Additive Manufactured Cooling Jackets for Electric Motors
Índice
Introduction: The Critical Role of Thermal Management in High-Performance Electric Motors
Electric motors are the workhorses of modern industry, powering everything from electric vehicles (EVs) and industrial robots to critical aerospace systems and precision medical devices. As engineers push the boundaries of power density and performance, managing the heat generated during operation becomes paramount. Inefficient heat dissipation leads to reduced efficiency, component degradation, shortened operational lifespan, and even catastrophic failure. This makes effective thermal management not just a desirable feature, but an absolute necessity for reliability and performance, especially in demanding B2B applications where downtime translates directly to lost revenue.
Enter the electric motor cooling jacket. This critical component acts as a heat exchanger, typically enveloping the motor housing (stator) or integrated directly into its structure. It utilizes a circulating coolant (like water-glycol mixtures or specialized dielectric fluids) to absorb excess heat from the motor’s core components (windings, rotor, bearings) and transfer it away to a radiator or other heat dissipation system. A well-designed cooling jacket is essential for:
- Maintaining Optimal Operating Temperatures: Preventing motors from exceeding their critical temperature limits.
- Enhancing Efficiency: Motors operate more efficiently within their designed temperature range.
- Increasing Power Density: Enabling smaller, lighter motors to deliver higher power outputs without overheating.
- Extending Motor Lifespan: Reducing thermal stress on insulation, bearings, and other sensitive components.
- Improving Reliability: Minimizing the risk of failures caused by excessive heat buildup in industrial and mission-critical systems.
Traditionally, cooling jackets have been manufactured using methods like casting, extrusion, fabrication (welding/brazing multiple parts), or subtractive machining from billet material. While effective to a degree, these conventional approaches often face limitations, particularly when dealing with complex internal geometries needed for highly efficient heat transfer or when customization and rapid iteration are required. Design constraints inherent in casting (minimum wall thickness, draft angles) or machining (tool access limitations) can restrict the optimization of coolant flow paths and surface area contact. Fabricated assemblies introduce potential leak points and add manufacturing complexity.
Aquí es donde fabricación aditiva (AM) de metales, often referred to as metal Impresión 3D, emerges as a transformative technology. By building parts layer by layer directly from digital designs using high-performance metal powders, AM unlocks unprecedented design freedom. This allows engineers to create highly complex, topology-optimized cooling jackets with intricate internal channels that conform precisely to the motor’s heat sources. The result is significantly improved thermal performance, reduced weight, consolidated parts, and the ability to produce custom designs rapidly – advantages highly sought after by procurement managers and engineering teams in the fabricación de automóviles, ingeniería aeroespacialy industrial automation sectors.
Companies seeking a competitive edge are increasingly turning to specialized AM service providers y proveedores de polvo de metal to leverage this technology. The ability to produce high-performance, custom cooling jackets on demand aligns perfectly with the needs of modern manufacturing, offering solutions that were previously impossible or prohibitively expensive. This blog post will delve into the specifics of using metal AM for electric motor cooling jackets, exploring applications, benefits, recommended materials like AlSi10Mg and CuCrZr, design considerations, and how to partner with the right B2B supplier for your thermal management needs.
Applications & Industries: Where are AM Cooling Jackets Deployed?
The advantages of additive manufacturing for thermal management components, particularly cooling jackets, resonate across a diverse range of industries where electric motor performance and reliability are critical. Procurement professionals and engineers sourcing components for high-stakes applications are increasingly specifying AM solutions to meet demanding thermal challenges. Key sectors include:
- Automotive (Especially Electric Vehicles – EVs):
- Traction Motors: EVs rely on powerful electric motors operating under highly variable loads. Efficient cooling is vital for maximizing range, performance (acceleration, top speed), and battery life. AM allows for compact, lightweight cooling jackets with highly optimized internal channels conforming to stator and rotor shapes, crucial for packaging constraints in vehicles.
- Power Electronics Cooling: Inverters and converters also generate significant heat. AM can create integrated cooling solutions or dedicated cold plates with complex geometries.
- Motorsport: Racing applications demand maximum performance and minimal weight. Custom AM cooling jackets provide a competitive edge through superior heat dissipation in extreme operating conditions. B2B suppliers focused on automotive manufacturing solutions find significant demand here.
- Aeroespacial y defensa:
- Actuation Systems: Electric motors drive flight control surfaces, landing gear, and other critical systems. Reliability and weight savings are paramount. AM enables topology-optimized, lightweight cooling jackets that meet stringent aerospace requirements (e.g., AS9100 certification often required from the AM provider).
- Auxiliary Power Units (APUs) & Generators: Ensuring thermal stability for power generation systems on aircraft.
- UAVs/Drones: Weight is a critical factor. Lightweight AM cooling solutions help extend flight times and payload capacity. Aerospace procurement specialists look for AM partners with proven experience and material traceability.
- Industrial Automation & Robotics:
- High-Precision Servo Motors: Robots and CNC machines utilize motors requiring precise control and high duty cycles. Consistent thermal performance ensures accuracy and prevents overheating during continuous operation. AM allows for integrated cooling channels directly into motor housings or highly efficient external jackets.
- Heavy-Duty Industrial Motors: Cooling jackets for large motors used in manufacturing plants, processing facilities, and resource extraction improve reliability and reduce maintenance downtime – a key concern for industrial procurement departments.
- Custom Machinery: OEMs building specialized industrial equipment can leverage AM for bespoke cooling solutions tailored to unique motor designs and operating environments.
- Productos sanitarios:
- Surgical Robots & Equipment: Motors in medical robotics require high precision and reliability. Effective thermal management prevents performance degradation during procedures.
- Imaging Systems (MRI/CT): Cooling components for motors within gantries or positioning systems. Biocompatibility might be a factor depending on the specific application, influencing material choice and post-processing.
- Sector energético:
- Downhole Drilling Motors: Operating in high-temperature, high-pressure environments demands robust thermal management.
- Pump & Compressor Drives: Ensuring reliability in critical infrastructure applications.
Why the Demand from B2B Buyers?
Procurement managers and engineers in these sectors are drawn to AM cooling jackets for several key reasons:
- Performance Improvement: Demonstrable gains in heat dissipation compared to conventional designs.
- Reducción de peso: Critical for aerospace, automotive, and robotics.
- Consolidación de piezas: Reducing assembly complexity, potential leak points, and part count.
- Customization & Rapid Prototyping: Ability to quickly iterate designs and produce tailored solutions for specific motor requirements without the high tooling costs associated with casting.
- Resiliencia de la cadena de suministro: On-demand production capabilities can shorten lead times and reduce reliance on complex traditional supply chains, a factor increasingly valued by compradores mayoristas y distribuidores seeking reliable component sourcing.
Partnering with an experienced impresión 3D en metal service provider is key to realizing these benefits across diverse industrial applications.
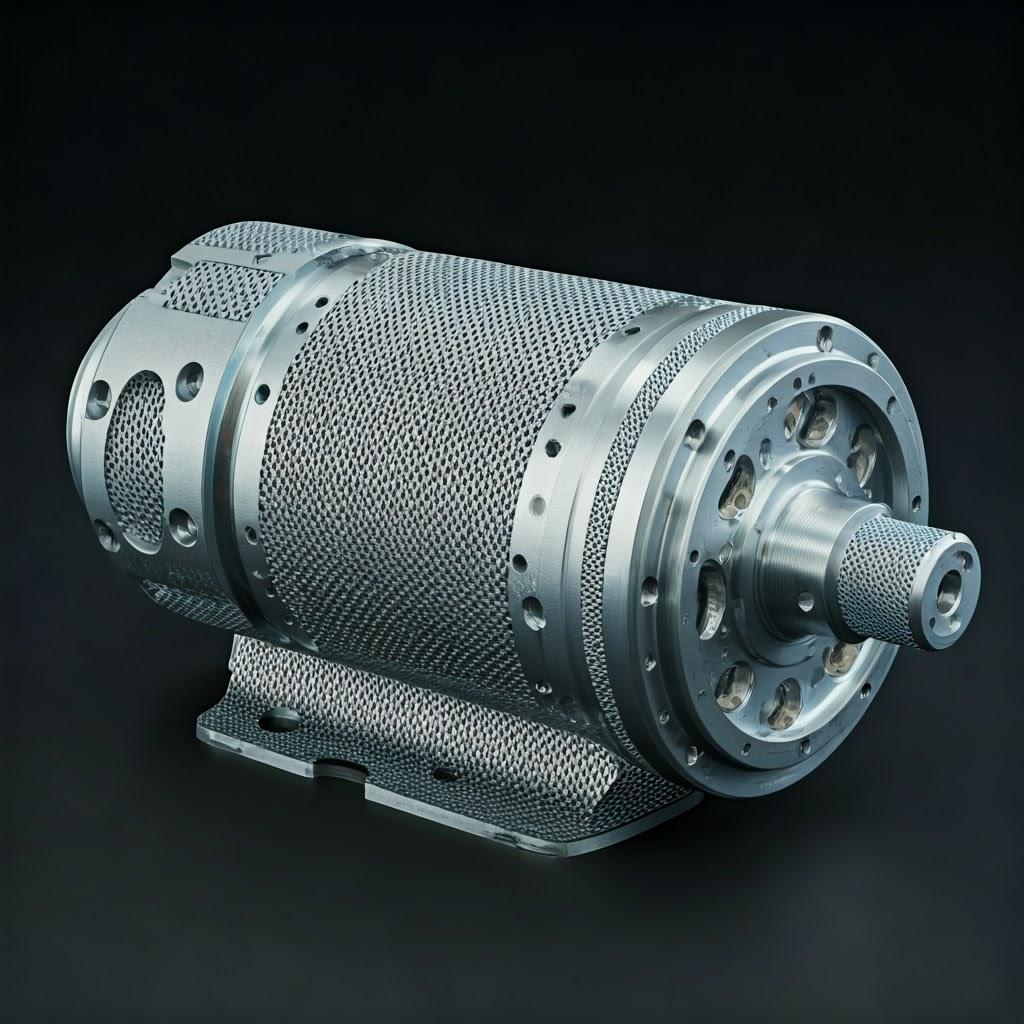
Why Metal Additive Manufacturing for Cooling Jackets? Unlocking Performance Gains
While traditional manufacturing methods have served the industry for decades, metal additive manufacturing offers compelling advantages that directly address the limitations of casting, machining, and fabrication, particularly for complex thermal management components like electric motor cooling jackets. For engineers striving for optimal performance and procurement managers seeking value and reliability, AM presents a paradigm shift.
Comparison: Metal AM vs. Traditional Methods for Cooling Jackets
Característica | Metal Additive Manufacturing (LPBF/SLM) | Fundición tradicional | Traditional Machining (Subtractive) | Traditional Fabrication (Welding/Brazing) |
---|---|---|---|---|
Complejidad del diseño | Very High: Enables intricate internal channels, thin walls, organic shapes, lattice structures, topology optimization. | Moderate: Limited by draft angles, wall thickness constraints, tooling complexity. | Low to Moderate: Limited by tool access, feature complexity drives cost significantly. | Low: Typically simpler geometries joined. |
Internal Channels | Highly Optimized: Conformal channels following heat sources, complex non-linear paths possible. | Simple: Typically straight or gently curved passages, limited complexity. | Very Limited: Primarily straight drilled/milled channels. Deep/complex channels difficult. | Simple: Tubes or plates welded together. |
Espesor de pared | Very Thin: Can achieve sub-millimeter walls (design dependent). | Limited: Minimum wall thickness required for metal flow. | Flexible: But thin features can be prone to vibration/chatter. | Depends on Sheet/Tube Stock: Can be thin. |
Consolidación de piezas | Excellent: Multiple components can be integrated into a single printed part. | Good: Can produce complex single pieces. | Poor: Typically starts with a single block. | Poor: Involves joining multiple parts. |
Reducción de peso | Excellent: Topology optimization and lattice structures significantly reduce weight. | Fair: Can be heavy due to minimum thickness constraints. | Fair: Material removal is inefficient for complex lightweighting. | Fair: Depends on design and materials used. |
Opciones de material | Growing Range: Includes Aluminum alloys (AlSi10Mg), Copper alloys (CuCrZr), Stainless Steels, Nickel alloys, Titanium. | Wide Range: Established casting alloys. | Wide Range: Any machinable stock. | Wide Range: Weldable/brazeable alloys. |
Coste de utillaje | None: Direct digital manufacturing. | High: Molds and patterns required. | Low/None: Standard tooling, but fixtures may be needed. | Low/Moderate: Fixtures often required. |
Lead Time (Prototype) | Fast: Days to weeks. | Slow: Weeks to months (tooling dependent). | Moderate: Days to weeks. | Moderate: Days to weeks. |
Lead Time (Production) | Scalable: Can be competitive depending on volume/complexity. | Fast (High Volume): Once tooling exists. | Slow (Complex Parts): Can be time-consuming. | Moderate: Depends on complexity/automation. |
Personalización | Excellent: Easy to modify designs without tooling changes. | Poor: Requires expensive tooling modification. | Good: Relatively easy to change programs. | Fair: Modifications may require fixture changes. |
Potential Leak Points | Minimized: Single-piece construction possible. | Low: Generally monolithic. | N/A (Monolithic): If machined from solid. | High: Welds/brazes are potential failure points. |
Export to Sheets
Key Advantages of AM for Cooling Jackets:
- Unparalleled Geometric Freedom: AM’s layer-by-layer approach liberates designers from the constraints of traditional manufacturing. This allows for:
- Canales de refrigeración conformados: Paths that precisely follow the contours of the motor’s hottest areas (e.g., stator windings), maximizing heat transfer efficiency.
- Complex Internal Networks: Designing intricate lattices, fins, or turbulence-promoting features within channels to enhance heat exchange with the coolant, something impossible with drilling or casting.
- Topology Optimization: Using software to computationally design the most efficient structure based on load paths and thermal requirements, often resulting in organic-looking, lightweight, yet strong designs.
- Enhanced Thermal Performance: The ability to place cooling channels exactly where needed and maximize the surface area for heat exchange leads to significantly better thermal performance compared to jackets with simpler, less targeted cooling paths. This translates directly to lower motor operating temperatures, higher efficiency, and increased power density.
- Consolidación de piezas: What might traditionally require multiple cast, machined, and welded components (e.g., jacket housing, inlet/outlet fittings, internal baffles) can often be consolidated into a single, monolithic part produced via AM. This drastically reduces assembly time, eliminates potential leak points at joints/welds, simplifies inventory management for Compradores B2B, and improves overall system reliability.
- Creación rápida de prototipos e iteración: AM allows engineers to move from a digital design to a physical metal prototype in a matter of days. This accelerates the development cycle, enabling quick testing and validation of different cooling strategies without incurring the high costs and long lead times associated with traditional tooling. Multiple design variants can be tested concurrently.
- La personalización en masa: Producing tailored cooling jacket designs for specific motor variants or applications is economically feasible with AM, as no physical tooling needs to be changed. This is ideal for OEMs producing specialized equipment or for the aftermarket and replacement parts sector.
- Reducción de peso: Through topology optimization and the use of internal lattice structures where appropriate, AM can create cooling jackets that are significantly lighter than their traditionally manufactured counterparts while maintaining structural integrity and thermal performance. This is a critical advantage in weight-sensitive applications like aerospace and automotive EVs.
While AM offers these significant benefits, it’s important to partner with a knowledgeable service provider who understands the nuances of the process, material science, and post-processing required to deliver high-quality, reliable cooling jackets ready for demanding industrial use.
Material Focus: AlSi10Mg and CuCrZr for Optimal Thermal Performance
Selecting the right material is fundamental to the performance of an additively manufactured electric motor cooling jacket. The primary function is heat transfer, making thermal conductivity a key property. However, mechanical strength, weight, corrosion resistance, printability, and cost are also crucial considerations for engineers and procurement specialists. Two materials stand out for this application in metal AM: AlSi10Mg (an aluminum-silicon-magnesium alloy) and CuCrZr (a copper-chromium-zirconium alloy).
Met3dp, leveraging its expertise in advanced powder manufacturing systems including industry-leading gas atomization and Plasma Rotating Electrode Process (PREP) technologies, produces high-quality, spherical metal powders ideal for AM processes like Selective Laser Melting (SLM) / Laser Powder Bed Fusion (LPBF). Our focus on powder characteristics like high sphericity and good flowability ensures optimal processing and results in dense, high-performance printed parts. We offer both AlSi10Mg and CuCrZr powders optimized for additive manufacturing.
AlSi10Mg (Aluminum Alloy): The Lightweight All-Rounder
AlSi10Mg is one of the most common and well-understood aluminum alloys used in metal AM. It’s essentially a casting alloy adapted for powder bed fusion processes.
- Key Properties & Benefits:
- Good Thermal Conductivity: While not as high as pure aluminum or copper alloys, its thermal conductivity (typically 120-150 W/m·K after appropriate heat treatment) is sufficient for many motor cooling applications.
- Excelente relación resistencia-peso: Aluminum alloys are significantly lighter than copper or steel, making AlSi10Mg ideal for weight-sensitive applications (automotive, aerospace).
- Buena imprimibilidad: It processes relatively easily in LPBF systems, allowing for fine features and complex geometries.
- Buena resistencia a la corrosión: Exhibits good resistance to corrosion in typical coolant environments (e.g., water-glycol mixtures).
- Rentabilidad: Generally more affordable than copper alloys.
- Post-procesamiento: Can be readily machined, polished, and heat-treated (e.g., T6 heat treatment to improve strength and ductility).
- Consideraciones:
- Lower absolute thermal conductivity compared to copper alloys.
- Requires appropriate heat treatment post-printing to achieve optimal mechanical properties and thermal conductivity.
- Aplicaciones típicas: EV motor cooling jackets, aerospace components, industrial motors where weight is a significant factor, heat exchangers requiring complex shapes.
CuCrZr (Copper Alloy): The High-Conductivity Champion
CuCrZr is a high-performance copper alloy specifically designed for applications requiring excellent thermal and electrical conductivity combined with good mechanical strength, especially at elevated temperatures.
- Key Properties & Benefits:
- Excelente conductividad térmica: Significantly higher than aluminum alloys (typically >300 W/m·K), making it extremely efficient at transferring heat. Ideal for very high-power density motors or applications with extreme thermal loads.
- Good High-Temperature Strength: Retains mechanical properties better than pure copper at elevated temperatures.
- Good Wear Resistance: Offers better wear characteristics than pure copper.
- Imprimibilidad: While more challenging to print than AlSi10Mg due to copper’s high reflectivity and thermal conductivity (requiring higher laser power and specific process parameters), specialized AM systems and optimized powders, like those from Met3dp, enable successful printing.
- Consideraciones:
- Higher Density: Significantly heavier than aluminum alloys (approx. 8.9 g/cm³ vs 2.67 g/cm³).
- Mayor coste de los materiales: Copper alloys are generally more expensive than aluminum alloys.
- More Challenging Printing: Requires optimized process parameters and potentially specific machine configurations (e.g., green or blue lasers can be advantageous). Surface finish might be rougher in the as-built state compared to AlSi10Mg.
- Aplicaciones típicas: High-performance motorsport motor cooling, cooling components for power electronics, heat sinks requiring maximum heat dissipation, induction coils, resistance welding electrodes (though cooling jackets are the focus here).
Material Selection Guide:
Propiedad | AlSi10Mg | CuCrZr | Key Consideration for Cooling Jackets |
---|---|---|---|
Conductividad térmica | Good (120-150 W/m·K post-HT) | Excellent (>300 W/m·K) | Primary function; CuCrZr offers ~2x performance but may be overkill. |
Densidad | Low (~2.67 g/cm³) | High (~8.9 g/cm³) | Weight savings critical in automotive/aerospace; favor AlSi10Mg. |
Resistencia mecánica | Good (especially post-HT) | Good (retains strength at temp) | Must withstand operating pressures and vibrations. |
Imprimibilidad | Excelente | Moderate (requires optimization) | Affects achievable feature resolution, surface finish, cost. |
Resistencia a la corrosión | Bien | Bien | Important for longevity with specific coolants. |
Coste | Baja | Más alto | Significant factor for B2B procurement and volume production. |
El más adecuado para | Balanced performance, weight-sensitive applications, cost-conscious projects. | Maximum thermal performance, high-power density applications where weight is secondary. | Match material to specific performance requirements and budget. |
Export to Sheets
Met3dp’s Role: As a provider of both advanced polvos metálicos and AM solutions, Met3dp can assist customers in selecting the optimal material for their cooling jacket application. Our high-quality AlSi10Mg and CuCrZr powders, produced using state-of-the-art atomization techniques, ensure the best possible starting point for achieving high-performance, reliable additively manufactured components. We understand the specific processing requirements for each material, ensuring successful prints and optimized end-part properties.
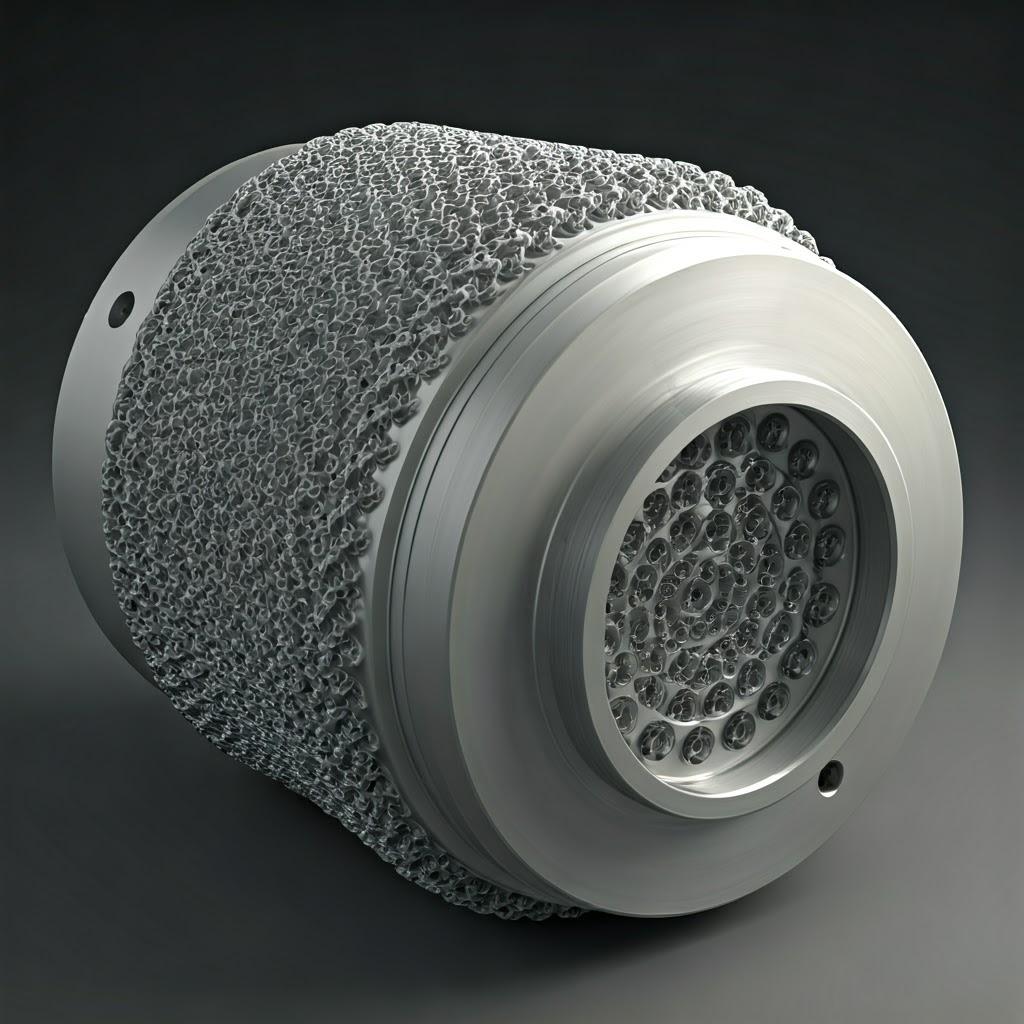
Design for Additive Manufacturing (DfAM): Optimizing Cooling Jacket Geometry
Simply replicating a traditionally designed cooling jacket using additive manufacturing often fails to capitalize on the technology’s true potential. To unlock the significant performance, weight, and consolidation benefits discussed earlier, engineers must embrace Diseño para fabricación aditiva (DfAM) principles. DfAM is not just about making a part printable; it’s about fundamentally rethinking the design to leverage AM’s unique capabilities, particularly the freedom to create complex internal and external geometries. For components like electric motor cooling jackets, where fluid flow and heat transfer are paramount, DfAM is critical.
Key DfAM Principles for AM Cooling Jackets:
- Embrace Geometric Complexity for Function:
- Conformal Channels: Design coolant channels that precisely follow the shape of the heat source (e.g., stator laminations or housing curves). This minimizes the thermal path and maximizes contact area, drastically improving heat transfer efficiency compared to straight, drilled channels common in traditional designs.
- Internal Flow Optimizers: Integrate features within the channels to enhance thermal performance. This can include:
- Turbulators: Small ribs or bumps that intentionally disrupt laminar flow, promoting turbulence which increases the heat transfer coefficient between the coolant and the channel wall.
- Internal Fins/Pin Arrays: Structures that increase the internal surface area available for heat exchange within the coolant passage.
- Variable Channel Cross-Sections: Optimizing flow velocity and pressure drop throughout the cooling circuit.
- Optimized Inlets/Outlets: Design smooth, efficient transitions into and out of the cooling jacket to minimize pressure losses and ensure even flow distribution. Consider integrating standard fitting interfaces directly into the AM design.
- Leverage Topology Optimization & Generative Design:
- Use specialized software tools to computationally determine the most efficient material distribution for the cooling jacket based on defined load cases (structural and thermal), boundary conditions (mounting points, fluid connections), and performance targets (maximum temperature, minimum weight).
- This often results in organic, “bionic” structures that remove material from low-stress areas while reinforcing critical load paths, leading to significant weight savings without compromising strength or thermal function. This is invaluable for automotive and aerospace procurement where weight reduction is a primary driver.
- Design for Self-Support & Minimize Support Structures:
- Overhangs and Angles: Understand the self-supporting angle limitations of the chosen material (AlSi10Mg, CuCrZr) and AM process (LPBF). Typically, angles greater than 45 degrees from the horizontal can be built without support structures. Design features like internal channels with teardrop or diamond cross-sections instead of purely circular or horizontal rectangular ones to minimize the need for internal supports, which can be very difficult or impossible to remove.
- Orientación de construcción: Consider the optimal build orientation early in the design phase. This affects surface finish on different faces, the location and amount of required support structures, residual stress accumulation, and potentially print time and cost. Orienting critical surfaces or complex channel networks strategically can simplify printing and post-processing.
- Sacrificial Features: Sometimes, small features might be added specifically to support a more critical section during the build, designed to be easily machined away later.
- Consolidate Parts:
- Actively look for opportunities to combine multiple components (e.g., housing, fittings, brackets, internal baffles) into a single, monolithic printed part. This reduces assembly labor, part count, inventory complexity for B2B suppliers and distributors, and eliminates potential leak paths associated with joints, welds, or seals.
- Optimize Wall Thickness & Feature Resolution:
- AM allows for very thin walls (down to ~0.4-0.5 mm, depending on geometry and process), enabling lightweight designs. However, ensure walls are thick enough to withstand operating pressures, handle potential vibrations, and allow for effective heat transfer without excessive thermal resistance.
- Be mindful of the minimum feature size printable by the specific LPBF system (~0.2-0.4 mm). Avoid features smaller than this resolution limit.
- Design for Post-Processing:
- Machining Allowances: If certain surfaces require high precision or specific finishes (e.g., sealing faces, mounting interfaces, threaded holes), add extra stock material (machining allowance) in the AM design for subsequent CNC machining.
- Powder Removal: Design internal channels with smooth bends and avoid sharp corners or dead ends where powder can become trapped and difficult to remove post-print. Consider adding access ports for cleaning if necessary, which can be plugged later.
- Support Access: Ensure support structures are accessible for removal, especially crucial for complex internal geometries.
Collaboration with AM Experts:
Successfully applying DfAM principles often requires collaboration with experienced AM service providers like Met3dp. Our engineers understand the nuances of various metal 3D printing methods and materials (AlSi10Mg, CuCrZr) and can provide valuable input during the design phase to ensure manufacturability, optimize performance, and minimize cost. Leveraging this expertise early can prevent costly redesigns and accelerate time-to-market for high-performance cooling solutions.
Achievable Precision: Tolerance, Surface Finish, and Dimensional Accuracy in AM Cooling Jackets
While AM offers incredible geometric freedom, understanding the achievable levels of precision is crucial for engineers designing functional components like cooling jackets, especially for integration into larger assemblies. Procurement managers also need assurance regarding the consistency and quality of parts delivered by industrial AM suppliers. Key aspects include dimensional tolerances, surface finish (roughness), and overall accuracy.
Dimensional Tolerances:
- Typical LPBF Tolerances: For well-controlled Laser Powder Bed Fusion (LPBF) processes using materials like AlSi10Mg or CuCrZr, typical achievable dimensional tolerances for smaller parts (e.g., < 100 mm) are often in the range of ±0.1 mm to ±0.2 mm (±0.004″ to ±0.008″). For larger dimensions, a tolerance of ±0.1% to ±0.2% of the nominal dimension is a common guideline.
- Factors Influencing Tolerance:
- Part Size and Geometry: Larger parts or those with complex features may exhibit greater deviation due to accumulated thermal stress and shrinkage.
- Material: Different materials shrink and warp differently during printing and cooling.
- Orientación de construcción: The orientation on the build plate affects accuracy, particularly in the Z-direction (build height).
- Gestión térmica: Machine stability and process control (laser parameters, gas flow) are critical.
- Support Strategy: How the part is supported influences stability during the build.
- Post-procesamiento: Stress relief heat treatments can cause minor dimensional changes. Machining operations achieve much tighter tolerances on specific features.
- Achieving Tighter Tolerances: Where tighter tolerances are required (e.g., mating surfaces, bearing interfaces, sealing grooves), it’s standard practice to design the AM part with additional material (machining stock) and use post-process CNC machining to achieve tolerances down to ±0.01 mm to ±0.05 mm (±0.0004″ to ±0.002″) or even better, comparable to conventional machining.
Surface Finish (Roughness):
- As-Built Surface Roughness: The surface finish of parts directly off the AM machine is inherently rougher than machined surfaces. This is due to the layer-by-layer process and the partially melted powder particles adhering to the surface.
- Typical Ra Values: As-built surface roughness (Ra – arithmetic average roughness) for LPBF typically ranges from 8 µm to 20 µm (315 µin to 787 µin), depending heavily on:
- Orientation: Upward-facing surfaces and vertical walls tend to be smoother than downward-facing surfaces (which show layer stepping) and surfaces relying on supports (which leave witness marks upon removal).
- Material: Finer powders and optimized parameters can lead to smoother finishes.
- Process Parameters: Laser power, speed, layer thickness all play a role.
- Typical Ra Values: As-built surface roughness (Ra – arithmetic average roughness) for LPBF typically ranges from 8 µm to 20 µm (315 µin to 787 µin), depending heavily on:
- Internal Channels: The roughness of internal channels is critical for fluid dynamics (pressure drop) and heat transfer. As-built internal surfaces will have similar roughness values. This inherent roughness can sometimes be beneficial, promoting turbulence and enhancing heat transfer, but excessive roughness increases pressure drop.
- Mejora del acabado superficial: For applications requiring smoother surfaces (e.g., sealing faces, reducing friction, aesthetics), various post-processing techniques are employed:
- CNC Machining: Provides the smoothest, most controlled finish on accessible surfaces.
- Abrasive Flow Machining (AFM) or Extrude Hone: Pushing an abrasive putty through internal channels to smooth them.
- Chemical Polishing: Using chemical etchants to smooth surfaces (material dependent).
- Tumbling/Vibratory Finishing: Using media to smooth external surfaces of batches of parts.
- Micro-Machining/Polishing: Manual or automated polishing for specific areas.
Dimensional Accuracy & Quality Control:
- Ensuring the final cooling jacket meets all dimensional and surface specifications is critical. Reputable B2B additive manufacturing providers employ rigorous quality control measures:
- Process Monitoring: In-situ monitoring during the build (e.g., melt pool monitoring) can help detect anomalies.
- Coordinate Measuring Machines (CMM): Used for precise dimensional inspection of critical features post-print or post-machining.
- 3D Scanning: Comparing the final part geometry against the original CAD model to verify overall accuracy and identify deviations.
- Medición de la rugosidad superficial: Using profilometers to quantify surface finish.
- CT Scanning: Can be used for non-destructive inspection of internal channels and detection of internal defects like porosity.
Understanding these precision aspects allows engineers to design effectively, specifying tighter tolerances only where functionally necessary (often relying on post-machining) and accepting as-built finishes where appropriate, thereby optimizing cost and lead time.
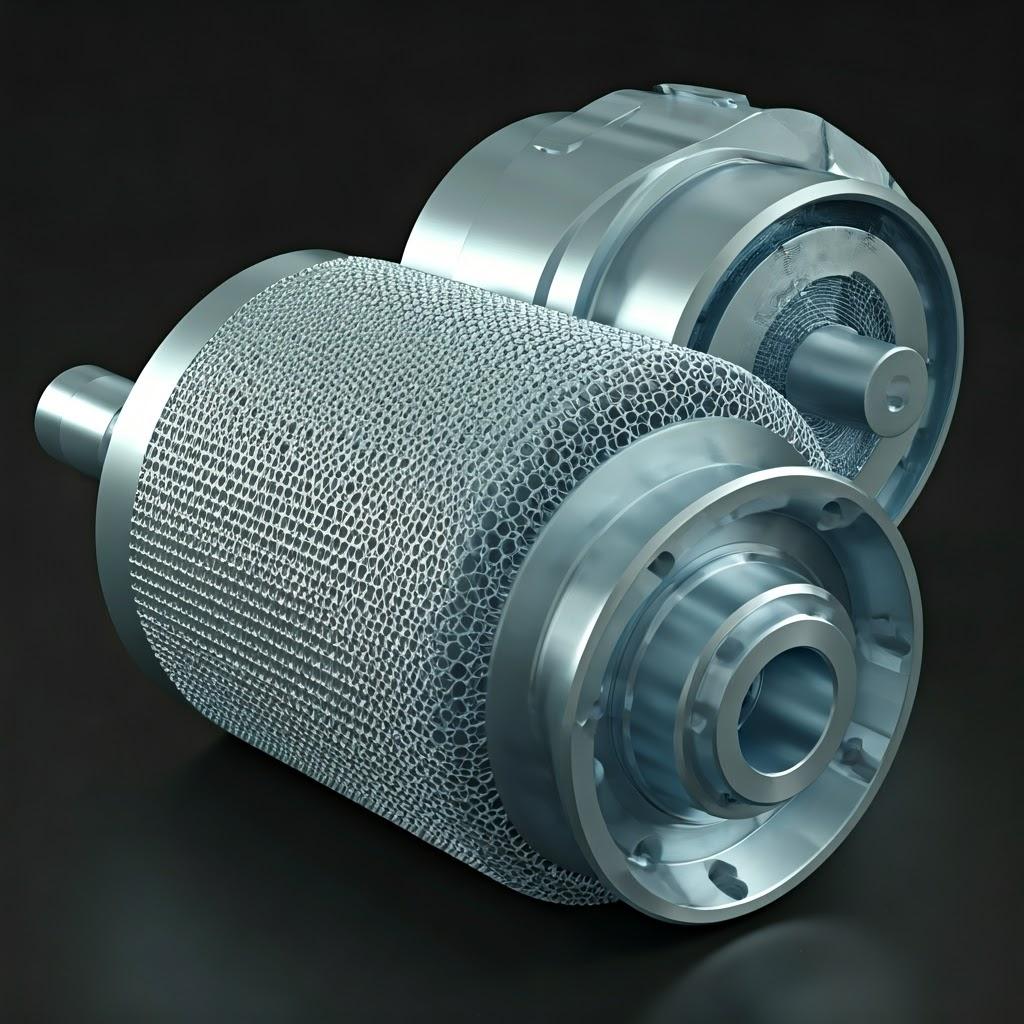
Post-Processing Pathways: From Printed Part to Finished Component
An additively manufactured metal part, as it comes off the printer, is rarely ready for end-use, especially for demanding applications like electric motor cooling jackets. A series of post-processing steps are typically required to achieve the desired material properties, dimensional accuracy, surface finish, and overall functionality. The specific pathway depends on the material (AlSi10Mg or CuCrZr), design complexity, and application requirements.
Common Post-Processing Steps for AM Cooling Jackets:
- Stress Relief / Heat Treatment:
- Propósito: The rapid heating and cooling cycles inherent in LPBF create significant internal stresses within the printed part. A stress relief heat treatment (typically performed while the part is still attached to the build plate) is crucial to reduce these stresses, preventing distortion or cracking during subsequent steps (like removal from the plate) and improving dimensional stability.
- Proceso: Involves heating the part in a controlled atmosphere furnace to a specific temperature (below the aging or solutionizing temperature), holding it for a set duration, and then cooling slowly.
- Further Heat Treatments (e.g., T6 for AlSi10Mg): Additional heat treatments may be required to achieve desired final mechanical properties (strength, ductility, hardness) and optimize thermal conductivity. For AlSi10Mg, a T6 cycle (solution treatment followed by artificial aging) is common. CuCrZr may also undergo specific aging treatments to optimize its strength and conductivity. Parameters must be carefully controlled based on the alloy specification.
- Extracción de la placa de construcción:
- Métodos: Parts are typically separated from the build plate using wire EDM (Electrical Discharge Machining) or band sawing. Care must be taken to avoid damaging the part.
- Retirada de la estructura de soporte:
- Propósito: Support structures are necessary during the build to anchor the part and support overhangs. These must be removed carefully.
- Métodos: Supports are often removed manually using hand tools (pliers, cutters) or machined away (milling, grinding). Access can be challenging, especially for internal supports within complex channel networks (highlighting the importance of DfAM to minimize internal supports). Residual witness marks where supports were attached are common and may require further finishing.
- Powder Removal / Cleaning:
- Propósito: Ensuring all loose or partially sintered powder is removed from the part, especially within intricate internal cooling channels, is critical for preventing contamination of the cooling system and ensuring unobstructed flow.
- Métodos: Compressed air blasting, bead blasting, ultrasonic cleaning baths, and specialized flushing systems are used. Designing for powder removal (avoiding dead ends, sharp internal corners) is crucial.
- CNC Machining:
- Propósito: To achieve tight tolerances, specific surface finishes, and features that cannot be accurately produced by AM alone.
- Aplicaciones: Machining mating surfaces for tight seals, creating precise O-ring grooves, drilling and tapping threaded holes for fittings, facing flanges flat, achieving high-precision diameters for bearing fits. Requires careful fixture design to hold the often complex AM part securely.
- Surface Finishing / Smoothing:
- Propósito: To improve surface roughness beyond the as-built state for functional (sealing, fluid dynamics) or aesthetic reasons.
- Métodos: As discussed previously – tumbling, bead blasting (can provide a uniform matte finish), abrasive flow machining (for internal channels), chemical polishing, electro-polishing, manual polishing. The choice depends on the desired finish level, geometry accessibility, and material.
- Leak Testing:
- Propósito: Absolutely critical for a cooling jacket. Verifies the integrity of the printed structure and ensures no coolant can escape.
- Métodos: Typically involves pressurizing the cooling channels with air or helium while the part is submerged in water (bubble test) or using a helium mass spectrometer leak detector for higher sensitivity. Pressure testing protocols should reflect the intended operating conditions.
- Inspection and Quality Control:
- Propósito: Final verification that the part meets all dimensional, material, and functional requirements before shipment.
- Métodos: CMM, 3D scanning, surface profilometry, visual inspection, material certification review, CT scanning (for internal integrity checks if required).
Workflow Example (Simplified):
Code snippet
graph TD
A[LPBF Printing (AlSi10Mg/CuCrZr)] --> B(Stress Relief on Plate);
B --> C{Remove from Plate};
C --> D(Support Removal);
D --> E(Powder Removal & Cleaning);
E --> F{Heat Treatment (e.g., T6)};
F --> G{CNC Machining (Interfaces/Threads)};
G --> H{Surface Finishing (Optional/Specific Areas)};
H --> I(Leak Testing);
I --> J(Final Inspection & QC);
J --> K(Ship to Customer);
%% Styling
classDef default fill:#f9f,stroke:#333,stroke-width:2px;
Understanding this workflow helps engineers design parts compatible with necessary post-processing and allows procurement managers to factor these steps into cost and lead time estimations when engaging with an AM contract manufacturer.
Navigating Challenges in AM Cooling Jacket Production & Solutions
While metal additive manufacturing offers tremendous advantages for producing high-performance cooling jackets, the process is not without its challenges. Awareness of potential issues and partnering with an experienced provider like Met3dp, who employs robust processes and quality controls, is key to successful outcomes.
Common Challenges and Mitigation Strategies:
- Residual Stress, Warping, and Distortion:
- Desafío: The rapid, localized heating and cooling inherent in LPBF builds up internal stresses. If not managed, these stresses can cause parts to warp during printing, distort after removal from the build plate, or even crack. This is particularly relevant for large flat sections or asymmetric designs common in some jacket styles.
- Solutions:
- Process Simulation: Using software to predict thermal stresses and distortion before printing allows for optimization of build orientation and support structures.
- Optimized Support Strategy: Well-designed supports anchor the part firmly and help dissipate heat.
- Build Plate Heating: Elevated build plate temperatures reduce thermal gradients.
- Optimized Scan Strategies: Using specific laser scanning patterns (e.g., island scanning) can help distribute heat more evenly.
- Effective Stress Relief: Performing a proper stress relief cycle immediately after printing is crucial.
- Support Structure Removal (Especially Internal):
- Desafío: Supports inside complex, narrow cooling channels can be extremely difficult or impossible to remove completely, potentially obstructing flow or breaking loose later.
- Solutions:
- DfAM Focus: Prioritize self-supporting channel designs (e.g., teardrop, diamond shapes) during the design phase. Minimize overhangs requiring internal support.
- Soluble/Breakaway Supports (Less Common in Metal): Research continues, but typically supports are dense metal.
- Design for Access: Ensure channels are large enough and pathways smooth enough for manual tool access or effective abrasive/chemical flow-through methods if supports are unavoidable.
- Careful Planning: Requires meticulous planning and often skilled manual labor or specialized post-processing like AFM.
- Achieving Leak-Proof Integrity:
- Desafío: Ensuring the thin walls and complex geometries of the cooling jacket are fully dense and free from micro-porosity or cracks that could lead to coolant leaks under pressure.
- Solutions:
- Optimized Process Parameters: Using validated parameters (laser power, speed, hatch spacing, layer thickness) specific to the material (AlSi10Mg, CuCrZr) and machine is critical for achieving >99.5% density.
- High-Quality Powder: Using high-sphericity, low-oxygen, contamination-free metal powders, like those produced by Met3dp using advanced atomization, is fundamental. Inconsistent powder leads to inconsistent melt behavior and potential defects. (Link to Met3dp Homepage)
- Robust Quality Control: Implementing rigorous leak testing (pressure testing, helium leak detection) on 100% of parts is essential.
- Potential HIPing (Hot Isostatic Pressing): For critical applications, HIP can be used post-print to close internal porosity, although it adds cost and lead time.
- Complete Powder Removal:
- Desafío: Ensuring all unfused powder is removed from intricate internal channels after printing. Trapped powder can impede flow or contaminate the cooling system.
- Solutions:
- DfAM for Drainage: Design channels with smooth bends, sufficient diameter, and potentially drainage/access holes (that can be plugged later). Avoid powder traps.
- Thorough Cleaning Procedures: Utilizing compressed air, vibration, ultrasonic cleaning, and potentially solvent flushing.
- Inspection: Using borescopes or CT scanning (for critical parts) to verify channel cleanliness.
- Surface Finish and Support Witness Marks:
- Desafío: As-built surface roughness, especially on internal channels, can affect pressure drop and heat transfer coefficients. Marks left after support removal can affect sealing or aesthetics.
- Solutions:
- Optimized Orientation: Position critical surfaces optimally during build setup.
- Targeted Post-Processing: Use appropriate methods (machining, AFM, polishing) specifically on surfaces where finish is critical. Accept as-built finish where it doesn’t impact function to control cost.
- Skilled Support Removal: Careful removal minimizes surface scarring.
- Cost and Lead Time:
- Desafío: Metal AM can sometimes be perceived as more expensive or slower than traditional methods, particularly for very high volumes of simple parts.
- Solutions:
- Focus on Value, Not Just Cost: Emphasize the performance gains, weight savings, and consolidation benefits that justify the investment.
- Optimize Design for AM: Efficient designs minimize material usage and print time.
- Partner with Experienced Suppliers: Efficient workflow, machine utilization, and established post-processing chains at experienced providers like Met3dp help manage costs and lead times effectively for B2B production orders.
- Volume Considerations: Discuss potential cost reductions for larger batch sizes or recurring orders.
By understanding these potential challenges and working with a knowledgeable and capable additive manufacturing partner equipped with high-quality materials and robust processes, engineers and procurement managers can confidently leverage AM to produce superior electric motor cooling jackets.
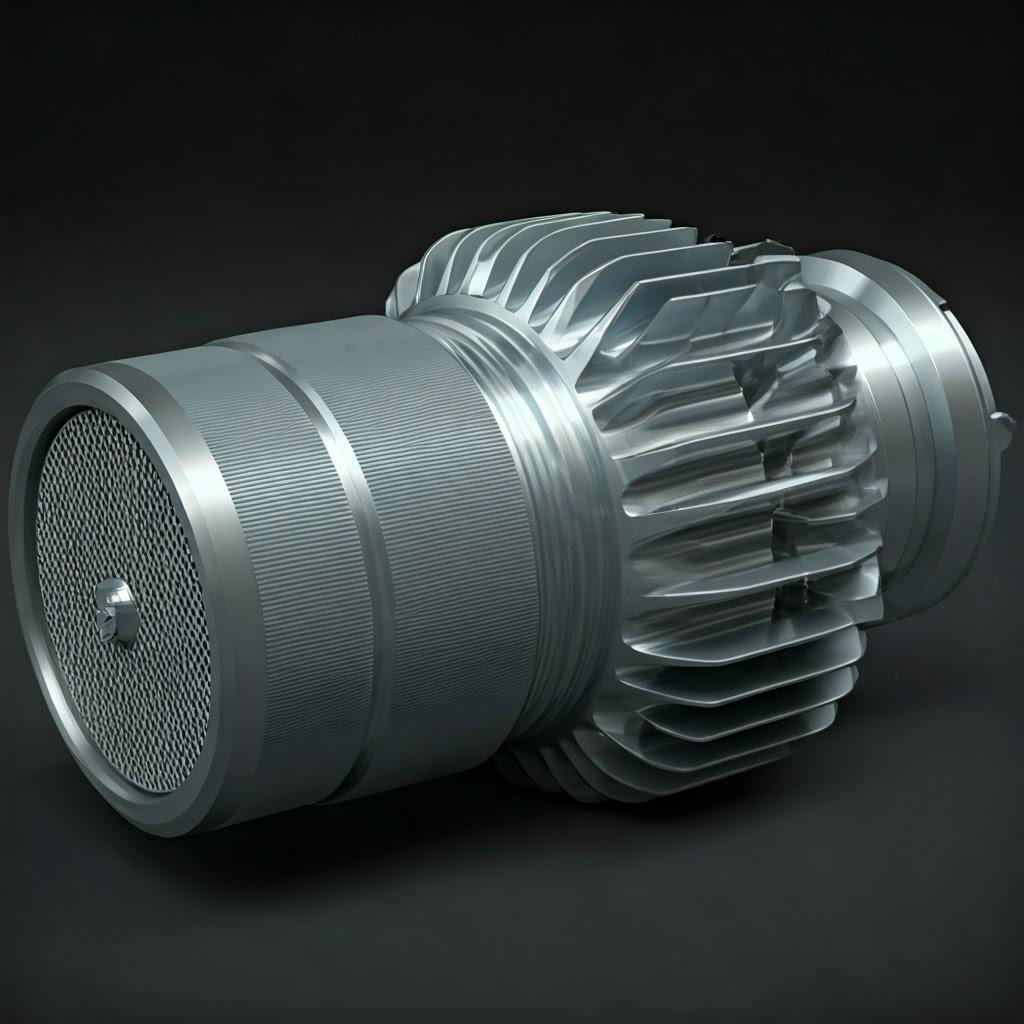
Selecting Your B2B Partner: Choosing the Right Metal AM Service Provider
The success of your additively manufactured cooling jacket project hinges not only on a well-optimized design and correct material selection but also significantly on the capabilities and expertise of your chosen metal AM service provider. Selecting the right B2B partner is a critical decision for procurement managers and engineering teams aiming for high-quality, reliable components delivered on time and within budget. Going beyond just price, a thorough evaluation should encompass technical expertise, quality systems, capacity, and overall partnership potential.
Key Criteria for Evaluating Metal AM Suppliers:
- Demonstrated Expertise & Experience:
- Application Specificity: Does the provider have proven experience with thermal management components like heat exchangers or cooling jackets? Can they showcase relevant case studies or examples?
- Experiencia en materiales: Do they have deep knowledge and validated processes for the specific materials required (AlSi10Mg, CuCrZr)? This includes understanding optimal print parameters, heat treatments, and achievable properties. Ask about their experience handling the nuances of printing copper alloys if CuCrZr is your choice.
- Experiencia en el sector: Have they worked with clients in your industry (automotive, aerospace, industrial)? Understanding industry-specific requirements and standards is crucial.
- Technology & Machine Park:
- Appropriate Technology: Ensure they utilize suitable AM technology, primarily Laser Powder Bed Fusion (LPBF/SLM) for these materials and applications.
- Machine Quality & Capacity: What is the make, model, and age of their printers? A well-maintained, modern machine park (like the systems used by Met3dp) often translates to better consistency and reliability. Do they have sufficient capacity to handle your prototyping and potential production volumes? Evaluate redundancy in case of machine downtime.
- Control de procesos: What measures are in place for process monitoring and control during the build?
- Material Quality & Handling:
- Powder Sourcing: Where do they source their metal powders? Do they have stringent quality control for incoming materials? Met3dp, for instance, manufactures its own high-quality spherical metal powders using advanced gas atomization and PREP technologies, ensuring consistency from the source. Learn more about Met3dp’s commitment to quality here.
- Manipulación y reciclaje de polvos: What are their procedures for powder storage, handling, sieving, and recycling to prevent contamination and ensure traceability?
- In-House Post-Processing Capabilities:
- Comprehensive Services: Does the provider offer the necessary post-processing steps in-house (stress relief, heat treatment, support removal, basic finishing)? While outsourcing some steps like complex CNC machining or specialized testing is common, having core capabilities in-house often streamlines the workflow, improves quality control, and reduces lead times.
- Network of Partners: If they outsource certain steps, do they have a network of qualified and trusted partners?
- Quality Management System (QMS) & Certifications:
- Robust QMS: A strong QMS is essential for ensuring consistency and repeatability. Ask about their quality procedures, inspection processes, and documentation practices.
- Relevant Certifications: Busque certificaciones como ISO 9001 (general quality management). For aerospace applications, AS9100 is often required. Certifications indicate a commitment to standardized quality processes, a key factor for industrial procurement.
- Design Support & DfAM Expertise:
- Collaborative Approach: Are their engineers available to collaborate on Design for Additive Manufacturing (DfAM)? Can they provide feedback on your design’s printability, suggest optimizations for performance or cost reduction, and help navigate AM-specific challenges? This partnership can be invaluable, especially if your team is new to metal AM.
- Project Management & Communication:
- Clear Communication: How responsive and transparent is the provider? Will you have a dedicated point of contact? Clear communication is vital throughout the project lifecycle.
- Quoting & Lead Times: Are their quotes detailed and easy to understand? Are their lead time estimations realistic and consistently met?
- References & Track Record:
- Client Testimonials/Case Studies: Ask for references or examples of similar projects they have successfully completed.
- Estabilidad financiera: For long-term B2B supply agreements, assessing the provider’s stability is also prudent.
By carefully evaluating potential proveedores de fabricación aditiva against these criteria, you can select a partner like Met3dp, who not only possesses the technical capabilities but also aligns with your quality expectations and project goals, ensuring a smooth path from design to finished, high-performance cooling jackets.
Understanding Cost Drivers and Lead Times for AM Cooling Jackets
Additive manufacturing offers significant design and performance advantages, but understanding the factors that influence cost and lead time is essential for budgeting, planning, and making informed decisions, particularly for B2B procurement activities involving custom or volume production.
Primary Cost Drivers:
- Material Type and Volume:
- Coste del material: The raw material powder cost varies significantly. Copper alloys like CuCrZr are substantially more expensive per kilogram than aluminum alloys like AlSi10Mg.
- Part Volume & Weight: The amount of material used directly impacts cost. Larger or denser parts consume more powder. DfAM practices like topology optimization can significantly reduce material usage and thus cost.
- Part Complexity and Print Time:
- Build Height (Z-Height): Print time is largely determined by the number of layers required, making taller parts generally take longer (and cost more) than shorter ones, assuming similar volume.
- Part Volume & Density: The total volume of material to be melted affects the time spent by the laser scanning each layer.
- Complexity & Supports: Intricate designs may require extensive support structures, increasing both print time (supports also need to be printed) and post-processing labor for removal. Optimized DfAM minimizes support needs.
- Nesting/Machine Utilization: Service providers aim to maximize the number of parts printed simultaneously on a single build plate (nesting). The efficiency of nesting impacts the allocated machine time cost per part.
- Costes de la máquina:
- Machine Hourly Rate: AM service providers factor in the capital cost of the sophisticated LPBF machines, maintenance, consumables (filters, gas), and operator time into an hourly machine rate.
- Requisitos de postprocesamiento:
- Labor & Equipment: Each post-processing step (stress relief, support removal, machining, finishing, testing) adds cost based on the labor intensity, required skill level, and specialized equipment usage (e.g., furnace time, CNC machine time, AFM).
- Complexity Impact: Complex internal channels requiring difficult support removal or specialized smoothing (like AFM) will incur higher post-processing costs. Machining multiple tight-tolerance features also adds significant cost compared to parts requiring minimal finishing.
- Quality Assurance & Inspection:
- Level of Inspection: The required level of quality control impacts cost. Basic dimensional checks are standard, but requirements like 100% leak testing, CT scanning for internal integrity, detailed CMM reports, or material certifications add time and cost.
- Order Volume & Setup:
- Setup Costs: There are fixed costs associated with setting up each build (programming, machine preparation, powder loading). These setup costs are amortized over the number of parts in a batch.
- Descuentos por volumen: For larger pedidos al por mayor or recurring production runs, per-part costs typically decrease due to amortization of setup costs and potential efficiencies in printing and post-processing batches. Discuss volume pricing tiers with your supplier.
Typical Lead Time Components:
Lead time is the total time from order placement to receiving the finished parts. It comprises several stages:
- Quoting & Order Confirmation: (1-3 days)
- Engineering Review & File Prep: DfAM checks, support strategy planning, build file generation. (1-2 days)
- Machine Queue: Waiting for an available machine slot. (Variable: days to weeks, depending on provider workload)
- Imprimiendo: The actual build time. (Hours to several days, depending on size/complexity/nesting)
- Cool Down & Depowdering: Letting the build chamber cool, removing the build plate, initial powder removal. (Several hours to 1 day)
- Stress Relief / Heat Treatment: Furnace cycle time. (1-2 days, including heating/cooling)
- Removal from Plate & Support Removal: (Hours to days, highly dependent on complexity)
- Post-procesamiento: Machining, finishing, cleaning, etc. (Days to weeks, depending on requirements)
- Quality Inspection & Testing: (1-3 days)
- Packing & Shipping: (1-2 days + transit time)
Estimated Timelines:
- Prototypes (1-5 units): Normalmente 1 to 3 weeks, depending heavily on complexity, material, current machine loading, and required post-processing.
- Low Volume Production (10-100 units): Often De 3 a 6 semanas.
- Higher Volume Production: Requires careful planning with the supplier; lead times depend on capacity, dedicated resources, and potential need for multiple machines.
It’s crucial to discuss specific cost and lead time expectations with your chosen AM contract manufacturer based on your final design and requirements. Providing clear specifications and realistic timelines facilitates accurate quoting and planning.
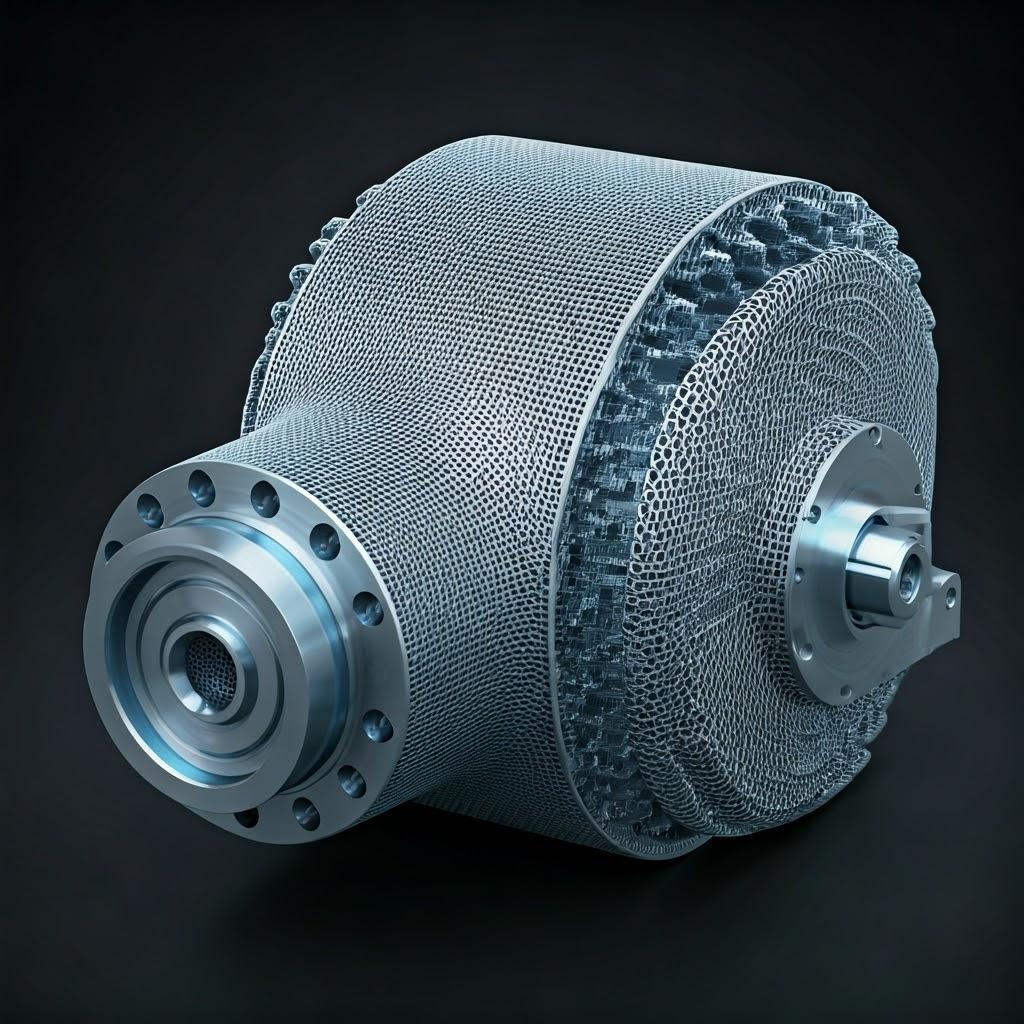
Frequently Asked Questions (FAQ) about AM Motor Cooling Jackets
Here are answers to some common questions engineers and procurement specialists have about using additive manufacturing for electric motor cooling jackets:
- Q1: How does the performance of AM cooling jackets compare to traditional ones (cast, fabricated)?
- A: Additively manufactured cooling jackets often demonstrate significantly improved thermal performance compared to their traditional counterparts. The key advantage lies in the design freedom offered by AM. Engineers can create highly complex internal cooling channels that conform precisely to the motor’s heat sources (conformal cooling) and incorporate features like turbulators or internal fins to maximize surface area and heat transfer coefficients. Studies and application examples have shown potential improvements of 20-50% or even higher in heat dissipation capability, leading to lower operating temperatures, increased power density, or extended motor life. However, the exact performance gain depends heavily on the specific design optimization achieved through DfAM compared to the baseline traditional design.
- Q2: What are the typical maximum operating temperature and pressure limits for AlSi10Mg or CuCrZr cooling jackets?
- A: The operational limits depend heavily on the specific design (especially wall thickness), the post-print heat treatment applied, and the overall system integration.
- Temperatura: AlSi10Mg performs well up to around 150°C (302°F), with mechanical properties starting to degrade significantly above 200°C (392°F). Properly processed CuCrZr retains good strength and excellent thermal conductivity at higher temperatures, potentially usable up to 300-400°C (572-752°F) or higher depending on the specific aging treatment and stress levels.
- Pressure: The pressure limit is primarily a function of the design’s structural integrity (wall thickness, geometry, support features) and the material’s yield strength. AM jackets can be designed and validated through simulation (FEA) and rigorous pressure testing (including burst testing) to withstand typical coolant loop pressures (e.g., 2-10 bar or higher). It is crucial to define operating requirements and perform appropriate validation testing on the final part design. General material properties alone do not guarantee performance; the design implementation is key.
- A: The operational limits depend heavily on the specific design (especially wall thickness), the post-print heat treatment applied, and the overall system integration.
- Q3: Can existing cooling jacket designs originally made for casting or machining be easily converted for additive manufacturing?
- A: While it’s technically possible to print a design originally intended for casting or machining, doing so often fails to leverage the primary advantages of AM and may even be suboptimal or more expensive. Directly printing a design optimized for casting, for example, might include features like draft angles or thick walls that are unnecessary and inefficient for AM. A simple conversion is generally not recommended. To achieve the benefits of improved performance, reduced weight, and part consolidation, the cooling jacket should ideally be re-designed or significantly adapted using Design for Additive Manufacturing (DfAM) principles. This involves rethinking channel paths, optimizing topology, minimizing supports, and potentially consolidating assemblies. An AM service provider can assist in evaluating an existing design and recommending modifications for optimal AM production.
- Q4: Is metal AM suitable for high-volume production of cooling jackets?
- A: Metal AM is increasingly viable for serial production, not just prototyping. While traditional methods like casting excel at extremely high volumes (hundreds of thousands to millions) of identical parts due to low per-part cost once tooling is made, AM can be very competitive for low-to-medium volumes (tens to thousands), especially when complexity, customization, or performance are key drivers. The “break-even” point depends on part complexity, material, and the costs of traditional tooling. For highly optimized, complex cooling jackets offering significant performance gains, AM can be the most economical solution even at moderate volumes when considering total system value. Discuss your volume requirements with potential Proveedores B2B like Met3dp to evaluate production feasibility and economics.
Conclusion: Revolutionizing Electric Motor Cooling with Additive Manufacturing
The challenge of managing heat in increasingly powerful and compact electric motors is a critical hurdle across demanding industries like automotive, aerospace, and industrial automation. Traditional manufacturing methods for cooling jackets, while established, often impose limitations on design complexity and thermal efficiency. Metal additive manufacturing emerges as a powerful solution, fundamentally changing how engineers can approach thermal management design.
By leveraging the layer-by-layer build process of technologies like Laser Powder Bed Fusion, AM enables the creation of electric motor cooling jackets with unprecedented geometric freedom. This translates directly into tangible benefits:
- Superior Thermal Performance: Through conformal cooling channels and complex internal features optimized for heat transfer.
- Significant Weight Reduction: Via topology optimization and efficient material usage, crucial for mobile applications.
- Consolidación de piezas: Reducing assembly complexity, potential leak points, and simplifying the supply chain.
- Rapid Prototyping & Customization: Accelerating development cycles and enabling bespoke solutions without tooling costs.
Materials like the lightweight AlSi10Mg and the highly conductive CuCrZr, when processed using high-quality powders and optimized AM parameters, provide the foundation for these high-performance components. However, realizing these benefits requires embracing Design for Additive Manufacturing (DfAM) principles and navigating potential production challenges through careful planning and execution.
Elegir bien B2B additive manufacturing partner is paramount. Expertise in materials science, robust process control, comprehensive post-processing capabilities, and a commitment to quality – hallmarks of a provider like Met3dp – are essential for transforming innovative designs into reliable, end-use components. With decades of collective expertise and advanced capabilities in both producción de polvo metálico and additive manufacturing systems, Met3dp is uniquely positioned to support your most demanding thermal management projects.
Metal AM is no longer just a prototyping tool; it is a viable, value-adding production method ready to revolutionize electric motor cooling. By strategically implementing this technology, businesses can unlock new levels of performance, efficiency, and reliability for their electric motor applications.
Ready to explore how additive manufacturing can optimize your electric motor cooling solutions? Contact Met3dp today to discuss your project requirements with our experts.
Compartir
Facebook
Twitter
LinkedIn
WhatsApp
Correo electrónico
MET3DP Technology Co., LTD es un proveedor líder de soluciones de fabricación aditiva con sede en Qingdao, China. Nuestra empresa está especializada en equipos de impresión 3D y polvos metálicos de alto rendimiento para aplicaciones industriales.
Solicite información para obtener el mejor precio y una solución personalizada para su empresa.
Artículos relacionados
Acerca de Met3DP
Actualización reciente
Nuestro producto
CONTACTO
¿Tiene alguna pregunta? ¡Envíenos un mensaje ahora! Atenderemos su solicitud con todo un equipo tras recibir su mensaje.
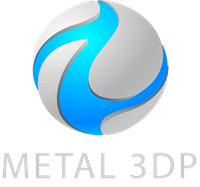
Polvos metálicos para impresión 3D y fabricación aditiva
PRODUCTO
cONTACT INFO
- Ciudad de Qingdao, Shandong, China
- [email protected]
- [email protected]
- +86 19116340731