their processes, and evidence of parameter optimization for these alloys.
- Application Experience: Have they previously manufactured similar components in terms of complexity, size, and criticality? Experience with aerospace structures, aerodynamic components, or defense hardware is highly valuable.
- Technical Support & DfAM Capability: Does the provider offer engineering support? Can they assist with Design for Additive Manufacturing (DfAM), helping optimize your fin design for lightweighting, performance, and manufacturability? A partner who collaborates on design is often more valuable than one who simply prints the file provided. Met3dp, for instance, emphasizes providing comprehensive solutions, including application development services. You can learn more about their background and expertise by visiting the 회사 소개 section of their website.
- Problem-Solving Skills: Experienced providers understand the common challenges (discussed in the previous section) and have proven strategies to mitigate them.
- Equipment Capability and Capacity:
- Technology Match: Do they operate the appropriate AM technology (e.g., L-PBF, EBM) best suited for your fin’s material, geometry, and required tolerances/surface finish?
- Machine Fleet: Do they have modern, well-maintained equipment from reputable manufacturers? What is the size and redundancy of their machine fleet? This impacts capacity, lead times, and risk mitigation (backup if one machine is down). Met3dp prides itself on employing industry-leading printing equipment known for volume, accuracy, and reliability.
- 볼륨을 구축합니다: Can their machines accommodate the size of your missile fin?
- Atmospheric Control: Particularly for reactive materials like titanium, ensure they have robust inert gas management systems (e.g., Argon) with oxygen monitoring to prevent material contamination.
- Robust Quality Management System (QMS):
- Traceability: The QMS must ensure full traceability from raw material (powder batch) through printing, post-processing, inspection, and final shipment. Lot tracking and documentation are critical.
- Powder Handling and Management: Stringent procedures for powder storage, handling, sieving/recycling, and testing are essential to maintain powder quality and prevent contamination or degradation over time.
- Process Control & Monitoring: Documented procedures for machine setup, calibration, and parameter control. Use of in-process monitoring tools adds value.
- 검사 기능: Do they have the necessary metrology equipment (CMM, 3D scanners) and NDT capabilities (CT scanning, FPI, UT) in-house or through certified partners? Are inspectors trained and qualified?
- Documentation: Ability to provide comprehensive quality documentation, including material certifications, certificates of conformance, inspection reports, and build logs.
- Comprehensive Post-Processing Capabilities:
- Integrated Services: Does the provider offer the necessary post-processing steps (stress relief, heat treatment/HIP, support removal, machining, surface finishing) either in-house or through a tightly managed network of qualified partners? A single point of contact managing the entire workflow is often more efficient and reliable.
- Expertise in Post-Processing: Understanding the specific requirements for heat treating aerospace alloys like Ti-6Al-4V (achieving specific alpha-beta microstructures) or Scalmalloy® (solutionizing and aging) is crucial. HIPing capabilities are often essential for critical titanium parts.
- Security and Confidentiality:
- Data Security: Procedures for handling sensitive CAD data and technical information, especially for defense projects (NDAs, secure data transfer, access controls).
- Facility Security: Physical security measures appropriate for handling potentially sensitive hardware.
- Customer Service and Communication:
- 응답성: How quickly do they respond to inquiries and provide quotes?
- 프로젝트 관리: Is there a dedicated point of contact for your project? How do they manage communication and project updates?
- 투명성: Willingness to discuss challenges, provide process insights, and collaborate on solutions.
The Strategic Value of Partnership:
Choosing an AM service provider for missile fins should be viewed as selecting a strategic partner, not just a vendor. The right partner contributes expertise, ensures quality, manages complexity, and ultimately helps you leverage the full benefits of additive manufacturing. Companies like Met3dp, with their foundation in both advanced powder manufacturing and state-of-the-art printing systems, coupled with decades of collective expertise in metal AM, position themselves as such partners. They aim to provide comprehensive solutions that span equipment, materials, and application development, enabling organizations to implement AM effectively for their most demanding applications. When evaluating potential suppliers, look beyond the quote and assess their overall capability to deliver aerospace-qualified, mission-ready components reliably.
Understanding Cost Factors and Lead Times for 3D Printed Missile Fins
Additive manufacturing introduces different cost structures and lead time dynamics compared to traditional manufacturing methods like CNC machining or casting. Understanding the key drivers behind the price and delivery schedule for 3D printed missile fins is essential for accurate budgeting, project planning, and evaluating the economic viability of AM for a specific application. Procurement managers and engineers should be aware of these factors when requesting quotes and managing supplier relationships.
Key Cost Drivers for Metal AM Missile Fins:
- Material Type and Consumption:
- 파우더 비용: High-performance aerospace-grade metal powders like Ti-6Al-4V and Scalmalloy® are significantly more expensive than standard steels or aluminum alloys used in traditional manufacturing. Scalmalloy® might offer some cost advantage over Titanium, but both are premium materials. The cost is typically calculated per kilogram or pound.
- Part Volume: The actual volume of material making up the final fin directly impacts cost.
- 지원 구조 볼륨: Material used for support structures also adds to the cost. Efficient DfAM aims to minimize this.
- Buy-to-Fly Ratio: While generally better than machining complex parts, AM still has material consumption beyond the final part (supports, unfused powder in the cake). The efficiency depends on nesting density on the build plate and powder recyclability.
- Machine Build Time:
- Primary Driver: This is often the single largest cost component. It’s determined by the total time the AM machine is occupied printing the part(s).
- Influencing Factors:
- 파트 볼륨 및 높이: Larger and taller parts take longer to print layer by layer.
- Number of Parts per Build (Nesting): Printing multiple fins simultaneously on a single build plate utilizes the machine more efficiently, reducing the cost per part (amortizing setup and non-printing time). Effective nesting is key for wholesale component pricing.
- 레이어 두께: Thinner layers provide better resolution and surface finish but significantly increase build time.
- Scan Speed & Strategy: Optimized parameters balance speed with quality.
- 복잡성: Highly complex geometries might require slower scanning speeds or intricate support structures, increasing time.
- 인건비:
- 사전 처리: CAD file preparation, build setup, support structure generation, slicing, and machine programming.
- Machine Operation: Monitoring the build process (though often highly automated).
- 후처리: This can be highly labor-intensive and includes:
- Depowdering / Powder Removal
- Part Removal from Build Plate
- Support Structure Removal (often manual or semi-automated)
- Basic Finishing (e.g., blasting)
- Inspection Labor
- Post-Processing Operations:
- Specialized Treatments: Costs associated with specific required steps like:
- Heat Treatment (Stress Relief, Annealing, Aging): Furnace time, energy consumption, controlled atmospheres.
- 열간 등방성 프레싱(HIP): A specialized and relatively expensive process due to the high pressures and temperatures involved, but often mandatory for fatigue-critical aerospace parts.
- CNC 가공: Machine time, tooling, programming, and skilled machinist labor for achieving tight tolerances and specific surface finishes.
- Advanced Surface Finishing: Costs for polishing, coating, etc.
- Specialized Treatments: Costs associated with specific required steps like:
- Quality Assurance and Inspection:
- 테스트: Costs for material testing (tensile coupons), NDT (CT scanning is particularly significant if required for internal inspection), FPI, etc.
- Metrology: Time and resources for dimensional inspection using CMMs, 3D scanners, etc.
- Documentation: Labor involved in preparing comprehensive quality documentation packages.
- Development & Engineering (NRE):
- Non-Recurring Engineering: For new designs, costs associated with DfAM consultation, process development, parameter optimization, and initial prototyping might be incurred.
- 주문 수량:
- 규모의 경제: As with most manufacturing, higher quantities generally lead to lower unit costs due to amortization of setup costs, optimized machine utilization (full build plates), and potentially more efficient post-processing workflows.
Typical Lead Times for Metal AM Missile Fins:
Lead time refers to the total time from order placement (or file submission) to delivery of the finished parts. It can vary significantly based on complexity, quantity, supplier workload, and the extent of post-processing required.
- Phase 1: Pre-Processing & Setup (1-5 days): Includes quote finalization, design review/DfAM checks, build file preparation (orientation, supports, slicing), and scheduling the job on a machine.
- Phase 2: Printing (1-10+ days): The actual machine time. Can range from less than a day for a single small fin prototype to over a week for a large build plate packed with multiple complex fins. Highly dependent on part height and volume.
- Phase 3: Post-Processing (3 days – 3+ weeks): This phase often has the most variability.
- Basic steps (stress relief, part removal, support removal, blasting): Typically 2-5 days.
- Heat Treatment / HIP: Can add several days to over a week, depending on cycle times and scheduling with specialized facilities if outsourced.
- CNC Machining: Highly variable depending on complexity, can add days to weeks.
- Surface Finishing/Coating: Adds further time based on the process.
- Phase 4: Quality Inspection & Shipping (1-3 days): Final dimensional checks, NDT review, documentation preparation, packaging, and shipping.
Total Lead Time:
- 프로토타입: For simple prototypes with minimal post-processing, lead times can be as short as 5-10 working days, showcasing AM’s rapid manufacturing capability.
- Production Parts (Low Volume): For fins requiring full heat treatment, HIP, machining, and rigorous inspection, lead times of 4-8 weeks are more typical.
- Factors Extending Lead Time: High supplier workload, need for external specialized processing (HIP, coating), complex inspection requirements, unexpected build issues requiring restarts.
Obtaining Accurate Quotes:
To get an accurate rapid manufacturing quote, provide potential suppliers with:
- A 3D CAD model (STEP or native format).
- A 2D drawing specifying tolerances, critical dimensions, surface finish requirements, and material specifications.
- The required material (Ti-6Al-4V, Scalmalloy®, etc.).
- Quantity needed.
- Required certifications and quality documentation.
- Desired delivery date.
By understanding these cost drivers and lead time components, organizations can better plan projects, negotiate with suppliers, and make informed decisions about adopting metal AM for demanding applications like missile fin production.
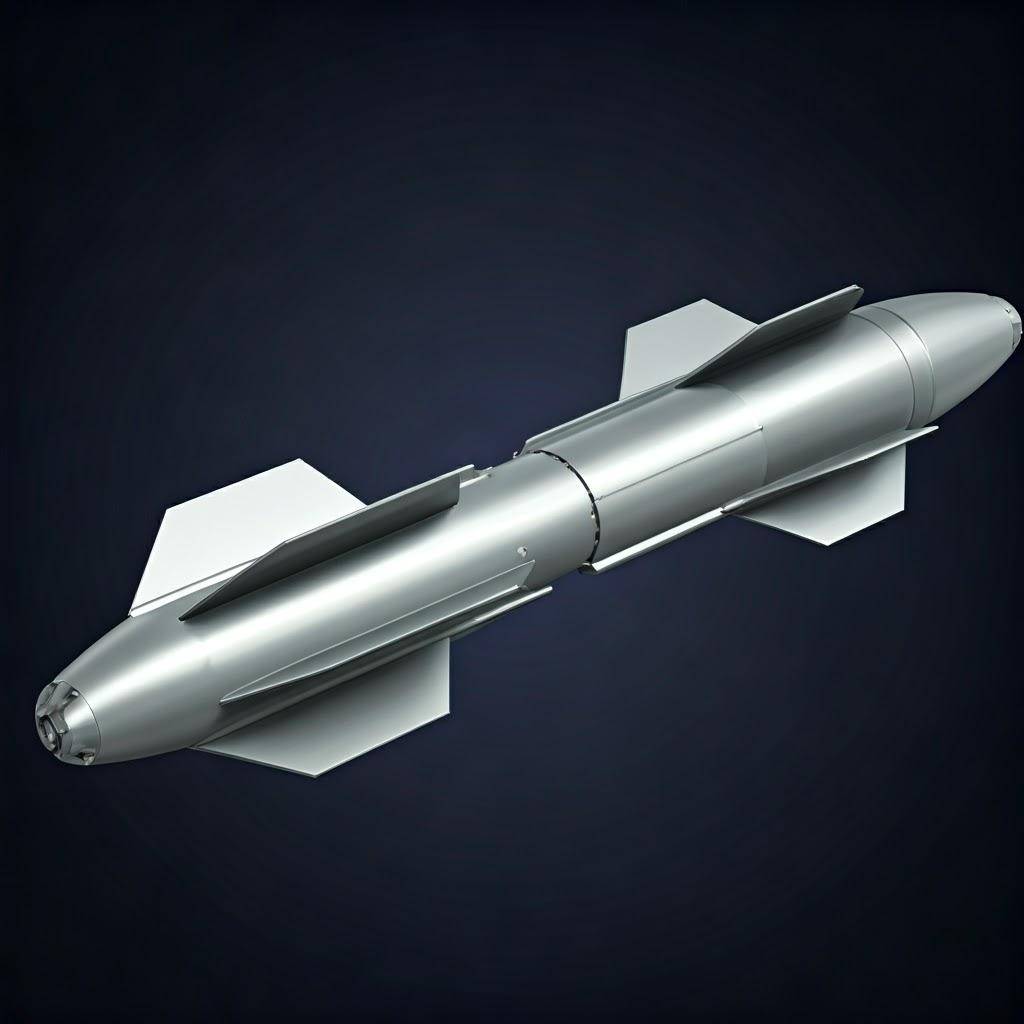
Frequently Asked Questions (FAQ) about 3D Printed Missile Fins
As metal additive manufacturing becomes increasingly adopted for critical aerospace and defense components, engineers, program managers, and procurement specialists often have pertinent questions regarding its capabilities, reliability, and comparison to traditional methods. Here are answers to some frequently asked questions about 3D printed missile fins:
1. How does the structural integrity and durability of 3D printed missile fins compare to those made by traditional CNC machining?
When manufactured using optimized and validated processes, the structural integrity and durability of metal AM missile fins can be comparable, and in some cases potentially superior, to their traditionally machined counterparts, especially when considering designs enabled 오직 by AM. Key points include:
- 머티리얼 속성: With proper process control and essential post-processing like Hot Isostatic Pressing (HIP) – particularly crucial for fatigue-critical materials like Ti-6Al-4V – AM parts can achieve densities exceeding 99.9% and mechanical properties (tensile strength, yield strength, elongation, fatigue life) that meet or exceed aerospace standards for wrought or cast materials. The rapid solidification in AM can create fine-grained microstructures that contribute to high strength.
- 피로 수명: HIPing is critical for eliminating internal micro-pores which act as crack initiation sites, drastically improving fatigue performance to levels required for aerospace applications. Rigorous testing and qualification are necessary to confirm fatigue life meets design requirements.
- 디자인 최적화: AM allows for topology optimization and part consolidation. This means fins can be designed to be inherently stronger and lighter by placing material exactly where needed and eliminating joints or fasteners that can be weak points in assemblies.
- Process Control is Key: Achieving these results depends entirely on using high-quality powder, optimized machine parameters, appropriate heat treatments, and rigorous quality control throughout the process chain. Working with an experienced provider like Met3dp, who understands these nuances, is essential. In summary, properly executed AM can produce fins that are not only structurally sound but potentially lighter and more performance-optimized than conventionally manufactured equivalents.
2. Are high-performance materials like Ti-6Al-4V and Scalmalloy® properly certified for aerospace applications when processed using Additive Manufacturing?
Yes, materials processed via AM can achieve aerospace certification, but it requires a stringent approach to process control, validation, and adherence to industry standards.
- 재료 사양: Established aerospace material specifications (e.g., AMS standards for Ti-6Al-4V) often have specific requirements for parts produced by additive manufacturing, or new AM-specific standards are being developed and adopted (e.g., SAE AMS7000 series). These standards define required chemical composition, microstructure, mechanical properties (tensile, fatigue, fracture toughness), and acceptable defect levels.
- 프로세스 자격: Certification is typically tied to a specific, validated manufacturing process. This involves qualifying the specific AM machine, the powder batch characteristics, the optimized build parameters, and the entire post-processing workflow (including heat treatment, HIP, machining). Extensive testing (material coupons, witness samples, potentially full component tests) is performed to demonstrate that the process consistently produces parts meeting the required specifications.
- Quality Management System: A certified QMS (like AS9100) is fundamental, ensuring traceability, process control, documentation, and consistent execution of the qualified process.
- Supplier Responsibility: Reputable AM service providers serving the aerospace industry, such as Met3dp, invest heavily in process development, material characterization, and achieving the necessary qualifications and certifications for the materials they offer. Clients should always verify that their supplier can provide parts manufactured under a process qualified to the relevant aerospace standards and deliver the required material certifications and conformity documentation.
3. What is the typical cost comparison between producing missile fins using metal AM versus traditional CNC machining?
There’s no single answer, as the cost-effectiveness depends heavily on several factors, primarily part complexity 및 production volume.
- 복잡성:
- High Complexity: For fins with highly complex geometries (e.g., topology optimized shapes, internal lattice structures, integrated features, complex curvatures) that are very difficult or impossible to machine, AM is often significantly more cost-effective, even at low volumes. Machining such parts would require extensive setup time, multi-axis machines, specialized tooling, and potentially multiple setups or assembly steps, driving up costs rapidly.
- Low Complexity: For relatively simple fin shapes that can be easily machined from standard stock material with minimal setups, CNC machining is generally more cost-effective, especially as volume increases.
- 생산량:
- Prototypes & Low Volume (e.g., 1-50 units): AM excels here due to the lack of tooling costs. Setting up for AM is relatively fast compared to designing fixtures or molds. For complex parts, AM is almost always cheaper at this volume. For simple parts, AM might still be competitive or slightly more expensive, but the lead time advantage can be significant.
- Medium Volume (e.g., 50-500 units): This is often a crossover range. For complex parts, AM may still hold an advantage. For simpler parts, the efficiencies of dedicated CNC setups start to outweigh AM costs.
- High Volume (e.g., 1000+ units): Traditional methods like high-speed CNC machining or potentially casting/forging (if geometry allows and tooling is justified) typically become more economical for simpler geometries due to faster cycle times per part once production is running. AM machine time remains a primary cost driver.
- Other Factors: Material waste (buy-to-fly ratio) can favor AM for expensive materials like titanium if the machined part generates significant scrap. The potential for lightweighting via AM can lead to downstream system-level benefits (range, payload) that justify a potentially higher component cost.
본질적으로: Use AM when complexity is high, volumes are low-to-medium, rapid prototyping is needed, or design features impossible with traditional methods offer significant performance benefits. Use CNC machining when geometry is simpler, volumes are high, and tooling costs can be amortized effectively.
4. How are export controls and security handled for sensitive defense components like missile fins manufactured using AM?
Handling sensitive defense components requires strict adherence to export control regulations and robust security protocols.
- ITAR/Export Controls: For parts subject to regulations like the US International Traffic in Arms Regulations (ITAR) or similar national controls, the AM service provider must be registered (if required, e.g., ITAR registration in the US) and have implemented strict procedures for:
- Data Handling: Secure transfer, storage, and access control for controlled technical data (CAD files, drawings, specifications). Encryption and secure networks are essential.
- Personnel: Ensuring only authorized personnel (e.g., US persons for ITAR) have access to controlled data and hardware.
- 제조: Secure manufacturing environment with access controls.
- 해운: Proper licensing and documentation for shipping controlled hardware.
- Confidentiality (NDAs): Non-Disclosure Agreements are standard practice to protect the client’s intellectual property, regardless of export controls.
- Facility Security: Physical security measures to prevent unauthorized access to design information or parts.
- Supplier Verification: Clients must verify that their chosen AM provider has the necessary registrations, security infrastructure, and compliance programs in place 전에 sharing controlled technical data or placing orders for controlled hardware. This is a critical step in supplier evaluation for defense projects.
Choosing a partner like Met3dp, who understands the requirements of working with international clients and has robust internal systems, provides assurance in handling projects with the necessary security and compliance, even if specific regulations like ITAR primarily apply to US defense articles.
Conclusion: The Future of Missile Component Manufacturing with Metal Additive Manufacturing
The landscape of aerospace and defense manufacturing is undergoing a profound transformation, driven by the imperative for higher performance, faster innovation cycles, and more resilient supply chains. Metal additive manufacturing stands at the forefront of this evolution, offering unprecedented capabilities for producing critical components like missile fins. As we’ve explored, the advantages are compelling: the ability to create highly complex, topology-optimized geometries for unparalleled lightweighting; the utilization of advanced, high-strength materials like Ti-6Al-4V and Scalmalloy® processed to meet demanding operational conditions; the acceleration of development through rapid prototyping; and the potential for on-demand production closer to the point of need.
However, realizing these benefits requires moving beyond viewing AM as merely a prototyping tool. It demands a holistic approach embracing Design for Additive Manufacturing (DfAM) principles, meticulous material selection and control, rigorous process optimization, comprehensive post-processing, and unwavering commitment to quality assurance through advanced inspection and validation. Overcoming challenges related to residual stress, porosity, precision, and repeatability is paramount, particularly for flight-critical hardware where failure is not an option.
The journey to successfully implementing metal AM for components like missile fins necessitates strategic partnerships. Selecting a service provider is not just a procurement decision; it’s an investment in expertise, capability, and trust. The ideal partner possesses not only state-of-the-art equipment but also deep materials science knowledge, proven aerospace process control, essential certifications like AS9100, robust quality management systems, and the collaborative spirit to assist with design optimization and problem-solving.
Met3dp embodies this philosophy, positioning itself as a leader in the field by integrating advanced metal powder production using Gas Atomization and PREP technologies with industry-leading printing systems and comprehensive application support. With decades of collective expertise, Met3dp delivers cutting-edge systems and high-quality powders, enabling next-generation manufacturing for aerospace, medical, automotive, and industrial partners worldwide. Their commitment extends beyond simply manufacturing parts; they partner with organizations to accelerate digital manufacturing transformations.
The future of missile component manufacturing will undoubtedly see increased adoption of metal AM. As the technology continues to mature, print speeds increase, material portfolios expand, and process controls become even more sophisticated, AM will unlock further performance enhancements and manufacturing efficiencies. It will enable the rapid deployment of next-generation defense systems characterized by greater speed, agility, range, and precision – performance attributes heavily influenced by components like advanced, additively manufactured fins.
For engineers and procurement managers in the aerospace and defense sectors, embracing metal AM is no longer a question of ‘if’, but ‘how’. By understanding its capabilities, acknowledging its challenges, and selecting the right strategic partners, organizations can leverage this transformative technology to gain a decisive competitive edge and shape the future of high-performance flight systems.
To explore how Met3dp’s capabilities in advanced metal powders and additive manufacturing solutions can power your organization’s goals, visit https://met3dp.com/ or contact their team today.
공유
페이스북
트위터
LinkedIn
WhatsApp
이메일
중국 칭다오에 본사를 둔 선도적인 적층 제조 솔루션 제공업체인 MET3DP Technology Co. 당사는 산업용 3D 프린팅 장비와 고성능 금속 분말을 전문으로 합니다.
관련 기사
Met3DP 소개
최근 업데이트
제품
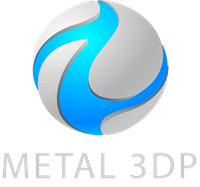
3D 프린팅 및 적층 제조용 금속 분말
문의 정보
- 칭다오시, 산둥성, 중국
- [email protected]
- [email protected]
- +86 19116340731