Custom Heat Shields for Engines via Metal 3D Printing
Índice
Introduction: Revolutionizing Engine Thermal Management with Custom Metal 3D Printed Heat Shields
In the high-stakes environments of aerospace, automotive, and industrial applications, effective thermal management is not just a performance enhancer – it’s a critical necessity. Engines, by their very nature, generate immense heat, posing significant risks to surrounding components, compromising efficiency, and potentially leading to catastrophic failures if not properly managed. Engine heat shields are the unsung heroes in this thermal battleground, acting as crucial barriers that protect sensitive electronics, fluid lines, structural elements, and even passenger compartments from excessive temperatures. Traditionally, manufacturing these vital components, especially those with complex geometries required for tightly packed modern engine bays, has involved costly tooling, long lead times, and design compromises inherent in methods like stamping or hydroforming. However, a paradigm shift is underway, driven by the advancements in fabricación aditiva (AM) de metalescomúnmente conocido como metal Impresión 3D.
This transformative technology unlocks unprecedented design freedom, enabling the creation of highly customized, performance-optimized engine heat shields that were previously impossible or prohibitively expensive to produce. By building parts layer by layer directly from digital designs using high-performance metal powders, metal AM eliminates the need for traditional tooling, drastically reduces lead times for prototypes and custom series, and facilitates the integration of complex features like internal cooling channels or weight-saving lattice structures. For engineers striving for optimal packaging and thermal efficiency, and for procurement managers seeking agile, cost-effective custom component sourcing, metal 3D printing offers a compelling solution for next-generation gestión térmicaEmpresas como Met3dp, with deep expertise in both advanced metal powders and industrial printing systems, are at the forefront, empowering industries to leverage metal AM for superior heat shield solutions. This article delves into the critical role of engine heat shields, explores the limitations of conventional manufacturing, highlights the compelling advantages of metal 3D printing, and guides you through material selection for these demanding applications.
What Are Engine Heat Shields and Why Are They Critical?
An engine heat shield is essentially a thermal barrier designed to reflect, absorb, or dissipate heat generated by an engine and its associated components, primarily the exhaust system (manifolds, catalytic converters, mufflers). Its primary function is to protect surrounding parts and systems from the damaging effects of high temperatures. The importance of these shields cannot be overstated across various sectors:
- Automóvil: In vehicles, heat shields protect under-hood components like wiring harnesses, fuel lines, brake lines, plastic reservoirs, sensors, and electronic control units (ECUs). They also prevent heat from radiating into the passenger cabin, ensuring comfort and safety, and shield the underbody and chassis components. The automotive heat shield is critical for vehicle longevity, reliability, and compliance with safety regulations.
- Aeroespacial: Within aircraft engines and auxiliary power units (APUs), aerospace engine components operate under extreme temperature variations. Heat shields are vital for protecting sensitive avionics, hydraulic lines, structural elements (like bulkheads and nacelles), and ensuring the overall integrity and safety of the flight systems. They play a crucial role in preventing heat damage that could compromise flight safety.
- Fabricación industrial: Stationary engines, generators, heavy machinery, and power generation equipment also rely heavily on heat shields. They protect operators, surrounding equipment, control systems, and infrastructure from intense heat, ensuring operational safety and preventing premature wear or failure of adjacent machinery.
- Motorsport: Performance racing applications push engines to their limits, generating extreme heat. Custom heat shields are essential for protecting critical systems, optimizing engine bay airflow, and ensuring consistent performance under demanding race conditions.
Key Functions of Engine Heat Shields:
- Thermal Protection: Blocking radiant and convective heat transfer to prevent overheating of nearby components.
- Performance Maintenance: Ensuring components like sensors and electronics operate within their optimal temperature range.
- Seguridad: Preventing fires by shielding flammable materials (fluids, insulation) and protecting personnel from burns.
- Durability Enhancement: Reducing thermal stress on surrounding parts, extending their lifespan.
- Cumplimiento de la normativa: Meeting industry standards for heat management and material temperature limits.
The geometry of heat shields is often complex, dictated by the tight packaging constraints within engine compartments. They need to conform closely to the heat source (e.g., exhaust manifold) while maintaining sufficient air gaps or insulation to effectively block heat transfer to the protected components. This complexity presents significant challenges for traditional manufacturing methods.
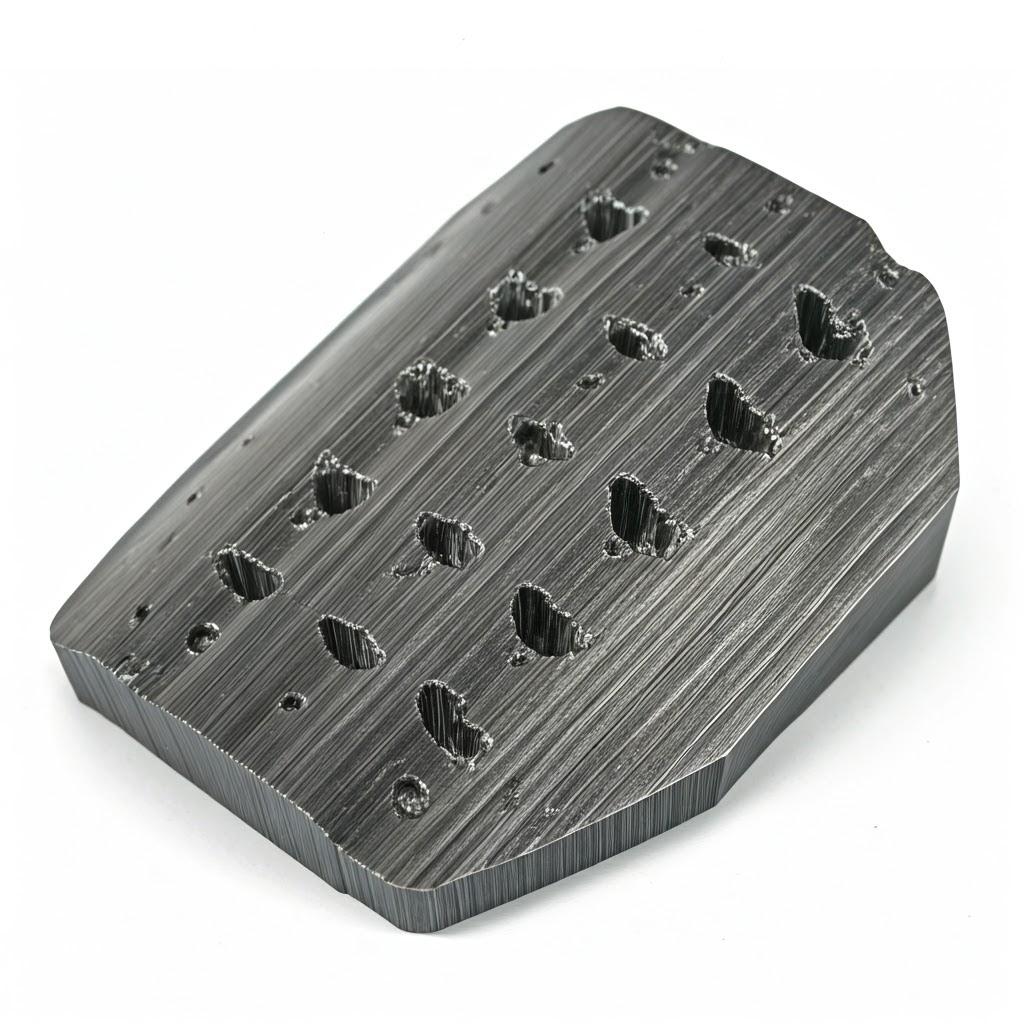
Limitations of Traditional Manufacturing for Complex Heat Shields
For decades, engine heat shields have predominantly been manufactured using conventional sheet metal techniques like stamping, hydroforming, and deep drawing. While effective for high-volume production of relatively simple designs, these methods encounter significant limitations when faced with the increasing complexity and customization demands of modern engines:
- High Tooling Costs: Creating the dies and molds required for stamping or forming is extremely expensive and time-consuming. This makes low-volume production runs or custom heat shield manufacturing economically unviable. Any design modification necessitates costly re-tooling.
- Long Lead Times: The process of designing, fabricating, and validating tooling can take weeks or even months, significantly delaying product development cycles and hindering rapid prototyping efforts.
- Design Constraints: Traditional forming methods have inherent limitations regarding geometric complexity. Achieving deep draws, sharp angles, undercuts, or integrated features like mounting bosses or internal structures is often difficult or impossible, forcing engineers to compromise on optimal thermal performance or packaging. Designs often need to be simplified to be manufacturable.
- Residuos materiales: Sheet metal processes often generate significant scrap material, particularly with complex shapes, increasing overall material costs and environmental impact.
- Assembly Complexity: Complex heat shields often require multiple stamped parts to be welded or fastened together, adding assembly steps, potential points of failure, and increased weight.
- Peso: Sheet metal parts, while relatively thin, may not be optimally designed for minimum weight, a critical factor in aerospace and performance automotive applications. Achieving lightweight designs often requires using thinner gauges, which can compromise stiffness or thermal performance.
These limitations make it challenging for manufacturers to quickly iterate on designs, produce custom solutions for niche applications, or optimize heat shields for maximum thermal efficiency and minimal weight using traditional approaches. This is precisely where the disruptive potential of impresión 3D en metal emerges.
The Additive Advantage: Why Choose Metal 3D Printing for Engine Heat Shields?
Metal additive manufacturing offers a powerful alternative for producing engine heat shields, overcoming many limitations of conventional methods and unlocking significant benefits for engineers and manufacturers:
- Libertad de diseño sin igual: AM builds parts layer-by-layer directly from a CAD file, enabling the creation of highly complex geometries without the constraints of traditional tooling. This allows for:
- Conformal Designs: Shields that perfectly follow the contours of engine components for maximum efficiency in tight spaces.
- Integrated Features: Mounting points, brackets, stiffening ribs, and even logos can be built directly into the part, reducing assembly complexity.
- Internal Structures: Ligero lattice structures or internal cooling channels can be incorporated to enhance thermal performance and reduce mass simultaneously.
- Optimización de la topología: Algorithms can be used to remove material from low-stress areas, creating organically shaped, highly efficient, and lightweight structures impossible to make otherwise.
- Creación rápida de prototipos e iteración: New heat shield designs can be printed and tested in days rather than weeks or months. This accelerates development cycles, allows for quick validation of thermal performance, and enables engineers to refine designs rapidly based on real-world testing. Rapid prototyping heat shields becomes feasible and cost-effective.
- Eliminación de herramientas: AM requires no part-specific tooling (dies, molds). This drastically reduces upfront costs and lead times, making it ideal for:
- Custom and Low-Volume Production: Manufacturing bespoke heat shields for specialized vehicles, prototypes, or aftermarket applications.
- Fabricación a la carta: Producing parts only when needed, reducing inventory costs and enabling virtual warehousing.
- Potencial de aligeramiento: Through topology optimization and the use of lattice structures, metal AM can produce heat shields that are significantly lighter than their traditionally manufactured counterparts while maintaining or even improving structural integrity and thermal performance. This is crucial for aerospace and performance automotive sectors where every gram matters.
- Versatilidad de materiales: AM processes can utilize a wide range of high-performance metal powders, including aluminum alloys, titanium alloys, and nickel superalloys (like those expertly produced by Met3dp using advanced gas atomization), allowing engineers to select the optimal material based on specific temperature requirements, weight targets, and environmental conditions.
- Consolidación de piezas: Complex assemblies that previously required multiple components and fasteners can often be consolidated into a single, integrated 3D printed part, simplifying assembly, reducing part count, and potentially improving reliability.
- Optimización del rendimiento: The design freedom allows for the creation of heat shields with superior thermal insulation or dissipation properties, tailored precisely to the specific application’s needs.
By leveraging these advantages, manufacturers can produce custom engine heat shields that offer superior performance, reduced weight, and faster development timelines compared to traditional methods, providing a significant competitive edge in demanding industries.
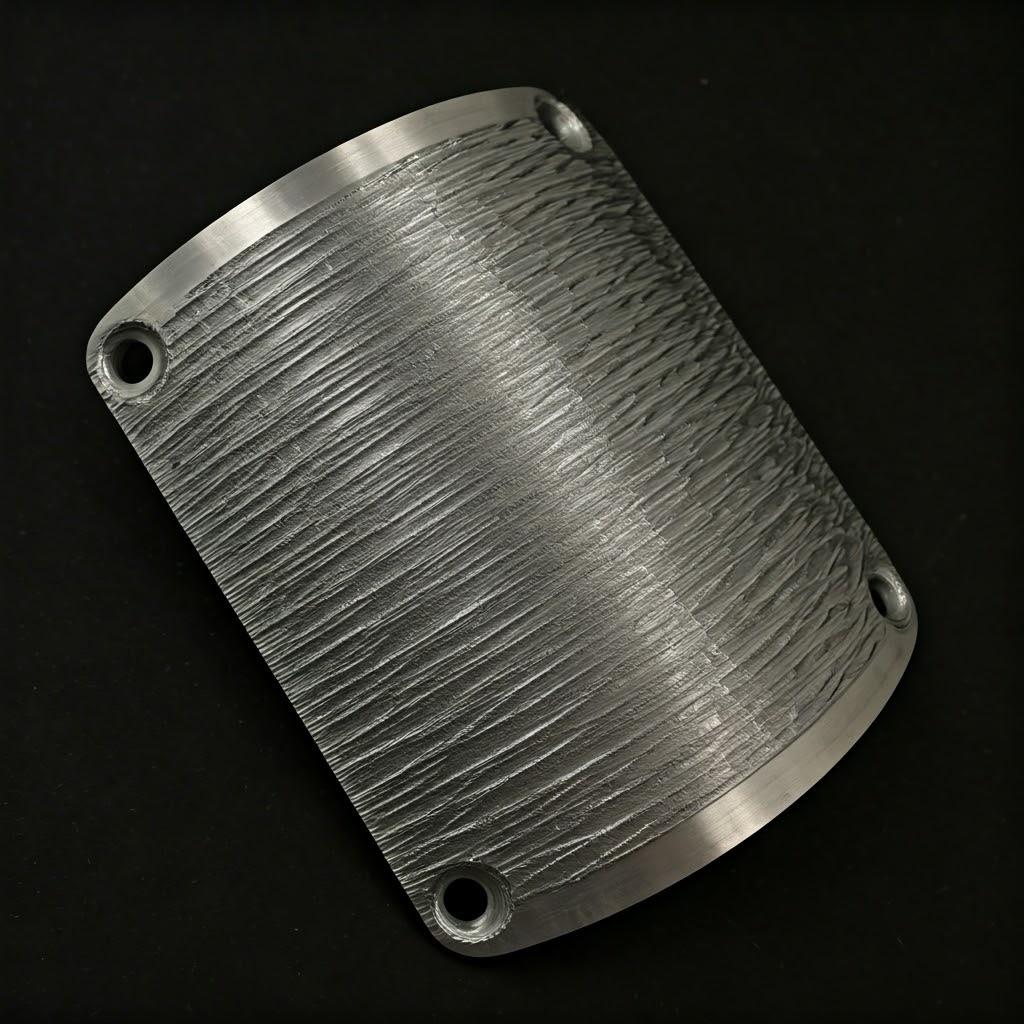
Material Deep Dive: Selecting the Right Metal Powders for Optimal Performance (AlSi10Mg, Ti-6Al-4V, IN625)
Choosing the correct material is paramount for the performance and longevity of an engine heat shield. Metal additive manufacturing offers a diverse palette of materials, but for heat shield applications, specific properties like temperature resistance, weight, corrosion resistance, and thermal conductivity are key decision drivers. The recommended powders – AlSi10Mg, Ti-6Al-4V, and IN625 – represent a spectrum of capabilities suitable for various engine environments. Sourcing high-quality, consistent metal powders is crucial for successful printing, and companies like Met3dp, utilizing advanced tecnologías de atomización de gas y PREP, ensure the production of highly spherical powders with excellent flowability, essential for achieving dense, high-performance parts. Let’s examine the characteristics of these key heat shield materials:
- AlSi10Mg (Aluminum Alloy):
- Propiedades: This aluminum-silicon-magnesium alloy is known for its good strength-to-weight ratio, excellent thermal conductivity, and relatively good corrosion resistance. It’s one of the most commonly used aluminum alloys in additive manufacturing.
- Heat Shield Suitability: Best suited for applications with moderate temperature requirements (typically up to 150-200°C, though suitability depends on load and duration). Its high thermal conductivity can be leveraged in designs aiming to dissipate heat rapidly. Its primary advantage is its low density, making it ideal for aligeramiento components in automotive or aerospace applications where temperatures aren’t extreme.
- Consideraciones: Its lower melting point compared to titanium or nickel alloys restricts its use in proximity to very high-temperature sources like exhaust manifolds or turbochargers without significant air gaps or insulation design features.
- Met3dp Advantage: Sourcing consistent AlSi10Mg powder with controlled particle size distribution, like that offered by Met3dp, is vital for achieving optimal mechanical properties and surface finish in printed parts.
- Ti-6Al-4V (Titanium Alloy – Grade 5):
- Propiedades: This is the workhorse titanium alloy, offering an excellent combination of high strength, low density (about 40% lighter than steel), outstanding corrosion resistance, and good elevated temperature performance (service temperatures typically up to 350-400°C, potentially higher for short durations).
- Heat Shield Suitability: Ti-6Al-4V is an excellent choice for applications requiring significant weight savings combined with higher temperature resistance than aluminum alloys can offer. It’s widely used in aerospace engine components and performance automotive exhaust systems where both heat tolerance and minimal mass are critical. Its low thermal conductivity also makes it effective as a thermal barrier, slowing heat transfer.
- Consideraciones: Titanium alloys are more expensive than aluminum and require specific handling and post-processing (like stress relief in a vacuum or inert atmosphere) to maintain optimal properties. Printing requires careful parameter control to manage residual stresses.
- Met3dp Advantage: Met3dp possesses significant expertise in processing reactive materials like titanium alloys, offering high-purity Ti-6Al-4V powder and optimized printing parameters on their advanced systems to ensure component integrity.
- IN625 (Inconel 625 – Nickel Superalloy):
- Propiedades: Inconel 625 is a nickel-chromium superalloy renowned for its exceptional high-temperature strength, excellent corrosion and oxidation resistance, and fatigue strength. It maintains its properties over a very wide temperature range, performing reliably at cryogenic temperatures up to 980°C (1800°F) or even higher for short exposures.
- Heat Shield Suitability: IN625 is the go-to material for the most demanding aplicaciones de alta temperatura. It’s ideal for heat shields placed very close to exhaust manifolds, turbochargers, gas turbine components, and other extreme heat sources. Its combination of thermal stability and resistance to harsh exhaust gas environments makes it exceptionally durable.
- Consideraciones: IN625 is denser and significantly more expensive than aluminum or titanium alloys. It also requires careful optimization of printing parameters and specific heat treatments to achieve desired microstructure and properties. Its lower thermal conductivity compared to aluminum is beneficial for barrier-type heat shields.
- Met3dp Advantage: Manufacturing and printing nickel superalloys like IN625 demand advanced powder production techniques and sophisticated printing equipment. Met3dp’s capabilities in producing high-quality superalloy powders and its industry-leading printers ensure reliable manufacturing of mission-critical parts from materials like IN625.
Material Selection Summary Table:
Característica | AlSi10Mg | Ti-6Al-4V | IN625 (Inconel 625) |
---|---|---|---|
Beneficio principal | Lightweight, Cost-effective | High Strength-to-Weight, Good Temp. Resistance | Excellent High-Temp. Strength & Corrosion Resistance |
Densidad | Low (~2.68 g/cm³) | Medium (~4.43 g/cm³) | High (~8.44 g/cm³) |
Max Service Temp. | Moderate (~150-200°C) | High (~350-400°C) | Very High (~980°C+) |
Thermal Cond. | Alta | Bajo | Bajo |
Corrosion Res. | Bien | Excelente | Excelente |
Coste relativo | Bajo | Medio-Alto | Alta |
Typical Use Case | General automotive, Moderate temp zones | Aerospace, Performance Automotive, Exhaust components | Extreme Heat Zones (Turbochargers, Manifolds, Turbines) |
Exportar a hojas
Selecting the right material involves balancing performance requirements (temperature, strength, corrosion), weight targets, and budget constraints. Consulting with experienced proveedores de servicios de metal AM like Met3dp can provide valuable insights into material suitability and process optimization for your specific heat shield application.
Designing for Additive Manufacturing (DfAM): Optimizing Heat Shields for Printability and Function
Simply converting a conventionally designed heat shield to a 3D printable format rarely unlocks the full potential of additive manufacturing. Designing para Additive Manufacturing (DfAM) involves leveraging the unique capabilities of the process while mitigating its constraints. Applying DfAM principles is crucial for creating engine heat shields that are not only manufacturable but also functionally superior and cost-effective.
Key DfAM Considerations for Heat Shields:
- Optimización de la topología: Use software tools to algorithmically remove material from areas experiencing low stress, resulting in organic, lightweight structures that meet performance requirements with minimum mass. This is ideal for creating highly efficient, stiff, and lightweight heat shields.
- Estructuras reticulares: Incorporate internal or external lattice structures to significantly reduce weight while maintaining structural integrity. These can also be designed to manipulate airflow for enhanced cooling or insulation, adding functional benefits beyond simple mass reduction.
- Consolidación de piezas: Redesign assemblies comprising multiple sheet metal pieces and fasteners into a single, integrated AM part. This reduces assembly time, eliminates potential failure points at joints, and often results in a lighter, stiffer component.
- Conformal Design: Take advantage of AM’s ability to create freeform shapes. Design heat shields that conform perfectly to complex engine or exhaust geometries, maximizing space utilization and thermal shielding effectiveness.
- Espesor de pared: Maintain appropriate minimum wall thickness suitable for the chosen material and AM process (e.g., Laser Powder Bed Fusion – LPBF). Thin walls can be challenging to print without warping or defects, while excessively thick sections increase print time and material consumption. Heat shields often benefit from thin walls for weight, but design must ensure printability and structural stability.
- Estructuras de apoyo: Metal AM processes typically require support structures to anchor the part to the build plate, manage thermal stresses, and support overhanging features (typically angles less than 45 degrees from the horizontal).
- Minimize the need for supports by orienting the part strategically on the build plate.
- Design supports for easy removal without damaging critical surfaces.
- Consider self-supporting angles (greater than 45 degrees) where possible.
- Thermal Management during Print: Large, flat sections common in heat shields can be prone to warping due to thermal stresses during the printing process. Incorporating design features like reinforcing ribs or optimizing print orientation can help mitigate this.
- Hole Orientation and Size: Small holes, especially horizontal ones, can be challenging to print accurately without supports. Consider designing holes vertically or using teardrop shapes for self-supporting horizontal holes if precise dimensions are not critical.
- Surface Features: Integrate mounting bosses, locating pins, stiffening ribs, or even airflow-directing features directly into the design.
Engaging with AM experts early in the design phase is highly recommended. Met3dp offers application development services, helping clients optimize their heat shield designs for additive manufacturing, ensuring printability, performance, and cost-effectiveness by leveraging advanced techniques like topology optimization and simulation.
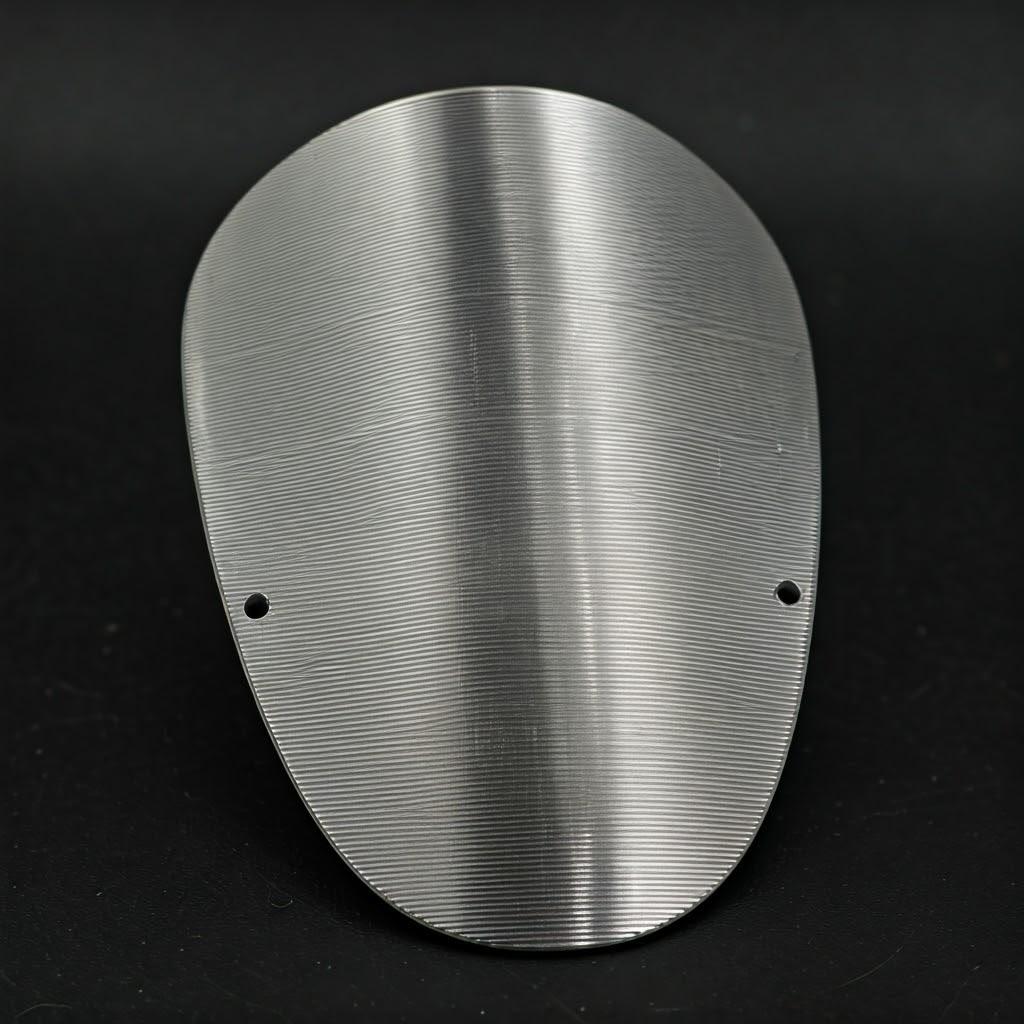
Achievable Tolerances, Surface Finish, and Dimensional Accuracy in Metal AM Heat Shields
Understanding the achievable precision is crucial when specifying metal 3D printed heat shields. While metal AM offers remarkable capabilities, it’s important to have realistic expectations regarding tolerances and surface finish compared to high-precision CNC machining.
- Tolerances: Typical dimensional accuracy for metal AM processes like Laser Powder Bed Fusion (LPBF) or Electron Beam Melting (EBM) is often in the range of ±0.1 mm to ±0.2 mm or ±0.1% to ±0.2% of the nominal dimension, whichever is greater. Tighter tolerances are possible but may require specific process controls or post-machining. Factors influencing final accuracy include:
- Material properties (thermal expansion, shrinkage)
- Part geometry and size
- Printer calibration and condition (Met3dp printers, known for industry-leading accuracy and reliability, help ensure consistent results)
- Print parameters and support strategy
- Post-processing steps (heat treatment can cause minor distortion)
- Acabado superficial (rugosidad): The as-built surface finish of metal AM parts is inherently rougher than machined surfaces due to the layer-by-layer process and partially melted powder particles adhering to the surface.
- Typical as-built surface roughness (Ra) ranges from 6 µm to 25 µm, depending on the material, process, orientation of the surface relative to the build direction (upward-facing surfaces are generally smoother than downward-facing ones), and print parameters.
- For many heat shield applications, the as-built finish may be perfectly acceptable, especially on non-critical surfaces.
- If a smoother finish is required for aesthetic reasons, improved fatigue life, or specific mating surfaces, post-processing techniques like bead blasting, tumbling, polishing, or CNC machining are necessary.
- Geometric Dimensioning and Tolerancing (GD&T): Apply GD&T specifications to drawings just as you would for conventionally manufactured parts, clearly indicating critical dimensions, datums, and required tolerances. This ensures the AM provider understands the critical features and can plan the manufacturing process accordingly.
It’s essential to discuss specific tolerance and surface finish requirements with your AM service provider early on. They can advise on the achievable precision with their equipment and processes and recommend appropriate post-processing steps if tighter specifications are needed. Met3dp’s focus on fabricación de precisión ensures that parts meet demanding industry requirements, leveraging their advanced printing systems and process control.
Essential Post-Processing Steps for 3D Printed Metal Heat Shields
Most metal 3D printed parts, including heat shields, require some form of post-processing after removal from the build plate to achieve the desired final properties, tolerances, and surface finish. The specific steps depend on the material, design complexity, and application requirements.
Common Post-Processing Operations:
- Alivio del estrés / Tratamiento térmico: Este suele ser el first and most crucial step. The rapid heating and cooling cycles during printing induce internal stresses within the part. Tratamiento térmico in a controlled atmosphere furnace (vacuum or inert gas for reactive materials like Ti-6Al-4V) relieves these stresses, preventing distortion or cracking during support removal or subsequent machining, and stabilizing the material’s microstructure. Specific heat treatment cycles (e.g., annealing, solution treatment, aging) may also be required to achieve the desired final mechanical properties (strength, hardness, ductility) for the chosen alloy.
- Retirada del soporte: The structures supporting the part during printing must be carefully removed. This can be done manually (using pliers, cutters) or through machining (milling, grinding, wire EDM), depending on the support design, location, and accessibility. Careful removal is necessary to avoid damaging the part surface.
- Extracción de la placa de construcción: The part needs to be separated from the build plate, typically using wire EDM or band sawing.
- Acabado superficial:
- Cleaning/Blasting: Bead blasting, sandblasting, or shot peening is commonly used to remove loose powder, smooth surfaces slightly, and create a uniform matte finish.
- Tumbling/Vibratory Finishing: Useful for smoothing surfaces and deburring edges on batches of smaller parts.
- Pulido: Manual or automated polishing can achieve very smooth, reflective surfaces if required for aesthetics or specific functional reasons (e.g., reflecting radiant heat).
- Mecanizado CNC: If critical dimensions require tolerances tighter than achievable with AM alone, or if specific features (e.g., mating surfaces, precise hole diameters) are needed, Mecanizado CNC is employed. This selectively removes material from the AM part to achieve final specifications.
- Prensado isostático en caliente (HIP): For critical applications, especially in aerospace, HIP may be used. This process applies high temperature and isostatic gas pressure to eliminate any residual internal microporosity, improving fatigue life, ductility, and ensuring maximum density.
- Revestimiento: Depending on the application, heat shield coatings (e.g., ceramic thermal barrier coatings – TBCs, high-emissivity coatings) may be applied to further enhance thermal performance or provide additional environmental protection.
- Inspection and Quality Control: Dimensional checks (using CMM, scanners), material testing, and non-destructive testing (NDT) like CT scanning may be performed to ensure the part meets all specifications.
Understanding these servicios de posprocesamiento and their impact on cost and lead time is essential when planning a project. Collaborating with a full-service provider like Met3dp, which possesses expertise across the entire AM workflow from powder to finished part, ensures that all necessary steps are performed correctly to deliver a heat shield meeting the required standards.
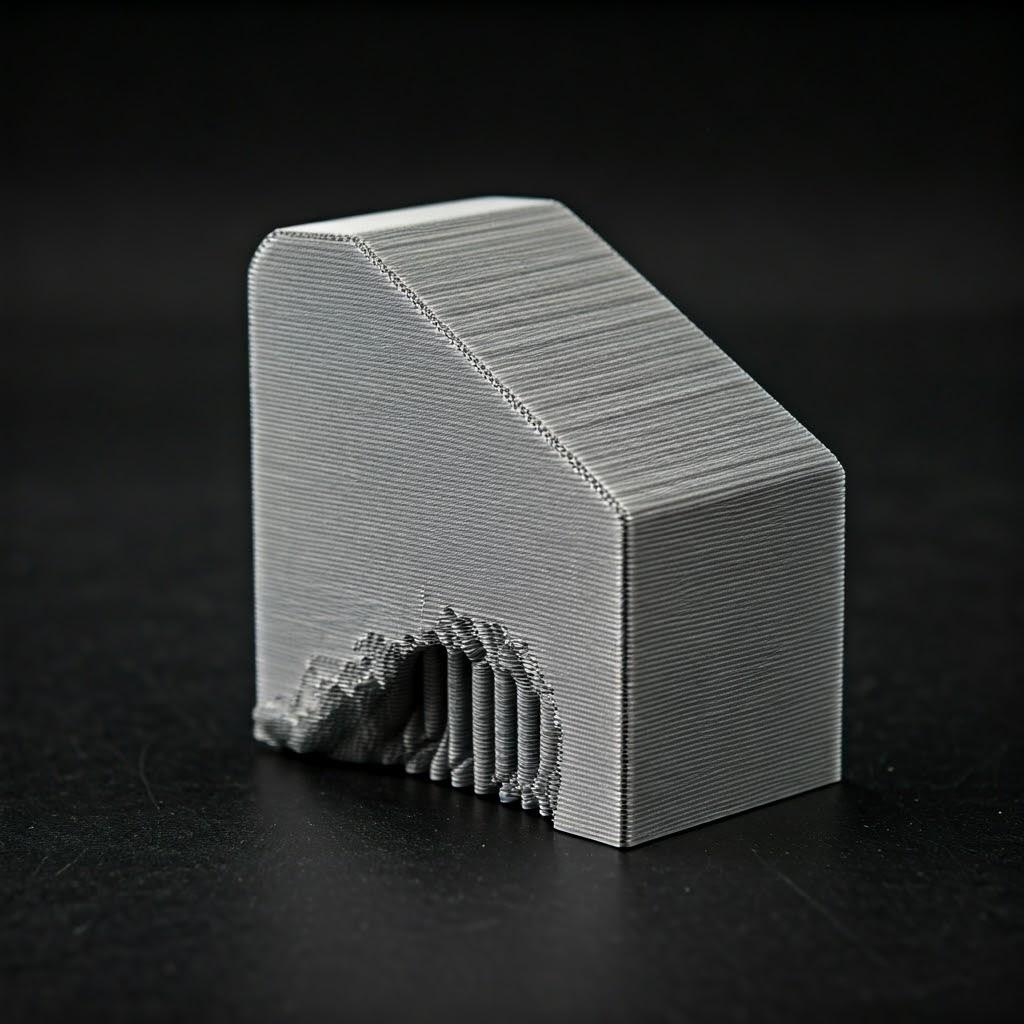
Navigating Challenges: Overcoming Potential Hurdles in Metal AM for Heat Shields
While metal additive manufacturing offers significant advantages for producing engine heat shields, it’s not without its challenges. Understanding these potential hurdles and collaborating with an experienced AM partner is key to successful implementation. Forewarned is forearmed, and proactive planning can mitigate most issues.
Common Challenges and Mitigation Strategies:
- Deformación y distorsión:
- Desafío: The significant temperature gradients during printing can cause internal stresses, leading thin, flat sections characteristic of many heat shields to warp or distort, especially upon removal from the build plate.
- Mitigación:
- Optimized Orientation: Carefully selecting the part orientation on the build plate can minimize stress accumulation in critical areas.
- Simulación térmica: Using simulation software to predict stress buildup and optimize the print strategy.
- Estrategia de apoyo: Employing robust but strategically placed support structures to anchor the part and dissipate heat effectively.
- Design Features: Incorporating slight curves, reinforcing ribs, or corrugations into the design can increase stiffness and resist warping.
- Process Parameter Control: Utilizing optimized printing parameters developed for the specific material and geometry, leveraging the precision control offered by systems like those from Met3dp.
- Alivio del estrés: Performing a proper stress relief heat treatment before removing the part from the build plate is crucial.
- Retirada de la estructura de soporte:
- Desafío: Supports are necessary but must be removed without damaging the part’s surface or leaving residual marks, especially on functional or visible areas. Dense or poorly designed supports can be difficult and time-consuming to remove.
- Mitigación:
- DfAM for Supports: Designing parts to minimize the need for supports in critical areas (e.g., using self-supporting angles >45°).
- Optimized Support Design: Utilizing specialized support structures (e.g., tree supports, block supports with perforation) designed for easier removal and minimal contact points on the part surface.
- Appropriate Removal Techniques: Employing careful manual removal or precise machining (EDM, milling) based on support type and location.
- Acabado superficial: Planning for subsequent surface finishing steps (blasting, polishing) to address any minor marks left after support removal.
- Control de la porosidad:
- Desafío: Microscopic voids or pores can sometimes form within the printed material if printing parameters are not fully optimized, potentially affecting mechanical properties, particularly fatigue life.
- Mitigación:
- Polvo de alta calidad: Using high-quality, spherical metal powders with consistent particle size distribution and low gas content, such as those produced via Met3dp’s advanced atomization processes, is fundamental. Explore Met3dp’s Product offerings for suitable powders.
- Parámetros de impresión optimizados: Precisely controlling laser/electron beam power, scan speed, layer thickness, and atmosphere conditions to ensure full melting and consolidation.
- Prensado isostático en caliente (HIP): For critical applications demanding maximum density and fatigue performance, HIP post-processing can effectively eliminate residual porosity.
- Achieving Desired Surface Finish:
- Desafío: The inherent layer-by-layer nature of AM results in a rougher surface finish compared to machining. Meeting stringent surface roughness requirements often necessitates additional steps.
- Mitigación:
- Orientación: Printing critical surfaces at optimal angles relative to the build direction can improve their as-built finish.
- Parameter Tuning: Fine-tuning print parameters can influence the resulting surface roughness.
- Post-procesamiento: Implementing appropriate surface finishing techniques (bead blasting, tumbling, polishing, machining) tailored to the specific requirements.
- Residual Stress Management:
- Desafío: Beyond warping, residual stresses can impact the part’s dimensional stability and mechanical performance over time if not properly managed.
- Mitigación:
- Estrategias de exploración optimizadas: Using specific laser/beam scanning patterns (e.g., island scanning) to distribute heat more evenly.
- Pre-heating: Some processes (like EBM) utilize build chamber pre-heating to reduce thermal gradients.
- Mandatory Stress Relief: Implementing a validated stress relief heat treatment cycle appropriate for the alloy is non-negotiable for most metal AM parts.
Successfully navigating these challenges requires deep process knowledge, robust quality control, and advanced equipment. Partnering with an established metal AM service provider with proven expertise in the specific materials and application types is the most effective way to ensure high-quality, reliable heat shields.
Selecting Your Metal AM Partner: Criteria for Choosing a Heat Shield Supplier
Choosing the right additive manufacturing partner is as critical as selecting the right material or optimizing the design. The quality of your 3D printed heat shields depends heavily on the capabilities, expertise, and quality systems of your chosen metal 3D printing service provider. When evaluating potential suppliers, consider the following criteria:
- Technical Expertise and Experience:
- Does the provider have demonstrated experience printing the specific metal alloys required (AlSi10Mg, Ti-6Al-4V, IN625)?
- Do they have application engineers who understand thermal management challenges and can provide DfAM support?
- Have they successfully produced parts for similar demanding industries (aerospace, automotive)? Look for case studies or references.
- Equipment and Technology:
- What type of metal AM technology do they use (e.g., LPBF, EBM)? Is it suitable for your requirements? Learn more about different Métodos de impresión.
- Do they operate modern, well-maintained industrial-grade printers known for accuracy and reliability?
- What is their build volume capacity? Can they accommodate the size of your heat shield?
- Material Capabilities and Quality:
- Do they offer the specific, high-quality metal powders you need?
- Can they provide material certifications and traceability for the powders used?
- Do they have robust processes for powder handling and recycling to ensure material consistency? Met3dp’s integrated approach, manufacturing both powders and printers, offers significant advantages here.
- Capacidades de postprocesado:
- Can they provide the necessary post-processing steps in-house or through qualified partners (heat treatment, machining, surface finishing, HIP)?
- Do they have expertise in the specific requirements for each step (e.g., vacuum heat treatment for titanium)?
- Quality Management Systems:
- Are they certified to relevant quality standards (e.g., ISO 9001, AS9100 for aerospace)?
- What quality control and inspection processes do they employ (e.g., CMM, NDT, material testing)?
- Lead Time and Capacity:
- What are their typical plazos de entrega for prototypes and production runs?
- Do they have sufficient capacity to meet your volume requirements and timelines?
- Customer Service and Communication:
- Are they responsive, communicative, and collaborative?
- Do they provide clear quotes and project updates?
- Rentabilidad:
- While cost is important, evaluate it in the context of quality, reliability, and overall value. The cheapest option may not be the best if it compromises part performance or delivery schedules.
Met3dp stands out as a comprehensive metal AM solutions provider, offering not only state-of-the-art SEBM printers and a wide range of high-performance metal powders produced in-house but also deep application expertise and development services. Their commitment to quality and innovation makes them a strong partner for demanding heat shield applications across various industries.
Understanding Cost Drivers and Lead Times for 3D Printed Heat Shields
A common question from engineers and procurement managers revolves around the cost and turnaround time for metal 3D printed components. Understanding the key factors influencing metal 3D printing cost estimation y manufacturing lead time helps in project planning and budgeting.
Principales factores de coste:
- Tipo de material y consumo:
- Material Choice: High-performance alloys like IN625 and Ti-6Al-4V are significantly more expensive per kilogram than AlSi10Mg or steels.
- Part Volume: The actual volume of material used in the part directly impacts cost. Design optimization (e.g., topology optimization, lattices) can reduce volume and thus cost.
- Volumen de la estructura de soporte: Material used for support structures also adds to the cost. Efficient design minimizes support needs.
- Print Time:
- Tamaño de la pieza y complejidad: Larger and more complex parts take longer to print, occupying expensive machine time. The overall height (Z-dimension) in the build chamber is often a primary driver of print duration.
- Build Density: Printing multiple parts simultaneously in a single build job can reduce the per-part machine time cost.
- Requisitos de postprocesamiento:
- Each post-processing step (stress relief, support removal, machining, finishing, HIP, coating) adds labor, machine time, and potentially specialized vendor costs. Complex finishing or tight tolerance requirements significantly increase costs.
- Labor and Engineering:
- Includes file preparation, build setup, machine operation, part removal, quality inspection, and any required DfAM or engineering support.
- Garantía de calidad:
- Costs associated with specific inspection requirements (CT scanning, detailed CMM reports, material testing) add to the overall price.
Factors Influencing Lead Time:
- Print Time: As mentioned, this is often the longest single step in the process.
- Machine Availability: Queue times for available printers at the service provider.
- Post-Processing Duration: Heat treatment cycles, machining setup and runtime, finishing processes all add to the overall time. HIP can add several days.
- Part Complexity & Quantity: More complex parts may require more intricate post-processing; larger quantities naturally take longer to produce.
- Envío: Time required for transport from the AM provider to your facility.
Typical Lead Times:
- Prototipos: Can often be produced in a matter of days (e.g., 3-10 working days), depending on complexity, material, and required post-processing.
- Producción de bajo volumen: May range from 2 to 6 weeks, depending on quantity and process requirements.
It’s crucial to get detailed quotes from potential suppliers like Met3dp that break down costs and provide realistic lead time estimates based on your specific design files and requirements. Providing comprehensive information (CAD files, material specs, tolerance requirements, quantity) will enable faster and more accurate quoting.
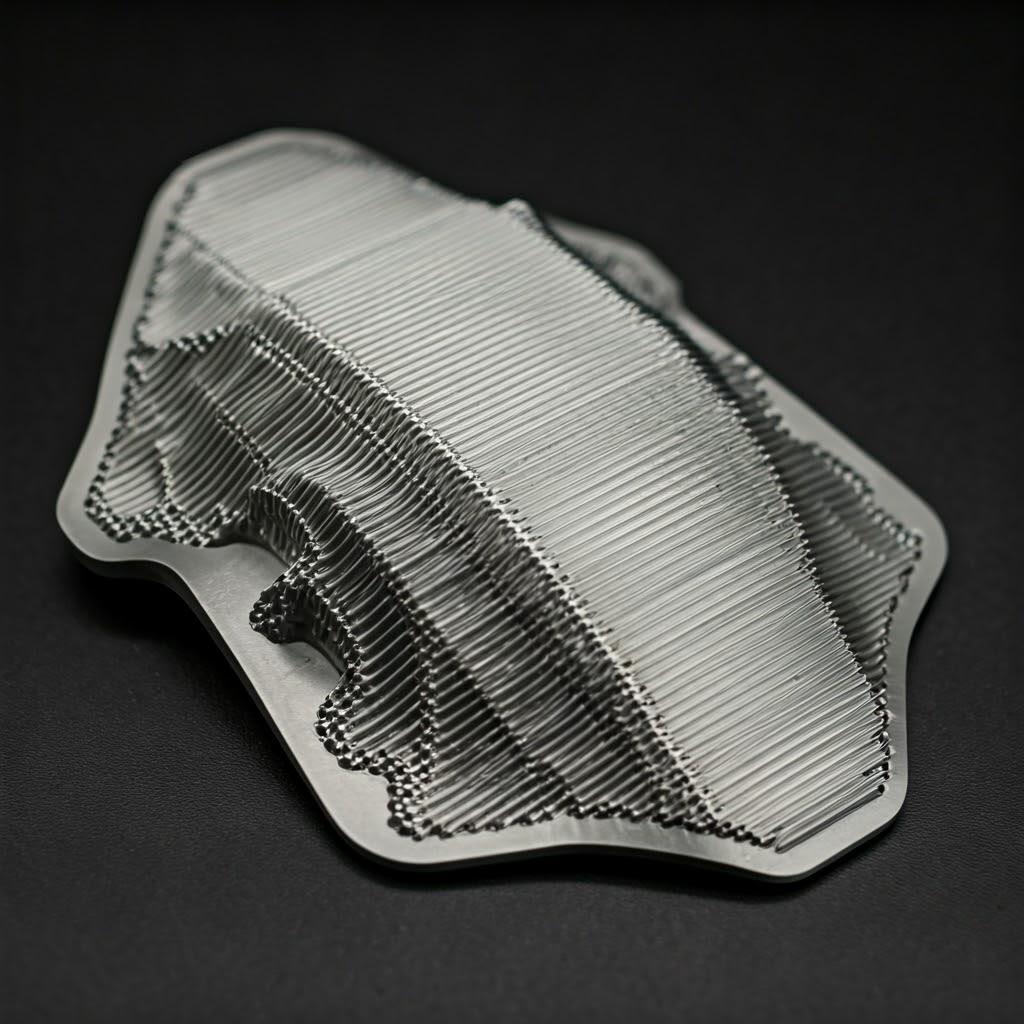
Frequently Asked Questions (FAQ) about Metal 3D Printed Engine Heat Shields
Here are answers to some common questions regarding the use of metal additive manufacturing for engine heat shields:
- Q1: What is the maximum temperature a 3D printed metal heat shield can withstand?
- A: This depends entirely on the material chosen. AlSi10Mg is suitable for lower temperatures (up to ~200°C). Ti-6Al-4V performs well up to ~400°C. For extreme heat near exhaust manifolds or turbochargers, nickel superalloys like IN625 are required, capable of handling temperatures exceeding 900°C. The design (air gaps, coatings) also plays a role.
- Q2: How much weight can be saved using a 3D printed heat shield compared to a traditional one?
- A: Significant weight savings are possible, especially when using topology optimization and materials like AlSi10Mg or Ti-6Al-4V. Savings of 30-60% or even more compared to traditionally designed and manufactured steel or even aluminum shields are achievable, depending on the original design and optimization level. This is a major advantage in aerospace and performance automotive applications.
- Q3: Is metal 3D printing suitable for both prototype and series production of heat shields?
- A: Absolutely. Metal AM is ideal for rapid prototyping due to the elimination of tooling costs and speed. It is also increasingly viable for low-to-medium volume series production, particularly for complex, high-value, or custom heat shields where the benefits of design freedom, weight reduction, and performance optimization outweigh potentially higher per-part costs compared to mass-produced stamped parts. For very high volumes of simple designs, traditional methods may still be more economical.
Conclusion: Embrace the Future of Thermal Management with Met3dp
Engine heat shields are indispensable components, critical for the safety, reliability, and performance of systems across the automotive, aerospace, and industrial sectors. While traditional manufacturing methods have served their purpose, they often fall short in meeting the demands for increased complexity, customization, lightweighting, and rapid development cycles characteristic of modern engineering.
Metal additive manufacturing has emerged as a transformative technology, offering unparalleled design freedom to create highly optimized, complex, and lightweight heat shields tailored to specific thermal challenges. By leveraging materials like versatile AlSi10Mg, high-strength Ti-6Al-4V, and extreme-temperature resistant IN625, metal AM enables the production of heat shields with superior performance characteristics. The ability to rapidly prototype, eliminate tooling costs, and consolidate assemblies further enhances its value proposition.
Successfully implementing metal AM for heat shields requires careful consideration of design principles (DfAM), material properties, achievable tolerances, post-processing necessities, and potential challenges. Partnering with a knowledgeable and capable supplier is paramount. Met3dp, with its vertically integrated capabilities spanning advanced metal powder production using tecnologías de atomización de gas y PREP, industry-leading 3D printing systems, and comprehensive application support, stands ready to assist engineers and procurement managers.
By embracing impresión 3D en metal, companies can revolutionize their approach to engine thermal management, achieving levels of performance, customization, and efficiency previously unattainable. Contact Met3dp today to explore how their cutting-edge systems, high-quality materials, and deep expertise can power your next-generation heat shield solutions and accelerate your organization’s additive manufacturing goals.
Compartir
Facebook
Twitter
LinkedIn
WhatsApp
Correo electrónico
MET3DP Technology Co., LTD es un proveedor líder de soluciones de fabricación aditiva con sede en Qingdao, China. Nuestra empresa está especializada en equipos de impresión 3D y polvos metálicos de alto rendimiento para aplicaciones industriales.
Solicite información para obtener el mejor precio y una solución personalizada para su empresa.
Artículos relacionados
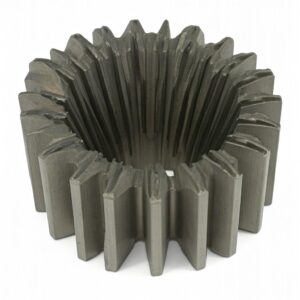
Segmentos de álabe de tobera de alto rendimiento: Revolucionando la eficiencia de las turbinas con la impresión metálica en 3D
Leer Más "
13 de mayo de 2025
No hay comentarios
Acerca de Met3DP
Actualización reciente
Nuestro producto
CONTACTO
¿Tiene alguna pregunta? ¡Envíenos un mensaje ahora! Atenderemos su solicitud con todo un equipo tras recibir su mensaje.
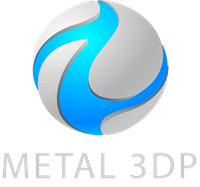
Polvos metálicos para impresión 3D y fabricación aditiva
PRODUCTO
cONTACT INFO
- Ciudad de Qingdao, Shandong, China
- [email protected]
- [email protected]
- +86 19116340731