3D Printing Custom Heat Sinks for Electronics
Índice
Introduction: Revolutionizing Thermal Management with 3D Printed Custom Heat Sinks
In the relentless pursuit of smaller, faster, and more powerful electronic devices, managing the generated heat has become a critical engineering challenge across countless industries. From densely packed server racks and high-performance computing clusters to advanced driver-assistance systems (ADAS) in vehicles, sophisticated medical imaging equipment, and powerful LED lighting arrays, effective thermal management is no longer just a desirable feature – it’s an absolute necessity for ensuring performance, reliability, and longevity. Traditional cooling solutions, often relying on extruded or machined aluminum heat sinks, have served well for decades. However, as power densities soar and component geometries become increasingly complex and space-constrained, these conventional methods often reach their limits in terms of design flexibility, performance optimization, and integration possibilities. Engineers and procurement specialists are constantly seeking innovative soluciones de gestión térmica that can overcome these limitations, pushing the boundaries of what’s possible in electronics design.
Enter the transformative world of metal additive manufacturing (AM), commonly known as metal Impresión 3D. This technology is rapidly moving beyond prototyping into functional part production, offering unprecedented capabilities for creating highly complex, performance-optimized components. One of the most promising applications lies in the production of custom heat sinks. Unlike traditional subtractive manufacturing methods that carve material away from a solid block, metal AM builds parts layer by layer directly from metal powder, guided by a digital design file. This fundamental difference unlocks a new paradigm in thermal component design, enabling the creation of geometries previously impossible or prohibitively expensive to manufacture. Imagine heat sinks with intricate internal channels mimicking organic vascular structures for enhanced fluid flow, complex lattice structures that maximize surface area while minimizing weight, or components perfectly conformal to the unique shape of the electronic device they need to cool. These are not futuristic concepts; they are tangible metal 3D printing benefits being realized today.
The ability to tailor heat sink designs precisely to the specific thermal load, airflow conditions, and spatial constraints of an application is a game-changer. Advanced cooling technology powered by metal AM allows engineers to move beyond off-the-shelf solutions and develop truly optimized thermal components. This translates directly into tangible B2B advantages: improved product performance, enhanced energy efficiency, reduced component size and weight (critical in aerospace and automotive sectors), increased design freedom leading to more integrated systems, and potentially faster time-to-market for innovative electronic products. Companies specializing in additive manufacturing electronics components are providing engineers with the tools and materials needed to tackle the most demanding thermal challenges.
Met3dp stands at the forefront of this technological shift. As a leading provider of comprehensive fabricación aditiva de metales solutions, headquartered in Qingdao, China, Met3dp offers not only state-of-the-art Selective Electron Beam Melting (SEBM) and Laser Powder Bed Fusion (LPBF) printing equipment renowned for industry-leading print volume, accuracy, and reliability, but also a portfolio of high-performance metal powders specifically optimized for AM processes. Our expertise, built on decades of collective experience, spans the entire AM ecosystem – from advanced powder production using proprietary gas atomization and Plasma Rotating Electrode Process (PREP) technologies to application development support and final part production. We partner with businesses across aerospace, medical, automotive, and industrial manufacturing sectors to leverage the power of metal AM, enabling the creation of mission-critical parts like next-generation custom heat sinks. This blog post will delve deep into the world of 3D printed custom heat sinks, exploring their applications, the advantages offered by AM, suitable materials like AlSi10Mg and CuCrZr, crucial design considerations, and how to partner with the right service provider, like Met3dp, to bring your advanced thermal management projects to life. For procurement managers and wholesale buyers, understanding the capabilities and potential of AM-produced heat sinks is crucial for sourcing components that deliver a competitive edge in performance and design.
Applications: Where are 3D Printed Custom Heat Sinks Making an Impact?
The versatility and design freedom offered by metal additive manufacturing enable the creation of custom heat sinks tailored for a vast array of demanding applications where conventional solutions fall short. The ability to optimize for specific thermal loads, flow paths, and geometric constraints makes 3D printed heat sinks particularly valuable in industries pushing the boundaries of performance and miniaturization. Procurement managers seeking reliable wholesale heat sink suppliers capable of delivering customized, high-performance solutions should pay close attention to the growing adoption of AM in these critical sectors.
Here’s a breakdown of key application areas:
1. High-Power Electronics Cooling:
- Aplicaciones: Power converters, inverters (e.g., for solar or wind energy, electric vehicles), high-frequency amplifiers, RF components, power supplies for data centers and industrial automation.
- Desafíos: These components generate significant heat in concentrated areas. Traditional heat sinks can struggle with high heat flux and uniform cooling, leading to thermal throttling or component failure. Space constraints are often severe.
- AM Advantage: Metal 3D printing allows for the creation of heat sinks with optimized fin geometries (e.g., pin fins, complex lattices), integrated microchannels for liquid cooling, or heat pipes embedded directly within the structure. Topology optimization can place material precisely where it’s needed for maximum heat dissipation while minimizing weight and volume. For B2B suppliers, offering AM solutions provides a distinct performance advantage for clients developing next-generation power systems.
2. Advanced LED Thermal Management:
- Aplicaciones: High-brightness LED modules for automotive headlights, architectural lighting, horticultural grow lights, industrial illumination, and stage lighting.
- Desafíos: LED efficiency and lifespan are highly dependent on junction temperature. Overheating leads to reduced light output, color shift, and premature failure. Compact designs often limit airflow and heat sink size.
- AM Advantage: AM enables the creation of highly conformal heat sinks that integrate seamlessly with LED modules and luminaire housings. Complex fin structures can maximize convective cooling even in tight spaces. Lightweight designs, especially using materials like AlSi10Mg, are beneficial for large arrays or weight-sensitive applications. LED thermal management solutions produced via AM can significantly improve product reliability and performance metrics, a key selling point for lighting manufacturers and distributors.
3. Automotive Electronics Cooling:
- Aplicaciones: Engine Control Units (ECUs), transmission controllers, ADAS processors, infotainment systems, battery management systems (BMS) in electric vehicles (EVs), power electronics for EV drivetrains.
- Desafíos: Harsh operating environments (vibration, temperature extremes), strict space and weight limitations, and the need for high reliability are paramount. Increasingly powerful processors and power components generate substantial heat.
- AM Advantage: Automotive electronics cooling benefits immensely from AM’s ability to produce lightweight, complex, and robust heat sinks. Topology optimization is key for reducing vehicle weight, improving fuel efficiency or EV range. Conformal cooling channels or integrated liquid cooling plates can be designed for maximum effectiveness within the confined spaces of a vehicle chassis or engine bay. Reliability is enhanced through optimized thermal design and robust materials like AlSi10Mg or even copper alloys for demanding power applications. Automotive suppliers leveraging AM can offer superior thermal solutions for Tier 1 and OEM clients.
4. Aerospace and Defense Components:
- Aplicaciones: Avionics cooling, radar systems, electronic warfare (EW) systems, power supplies for satellites and aircraft, cooling for directed energy systems.
- Desafíos: Extreme operating conditions (temperature swings, pressure changes, high G-forces, vibration), stringent weight reduction requirements (buy-to-fly ratio), and the need for exceptionally high reliability define this sector. Complex system integration often requires highly customized component shapes.
- AM Advantage: Metal AM is a natural fit for componentes aeroespaciales. It allows for the creation of highly complex, organically shaped heat sinks that integrate multiple functions, maximize surface area-to-volume ratios, and drastically reduce weight through lattice structures and topology optimization. The ability to print high-performance alloys ensures durability and reliability in demanding environments. Companies like Met3dp, with expertise in aerospace-grade materials and processes, are crucial partners for defense contractors and aerospace manufacturers seeking cutting-edge thermal solutions.
5. Industrial and High-Performance Computing (HPC):
- Aplicaciones: Cooling high-density server blades, CPUs and GPUs in data centers, processors in industrial control systems, ruggedized computers for field use, scientific instrumentation.
- Desafíos: Enormous heat loads generated by modern processors, extreme packaging density limiting airflow, the need for energy efficiency (reducing cooling costs), and high reliability for continuous operation.
- AM Advantage: AM enables advanced cooling solutions like two-phase immersion cooling manifolds, complex air-cooled heat sinks optimized for specific airflow patterns within server racks, or highly compact liquid cold plates with intricate internal channel networks. For industrial computing and data centers, even marginal improvements in cooling efficiency, enabled by optimized AM heat sinks, can translate into significant operational cost savings and improved processing performance. Suppliers offering these advanced thermal solutions provide a competitive edge in the HPC market.
6. Medical Devices:
- Aplicaciones: Cooling for medical imaging systems (MRI, CT scanners), laser systems for surgery or diagnostics, portable medical devices, processing units for complex diagnostic equipment.
- Desafíos: Strict biocompatibility requirements (sometimes), need for silent operation, high reliability, often compact device footprints, and specific thermal control needs for sensitive electronics or detectors.
- AM Advantage: AM allows for miniaturized, highly efficient heat sinks tailored to the unique forms of medical devices. Materials like Aluminum alloys or even Titanium (if biocompatibility or specific strength is needed) can be used. Complex internal structures can facilitate silent, passive cooling or highly efficient liquid cooling where required.
Across these diverse sectors, the common thread is the need for thermal management solutions that go beyond the limitations of traditional manufacturing. Metal 3D printing provides the design freedom and material capabilities to create custom thermal solutions B2B buyers require for their most challenging applications. Partnering with an experienced AM provider like Met3dp ensures access to the technology, materials, and expertise needed to leverage these advantages effectively.
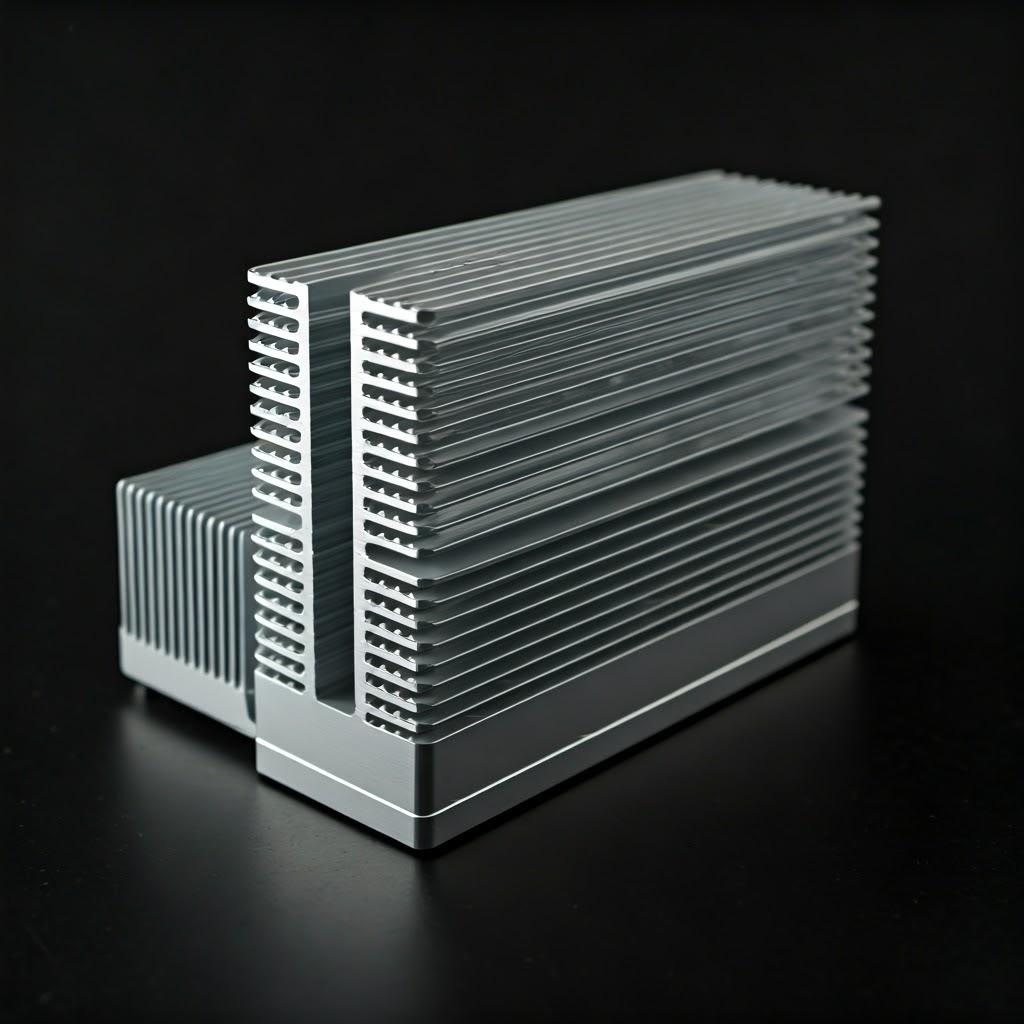
Why Metal 3D Printing for Custom Heat Sinks? Unlocking Design Freedom and Performance
While traditional manufacturing methods like CNC machining, extrusion, or casting have long been the standard for producing heat sinks, they inherently come with design constraints that can limit thermal performance, integration possibilities, and overall efficiency, especially when dealing with complex requirements. Metal additive manufacturing fundamentally changes the equation, offering compelling advantages that make it an increasingly attractive option for engineers and procurement managers seeking cutting-edge custom thermal solutions B2B. En additive manufacturing vs machining debate for heat sinks often hinges on complexity, volume, and performance requirements.
Here’s a detailed look at why metal AM is a superior choice for many custom heat sink applications:
1. Unparalleled Design Freedom & Geometric Complexity:
- Traditional Limitations: Extrusion limits designs to 2.5D shapes with consistent cross-sections. Machining, while versatile, becomes exponentially more expensive and time-consuming as complexity increases (e.g., undercuts, deep channels, thin features). Complex internal structures are often impossible.
- AM Advantage: Metal AM builds parts layer-by-layer, freeing designers from many traditional constraints. This enables:
- Complex Fin Geometries: Creation of highly optimized fin shapes like pin fins, wavy fins, or complex lattice structures (e.g., gyroids, octet-truss) that maximize surface area for convection within a given volume, significantly boosting thermal performance.
- Internal Cooling Channels: Integration of intricate, conformal cooling channels directly within the heat sink body for high-efficiency liquid cooling, eliminating the need for assembly and potential leak points. These channels can follow curved paths and adapt to component layouts.
- Conformal Designs: Heat sinks can be designed to perfectly match the curved or irregular surfaces of the components they need to cool, improving thermal contact and overall system integration.
- Consolidación de piezas: Multiple components (e.g., heat sink, flow manifold, mounting brackets) can potentially be consolidated into a single, complex 3D printed part, reducing assembly time, weight, and potential points of failure.
- Benefit: Este complex heat sink geometry capability allows engineers to design for optimal thermal performance rather than being limited by manufacturability constraints.
2. Topology Optimization for Performance and Lightweighting:
- Concept: Topology optimization software uses algorithms (often Finite Element Analysis – FEA) to determine the most efficient material distribution within a defined design space, subject to specific loads (thermal, mechanical) and constraints. It essentially “carves away” unnecessary material, leaving only the load-bearing or thermally critical structures.
- AM Advantage: AM is uniquely capable of producing the organic, often complex shapes resulting from topology optimization thermal analysis. This allows for:
- Maximum Performance: Placing thermally conductive material precisely where heat needs to be transferred and dissipated most effectively.
- Significant Lightweighting: Removing non-essential material drastically reduces component weight, a critical factor in aerospace, automotive, and portable electronics applications. Lightweight heat sink design is a major driver for AM adoption.
- Benefit: Achieving optimal thermal performance with the minimum possible mass, which is often unattainable with traditional methods due to manufacturing limitations or cost.
3. Rapid Prototyping and Iteration:
- Traditional Limitations: Creating custom tooling (e.g., extrusion dies) or complex machining setups for prototypes can be time-consuming and expensive, slowing down the design iteration cycle.
- AM Advantage: Metal AM requires no part-specific tooling. Designs can go directly from a CAD file to a physical part relatively quickly. This facilitates:
- Faster Design Cycles: Engineers can quickly produce and test multiple design variations (e.g., different fin structures, channel layouts) to empirically determine the best performing solution.
- Reduced Development Costs: Avoiding tooling costs for prototypes makes exploring innovative designs more feasible.
- Benefit: Accelerates product development and allows for more thorough design validation, leading to better final products. This rapid prototyping electronics capability is invaluable in fast-moving markets.
4. Customization and On-Demand Production:
- Traditional Limitations: Producing small batches or highly customized heat sinks via traditional methods can be economically challenging due to setup costs and tooling requirements.
- AM Advantage: The digital nature of AM makes it highly suitable for:
- Low-to-Medium Volume Production: Cost-effective manufacturing of batches ranging from single prototypes to hundreds or thousands of parts without tooling investment.
- La personalización en masa: Easily tailoring heat sink designs to specific customer requirements or application variations without significant process changes.
- Fabricación a la carta: Producing parts as needed, reducing inventory requirements and enabling faster response to fluctuating demand.
- Benefit: Provides flexibility for niche applications, customized equipment, and managing supply chain uncertainties, offering significant value for B2B procurement.
5. Potential for Enhanced Thermal Performance:
- Beyond Geometry: While complex geometries are the primary driver, the layer-by-layer process itself can sometimes be manipulated (though careful control is needed) to influence microstructure, potentially impacting thermal properties. More significantly, AM enables the use of materials and geometries that maximize heat transfer mechanisms (conduction, convection, radiation) in ways traditional methods cannot replicate efficiently. The ability to create micro-features or specific surface textures can enhance convective heat transfer or improve thermal interface material (TIM) contact.
- Benefit: Pushing the absolute limits of thermal performance by combining material properties with unparalleled geometric freedom.
Met3dp leverages its advanced SEBM and LPBF printing technologies and high-quality metal powders to fully realize these advantages for its customers. Our engineering team can assist in optimizing designs for additive manufacturing (DfAM), ensuring that the transition from concept to functional, high-performance custom heat sink is seamless and efficient. By embracing metal AM, companies can unlock superior thermal management solutions that drive innovation in their respective industries.
Material Deep Dive: AlSi10Mg and CuCrZr for Optimal Heat Sink Performance
Selecting the right material is paramount to designing an effective heat sink, regardless of the manufacturing method. However, metal additive manufacturing opens the door to using specific alloys that offer an excellent balance of thermal conductivity, mechanical properties, weight, and processability via layer-by-layer fusion. For 3D printed custom heat sinks, two materials stand out as primary choices offered by leading metal powder for 3D printing suppliers like Met3dp: AlSi10Mg (an aluminum alloy) and CuCrZr (a copper alloy). Understanding their properties is crucial for engineers designing thermal solutions and for procurement managers sourcing these advanced components.
Met3dp takes material quality extremely seriously, employing industry-leading powder manufacturing techniques like Vacuum Induction Melting Gas Atomization (VIGA) and PREP (Plasma Rotating Electrode Process). Our advanced powder making systems utilize unique nozzle and gas flow designs in gas atomization to produce highly spherical metallic powders with excellent flowability and minimal satellite particles. PREP technology yields powders with even higher purity and sphericity, ideal for the most demanding applications. This commitment ensures that the Met3dp powders used in our printers provide the foundation for dense, high-quality metal parts with superior and consistent mechanical and thermal properties – critical for reliable heat sink performance.
Let’s examine the recommended powders:
1. Aluminum-Silicon-Magnesium Alloy (AlSi10Mg): The Lightweight Workhorse
- Composición: Primarily aluminum, with significant additions of silicon (around 10%) and magnesium (around 0.3-0.5%).
- Propiedades clave:
- Conductividad térmica: Good, typically in the range of 120-180 W/(m·K) after appropriate heat treatment. While lower than pure aluminum or copper, it’s significantly better than steels or titanium alloys.
- Densidad: Low density (approx. 2.67 g/cm³), making it ideal for applications where weight saving is critical (aerospace, automotive, portable devices).
- Resistencia mecánica: Offers good strength and hardness, especially after heat treatment (T6 condition), making the resulting heat sinks robust.
- Procesabilidad: One of the most mature and well-understood alloys for Laser Powder Bed Fusion (LPBF/SLM), allowing for fine features and good surface finish.
- Resistencia a la corrosión: Generally good atmospheric corrosion resistance, which can be further enhanced by anodizing.
- Costo: Relatively cost-effective compared to copper alloys or other exotic materials.
- Why it Matters for Heat Sinks: AlSi10Mg provides an excellent balance of good thermal conductivity, low weight, good mechanical strength, and processability. It’s often the default choice when extreme thermal conductivity isn’t the absolute primary driver, but a combination of performance, weight, and manufacturability is required. Its widespread use means processing parameters are well-established, leading to reliable and repeatable results. The ability to achieve significant lightweight heat sink design makes it highly valuable.
- Met3dp Advantage: Met3dp produces high-quality AlSi10Mg powder optimized for LPBF processes, ensuring consistent melt pool behavior, high part density (>99.8%), and predictable final properties after appropriate post-processing, including T6 heat treatment for enhanced strength and thermal performance.
Table: AlSi10Mg Properties (Typical Values for AM Parts)
Propiedad | Typical Value (After Heat Treatment, e.g., T6) | Unidad | Significance for Heat Sinks |
---|---|---|---|
Conductividad térmica | 120 – 180 | W/(m-K) | Good heat transfer capability |
Densidad | ~2.67 | g/cm³ | Excellent for lightweight designs |
Límite elástico | 230 – 300 | MPa | Provides structural integrity and robustness |
Resistencia a la tracción | 350 – 450 | MPa | Indicates overall strength |
Alargamiento a la rotura | 3 – 10 | % | Indicates ductility (resistance to brittle fracture) |
Processability (LPBF) | Excelente | – | Allows complex geometries, fine features, good surface finish |
Coste relativo | Moderado | – | Cost-effective balance of properties |
Export to Sheets
2. Copper-Chromium-Zirconium Alloy (CuCrZr): The High-Conductivity Champion
- Composición: Primarily copper, with small additions of chromium (Cr) and zirconium (Zr).
- Propiedades clave:
- Conductividad térmica: Excellent, approaching that of pure copper (typically >300 W/(m·K) after heat treatment). This is significantly higher than aluminum alloys.
- Conductividad eléctrica: Also very high, which can be relevant if the heat sink also serves an electrical function.
- Densidad: High density (approx. 8.9 g/cm³), similar to pure copper. This is a key consideration where weight is a major constraint.
- Resistencia mecánica: Good strength and hardness, particularly after precipitation hardening (aging) heat treatments, significantly better than pure copper. Retains strength well at moderately elevated temperatures.
- Procesabilidad: More challenging to process via LPBF than AlSi10Mg due to copper’s high reflectivity and thermal conductivity, requiring higher laser power and careful parameter control. Susceptible to oxidation. Often processed under specific atmosphere conditions.
- Resistencia a la corrosión: Good, but can tarnish or oxidize in certain environments.
- Costo: Significantly more expensive than AlSi10Mg, both in terms of raw powder cost and processing difficulty.
- Why it Matters for Heat Sinks: CuCrZr is the material of choice when maximizing thermal performance is the absolute priority and weight is a secondary concern. Its high thermal conductivity allows for rapid heat spreading and dissipation, making it ideal for applications with very high heat flux or where the lowest possible component temperatures are required (e.g., high-power electronics, demanding RF components, laser diodes). The CuCrZr properties, specifically its thermal conductivity, enable performance levels unattainable with aluminum.
- Met3dp Advantage: Processing copper alloys requires specialized expertise and equipment. Met3dp’s experience and advanced printing systems are capable of handling challenging materials like CuCrZr. We provide high-purity CuCrZr powder and utilize optimized process parameters developed through rigorous R&D to achieve high density and optimal post-heat treatment properties, ensuring our clients can leverage the superior thermal performance of this alloy for their most demanding applications.
Table: CuCrZr Properties (Typical Values for AM Parts)
Propiedad | Typical Value (After Heat Treatment) | Unidad | Significance for Heat Sinks |
---|---|---|---|
Conductividad térmica | >300 | W/(m-K) | Excellent heat transfer, ideal for high heat flux |
Densidad | ~8.9 | g/cm³ | Heavy, potential drawback for weight-sensitive applications |
Límite elástico | 350 – 500 | MPa | Good strength, retains properties at moderate temperatures |
Resistencia a la tracción | 450 – 550 | MPa | High overall strength for a copper alloy |
Alargamiento a la rotura | 10 – 20 | % | Buena ductilidad |
Processability (LPBF) | Desafío | – | Requires high power, parameter optimization, atmosphere control |
Coste relativo | Alta | – | Higher material and processing costs |
Export to Sheets
Consideraciones sobre la selección de materiales:
The choice between AlSi10Mg and CuCrZr depends heavily on the specific application requirements:
- Choose AlSi10Mg when:
- Weight reduction is a primary goal.
- Good (but not extreme) thermal performance is sufficient.
- Cost is a significant factor.
- Complex geometries with fine features are needed, leveraging its excellent processability.
- Choose CuCrZr when:
- Maximum thermal conductivity and heat dissipation are paramount.
- High heat flux needs to be managed effectively.
- Weight is not the primary constraint (or the performance gain justifies the weight).
- Higher cost is acceptable for achieving top-tier thermal performance.
By offering aleaciones de alto rendimiento like both AlSi10Mg and CuCrZr, manufactured using superior gas atomization quality processes, Met3dp provides engineers and procurement managers the material options needed to create truly optimized, high-performance custom heat sinks through the power of metal additive manufacturing. Our team can assist in selecting the ideal material based on your specific thermal, mechanical, and economic requirements.
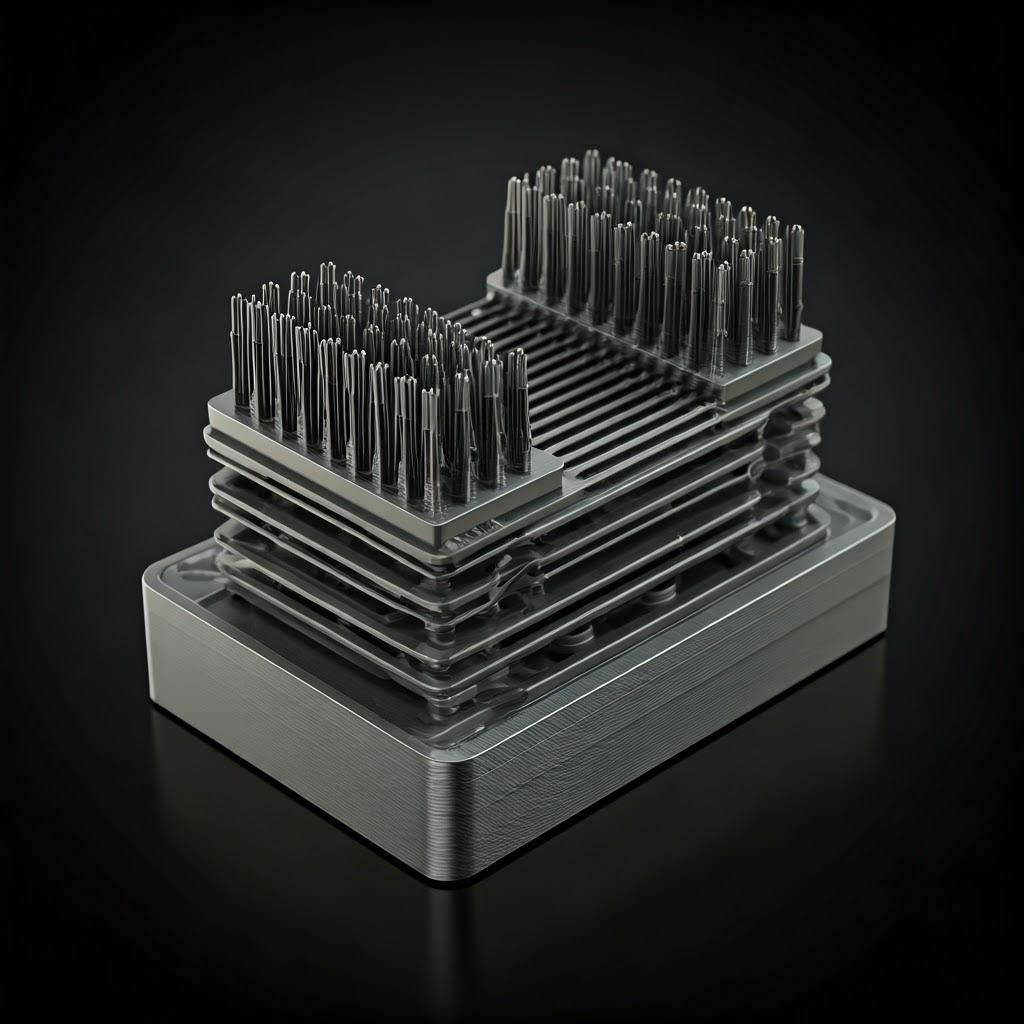
Design for Additive Manufacturing (DfAM): Optimizing Heat Sinks for 3D Printing
Simply replicating a heat sink design intended for machining or extrusion using additive manufacturing rarely unlocks the full potential of the technology. To truly leverage the benefits of metal 3D printing – unparalleled geometric complexity, lightweighting, and potentially superior thermal performance – engineers must embrace Design for Additive Manufacturing (DfAM). DfAM is not just a set of rules; it’s a change in mindset, encouraging designers to think layer-by-layer and utilize the unique capabilities of AM processes like Selective Laser Melting (SLM) / Laser Powder Bed Fusion (LPBF) or Selective Electron Beam Melting (SEBM). For custom heat sinks, applying DfAM thermal management principles is crucial for creating components that outperform their traditional counterparts.
Here are key DfAM considerations specifically for optimizing heat sinks:
1. Harnessing Complex Geometries: Beyond Simple Fins
- Estructuras reticulares: AM excels at creating intricate, periodic structures within a volume. For heat sinks, this means:
- Tipos: Triply Periodic Minimal Surfaces (TPMS) like Gyroids or Schwarz-P structures, or strut-based lattices like Body-Centered Cubic (BCC) or Octet-truss.
- Ventajas: These structures offer an exceptionally high surface area-to-volume ratio, significantly enhancing convective heat transfer compared to traditional fins. They can also promote fluid mixing in liquid-cooled applications and contribute to significant lightweighting.
- Consideraciones: Cell size, strut/wall thickness, and overall density must be carefully chosen based on thermal requirements, fluid flow (pressure drop), and printability constraints (minimum feature size, powder removal). Designing a lattice structure heat sink requires specialized software tools and an understanding of AM limitations.
- Biomimicry & Organic Shapes: Nature often optimizes for efficient transport and structural integrity. DfAM allows designers to take inspiration from biological structures (e.g., vascular networks, lung alveoli) to create highly efficient, non-intuitive flow paths or heat-spreading geometries.
- Function Integration: Combine features like mounting points, flow distributors, or sensor housings directly into the heat sink structure, reducing part count and assembly complexity.
2. Thin Walls, High Aspect Ratios, and Fin Optimization:
- AM allows for thinner walls and fins than often practical with machining or casting. This can increase surface area density. However, there are limits:
- Minimum Thickness: Determined by the AM process (laser spot size, powder characteristics), typically around 0.3-0.5 mm for reliable printing, though thinner features may be possible with careful parameter tuning.
- Aspect Ratio: Very tall, thin fins can be prone to vibration during printing or residual stress-induced distortion. Design guidelines often suggest limiting aspect ratios (height-to-thickness) or incorporating stiffening features.
- Thermal Performance: While thinner fins increase density, overly thin fins can suffer from poor heat conduction from the base to the tip (fin efficiency). DfAM involves balancing surface area with conductive path effectiveness. Thin wall design AM requires careful simulation (CFD/FEA).
3. Designing for Internal Channels (Liquid Cooling):
- AM enables monolithic cold plates with complex internal channels.
- Self-Supporting Shapes: Designing channels with teardrop or diamond cross-sections (angles typically > 45° from horizontal) allows them to be printed without internal support structures, simplifying post-processing significantly.
- Support Considerations: If complex horizontal or low-angle internal channels are necessary, internal supports might be required. These can be extremely difficult or impossible to remove completely. DfAM focuses on avoiding internal supports wherever possible.
- Powder Removal: Channels must be designed with adequate inlet/outlet ports and smooth paths to facilitate complete removal of unmelted powder after printing. Dead-end channels or sharp corners that trap powder should be avoided.
- Flow Optimization: Channel paths, cross-sections, and internal features (like turbulators, achievable with AM) can be optimized using Computational Fluid Dynamics (CFD simulation AM) to maximize heat transfer and minimize pressure drop.
4. Topology Optimization for Thermal Performance:
- As mentioned earlier, topology optimization software is a powerful DfAM tool. For heat sinks, it identifies the most efficient pathways for heat conduction and material placement for convective surfaces.
- Workflow: Define the design space, heat sources, boundary conditions (airflow, ambient temperature), target mass reduction, and performance goals. The software generates an optimized, often organic-looking shape.
- Ventajas: Creates the stiffest, most thermally efficient structure possible for a given mass or volume constraint. Ideal for lightweight heat sink design in aerospace and automotive.
- Consideraciones: Optimized shapes can be complex and may require some smoothing or interpretation for manufacturing. Ensuring printability (e.g., minimum feature sizes, no enclosed voids trapping powder) is key.
5. Strategic Support Structure Design & Minimization:
- Metal AM processes require support structures for overhanging features (typically below 45° from the horizontal) and to anchor the part to the build plate, managing thermal stress.
- Minimization: The primary DfAM goal is to minimize the need for supports through clever design orientation and feature modification (e.g., using chamfers instead of sharp overhangs).
- Placement: Avoid supports on critical functional surfaces (e.g., TIM interfaces, fin surfaces) as removal can mar the surface. Place them in less critical areas or where they can be easily accessed and removed.
- Tipo: Choose appropriate support types (e.g., solid block, lattice, cone, tree supports) based on the geometry and ease of removal needs. Some support types use less material and are easier to detach.
- Ease of Removal: Design supports with appropriate connection points (e.g., perforated or tapered contacts) to facilitate breaking them off without damaging the part. Consider access for tools. Support structure optimization is critical for cost-effective AM.
6. Build Orientation Strategy:
- How a part is oriented on the build platform significantly impacts:
- Support Requirements: Directly influences the amount and location of supports needed.
- Acabado superficial: Downward-facing surfaces tend to be rougher than upward-facing or vertical surfaces. Critical surfaces should ideally be oriented upwards or vertically.
- Tiempo de construcción: Taller builds generally take longer. Orienting to minimize Z-height can speed up printing.
- Residual Stress & Warping: Orientation affects thermal gradients and stress accumulation. Strategic orientation can help mitigate warping.
- Anisotropía: Mechanical and sometimes thermal properties can vary slightly depending on the build direction relative to the part’s geometry. This needs consideration for performance-critical applications.
- En build orientation impact requires careful consideration during the design and print preparation stages.
7. Adhering to Process Constraints:
- Designers must be aware of the specific AM machine’s capabilities:
- Tamaño mínimo de característica: Smallest reliable feature (wall, hole, strut) achievable.
- Minimum Hole Diameter: Small holes can be challenging to print accurately and clear of powder.
- Maximum Build Size: Ensure the part fits within the printer’s build volume.
- Grosor de la capa: Affects resolution, surface finish, and build speed.
Applying these heat sink design guidelines for AM requires collaboration between designers and AM specialists. Met3dp’s application engineering team works closely with clients, providing DfAM expertise to optimize their heat sink designs for our advanced SEBM and LPBF systems. By leveraging DfAM, businesses can unlock the full potential of additive manufacturing, creating custom heat sinks seen on our product showcase that offer superior performance, reduced weight, and enhanced integration compared to traditional alternatives. Procurement managers should favor suppliers who demonstrate strong DfAM capabilities, as this directly translates to better, more cost-effective final parts.
Achieving Precision: Tolerance, Surface Finish, and Accuracy in 3D Printed Heat Sinks
While metal additive manufacturing unlocks incredible design freedom, potential users, especially engineers and procurement managers accustomed to the precision of CNC machining, often have questions about the achievable levels of tolerance, surface finish, and overall dimensional accuracy. Understanding these aspects is crucial for managing expectations, designing effectively, and determining necessary post-processing steps for functional heat sinks. Precision manufacturing in AM involves careful control over the entire process chain, from powder quality to machine calibration and post-processing.
1. Dimensional Tolerances:
- Typical Values: Metal AM processes like LPBF and SEBM typically achieve dimensional tolerances in the range of ±0.1 mm to ±0.2 mm for smaller features (e.g., up to 50-100 mm), or ±0.1% to ±0.2% of the nominal dimension for larger parts. These are general guidelines and can vary significantly based on:
- Machine Calibration: Well-maintained, high-quality machines like those used by Met3dp offer better accuracy.
- Part Geometry & Size: Larger, more complex parts are more susceptible to thermal distortion, potentially impacting overall accuracy.
- Material: Different materials exhibit varying shrinkage and stress behaviors during printing.
- Orientación de construcción: Affects thermal history and potential for distortion.
- Support Strategy: Effective support placement is crucial for maintaining accuracy during the build.
- Post-procesamiento: Stress relief heat treatments can sometimes cause minor dimensional changes.
- Comparación: While impressive for a layer-wise fusion process, these tolerances are generally looser than those achievable with precision CNC machining (which can reach ±0.01 mm or better for critical features).
- Implication: For heat sink features requiring very tight tolerances (e.g., mounting holes, surfaces mating with sensitive components, TIM interfaces), relying solely on as-built AM tolerances might not be sufficient. Post-machining of these critical features is often necessary.
2. Surface Finish (Roughness):
- As-Built Condition: The nature of layer-by-layer powder fusion results in a characteristic surface texture. As-built surface roughness (Ra) for metal AM parts typically ranges from 5 µm to 20 µm (micrometers). This depends heavily on:
- Orientation:
- Upward-facing (Top) Surfaces: Generally the smoothest, as they are formed by the top layer of melted powder.
- Vertical (Side) Walls: Show layer lines, resulting in moderate roughness.
- Downward-facing (Bottom/Overhang) Surfaces: Tend to be the roughest due to the attachment points of support structures and the interaction with partially sintered powder below.
- Process Parameters: Layer thickness, laser/electron beam power, scan speed all influence the melt pool dynamics and resulting surface.
- Material & Powder Size: Finer powders can potentially lead to smoother surfaces but present other challenges.
- Orientation:
- Impact on Heat Sink Performance:
- Thermal Interface Material (TIM) Compatibility: As-built roughness can be too high for optimal thermal contact with flat components, potentially trapping air or requiring thicker TIM layers, increasing thermal resistance. Polishing or machining the contact surface is often recommended for best performance.
- Convective Heat Transfer: While extreme smoothness isn’t always necessary for convection, very high roughness (especially on internal channels) can increase flow resistance (pressure drop) in liquid or forced-air cooling systems. However, moderate roughness can sometimes slightly enhance turbulent flow, aiding heat transfer in specific regimes.
- Radiation: Surface finish and subsequent coatings (like high-emissivity paints or anodizing) significantly impact radiative heat transfer, which can be relevant in vacuum or natural convection environments.
- Met3dp’s Approach: Met3dp utilizes optimized process parameters and high-quality, spherical powders produced via advanced gas atomization and PREP methods to achieve the best possible as-built surface finish within the inherent capabilities of LPBF and SEBM processes. Our quality control measures ensure consistency across builds.
3. Dimensional Accuracy & Warping:
- Desafío: The rapid heating and cooling cycles inherent in powder bed fusion can generate significant internal stresses within the part. If not managed properly, these stresses can lead to warping or distortion, particularly after the part is cut from the build plate and stress is relieved unevenly.
- Mitigation Strategies:
- Process Simulation: Simulating the build process beforehand can predict areas of high stress and potential distortion, allowing for adjustments to orientation or support strategy.
- Optimized Parameters: Fine-tuned melt parameters minimize thermal gradients.
- Robust Support Structures: Securely anchoring the part prevents movement during the build and helps manage stress.
- Stress Relief Heat Treatment: A critical post-processing step (discussed next) performed before support removal to homogenize the microstructure and reduce internal stress, stabilizing the part’s dimensions.
- Result: By employing these strategies, reputable AM providers like Met3dp can achieve high levels of dimensional accuracy AM parts require, minimizing deviation from the intended CAD model. However, designers should still account for standard AM tolerances and plan for post-machining where ultimate precision is needed.
Table: AM Precision Overview for Heat Sinks
Parámetro | Typical As-Built Range (LPBF/SEBM) | Key Influencing Factors | Impact on Heat Sinks & Solutions |
---|---|---|---|
Tolerancia dimensional | ±0.1 to ±0.2 mm or ±0.1% to ±0.2% | Machine, material, geometry, size, orientation, supports | Sufficient for many features; Critical interfaces often require post-machining. |
Rugosidad superficial (Ra) | 5 – 20 µm | Orientation, parameters, powder, material | Affects TIM contact (polish/machine contact surfaces), flow resistance (consider in CFD). |
Warping/Distortion | Can occur if not managed | Thermal stress, support strategy, part geometry, stress relief | Mitigated by simulation, supports, orientation, essential stress relief heat treatment. |
Quality Control Metal AM | Crucial throughout | Powder handling, machine calibration, process monitoring | Ensures consistency and reliability; Provided by experienced suppliers like Met3dp. |
Export to Sheets
In summary, while metal 3D printing may not match the sub-micron precision of specialized machining in its as-built state, it offers remarkable accuracy for a direct digital manufacturing process. By understanding the typical tolerances and surface finishes, designing accordingly (DfAM), and incorporating appropriate post-processing steps, engineers can confidently utilize metal AM to produce highly functional, complex custom heat sinks that meet demanding performance requirements. Partnering with a provider focused on quality control metal AM ensures these precision levels are consistently met.
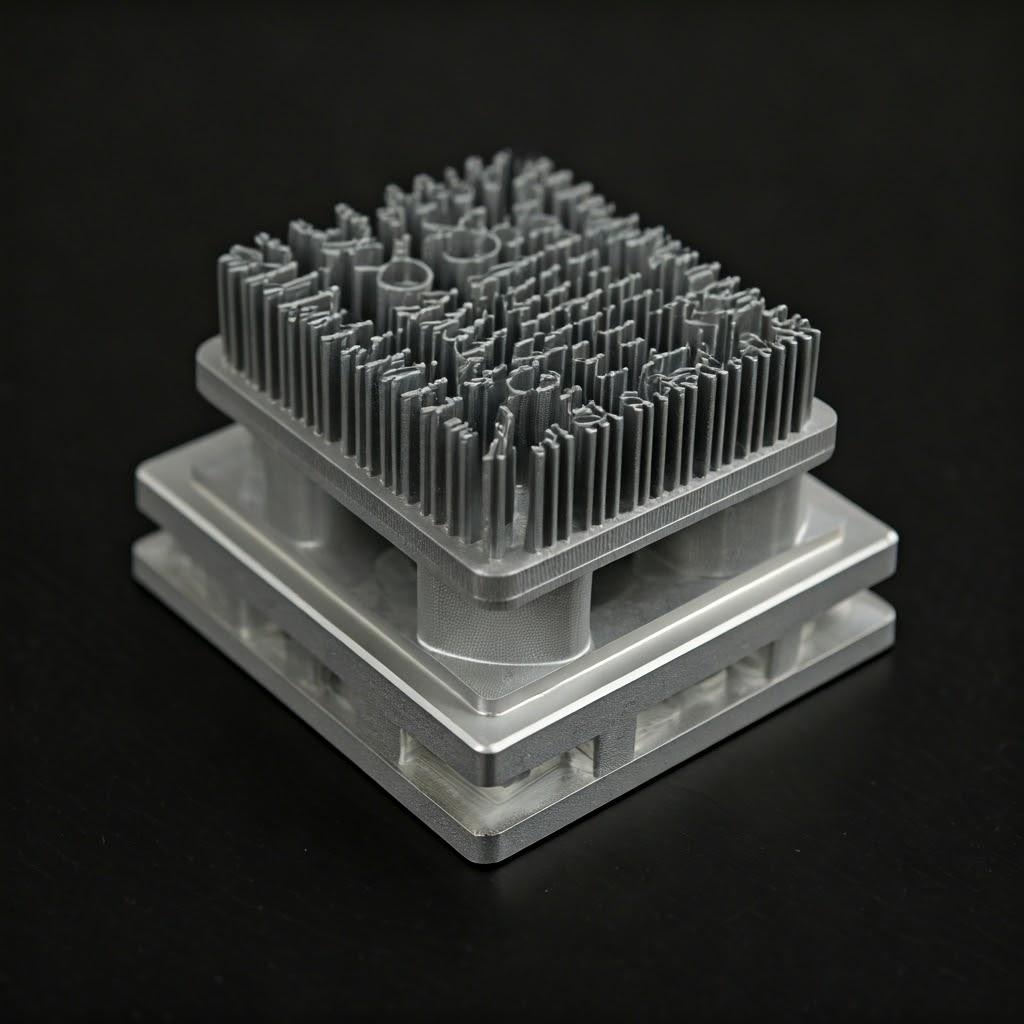
Beyond Printing: Essential Post-Processing for Functional Heat Sinks
Creating a custom heat sink using metal additive manufacturing involves more than just hitting “print.” The as-built part, fresh off the printer, is rarely ready for immediate deployment. A series of crucial post-processing steps are typically required to transform the raw printed component into a fully functional, reliable, and high-performance heat sink that meets engineering specifications. Understanding these steps is vital for procurement managers budgeting projects and for engineers designing parts, as post-processing requirements can significantly impact final cost, lead time, and component performance. Reputable AM service providers offer comprehensive solutions that include these essential finishing steps, often providing tailored B2B finishing services.
Here’s a breakdown of common post-processing stages for 3D printed metal heat sinks:
1. Stress Relief Heat Treatment:
- Propósito: To alleviate the internal stresses accumulated during the rapid heating and cooling cycles of the layer-by-layer fusion process. This is particularly critical for materials like AlSi10Mg printed via LPBF. Without stress relief, parts can warp or distort when cut from the build plate or during subsequent machining.
- Proceso: The entire build plate with the parts still attached is placed in a furnace and heated to a specific temperature (below the material’s aging temperature, e.g., around 300°C for AlSi10Mg) for a defined period, followed by controlled cooling. This allows the material’s microstructure to relax, reducing residual stress.
- Importance: Absolutely essential for dimensional stability and preventing premature failure. It’s typically the very first step after the build completes and the chamber cools.
2. Part Removal from Build Plate:
- Propósito: To separate the printed heat sink(s) from the metal base plate they were built upon.
- Métodos: Commonly done using wire Electrical Discharge Machining (EDM) or a bandsaw. Care must be taken not to damage the parts. The support structures connecting the part to the plate are cut through.
- Consideraciones: The removal method can influence the surface finish of the part’s base.
3. Support Structure Removal:
- Propósito: To remove the temporary structures that supported overhanging features and anchored the part during printing.
- Métodos: This can be one of the most labor-intensive steps, especially for complex geometries.
- Eliminación manual: Supports are often designed with weakened connection points and can be broken off manually using pliers or hand tools.
- Machining/Grinding: More stubborn supports or those on critical surfaces may need to be removed by CNC machining or manual grinding/linishing.
- Wire EDM: Sometimes used for precise removal in tight areas.
- Desafíos: Accessing internal supports within complex channels or lattice structures can be extremely difficult. Support removal metal AM requires careful planning during the DfAM stage to ensure accessibility and minimize the amount of support needed. Damage can occur if removal is not done carefully.
4. Powder Removal (Depowdering):
- Propósito: To remove any unfused metal powder trapped within the part, especially in internal channels, lattices, or complex voids. Residual powder can add weight, potentially compromise thermal performance if blocking flow paths, and become loose later.
- Métodos: Compressed air blowing, vibration tables, ultrasonic cleaning baths, and sometimes specialized flushing systems are used.
- Importance: Critical for parts with internal features, like liquid-cooled cold plates or heat sinks with dense lattice structures. DfAM plays a role here too – designing escape holes and smooth internal pathways aids depowdering.
5. Further Heat Treatment (Aging/Hardening):
- Propósito: To optimize the material’s mechanical properties (strength, hardness) and, importantly for heat sinks, often its thermal conductivity.
- AlSi10Mg: Typically undergoes a T6 solution heat treatment and artificial aging process. This involves heating to a higher temperature (around 500-540°C) to dissolve precipitates, quenching rapidly, and then aging at a lower temperature (around 150-170°C) to form fine precipitates that strengthen the material. The heat treatment AlSi10Mg receives significantly impacts its final properties. T6 condition generally offers the best combination of strength and thermal conductivity for this alloy.
- CuCrZr: Requires precipitation hardening (aging) at specific temperatures (e.g., 450-500°C) to form Cr and Zr precipitates, which dramatically increase strength and hardness while largely retaining the high thermal and electrical conductivity of the copper matrix.
- Importance: Essential for achieving the target material specifications listed in datasheets and ensuring the heat sink has the required strength and thermal performance.
6. Surface Finishing:
- Propósito: To modify the as-built surface texture for improved aesthetics, function, or subsequent processing.
- Bead Blasting/Sand Blasting: Creates a uniform, non-directional matte finish by propelling fine media (glass beads, grit) at the surface. Removes minor imperfections and can provide a clean base for coatings.
- Tumbling/Vibratory Finishing: Parts are placed in a tumbler with media to deburr sharp edges and provide a smoother, more uniform finish, especially for smaller parts or those with complex external features.
- Pulido: Mechanical or electrochemical polishing can be used to achieve very smooth, reflective surfaces (low Ra). Often applied selectively to TIM interface areas to minimize thermal contact resistance.
- Options: The choice depends on the desired outcome – aesthetic uniformity, specific roughness value, or preparation for coating. A range of surface finishing options are available.
7. CNC Machining:
- Propósito: To achieve tighter tolerances, specific surface finishes, or features not possible with AM alone.
- Aplicaciones:
- Critical Interfaces: Machining surfaces that mate with TIMs or other components to ensure flatness and required roughness.
- Tight Tolerances: Achieving dimensional tolerances better than ±0.1mm.
- Threading/Tapping: Creating threaded holes for mounting.
- Feature Refinement: Sharpening edges or creating features difficult to define precisely in AM.
- Integration: CNC machining 3D prints is a common hybrid approach, leveraging AM for complex geometries and machining for precision features.
8. Coating / Surface Treatment:
- Propósito: To add further functionality or protection.
- Anodizing (for Aluminum alloys): Provides enhanced corrosion resistance, electrical insulation, wear resistance, and allows for coloring (aesthetics). Heat sink anodizing is common for AlSi10Mg parts.
- Plating (e.g., Nickel, Gold): Can enhance corrosion resistance, solderability, or surface conductivity.
- Painting/Powder Coating: For aesthetics or applying specialized coatings (e.g., high-emissivity paints to improve radiative cooling).
9. Quality Assurance Testing:
- Propósito: To verify the part meets all specifications before shipping.
- Métodos: Dimensional inspection (CMM, laser scanning), material property verification (e.g., hardness testing), surface finish measurement, leak testing (for liquid-cooled channels), thermal performance testing (if required), NDT (Non-Destructive Testing like CT scanning) for critical internal structures or porosity checks. Quality assurance testing is the final gate before delivery.
Met3dp understands that post-processing is integral to delivering functional additive manufactured parts. We offer or manage a comprehensive suite of post-processing services, ensuring that the custom heat sinks we produce meet the stringent requirements of industries like aerospace, automotive, and medical, right through to final inspection and delivery. Engaging with a provider who considers the entire workflow, including post-processing, is key to successful project outcomes.
Overcoming Challenges in 3D Printing Heat Sinks: Solutions and Best Practices
While metal additive manufacturing offers tremendous advantages for custom heat sinks, like any advanced manufacturing process, it comes with potential challenges. Awareness of these potential hurdles and understanding the solutions and best practices employed by experienced AM providers like Met3dp is crucial for mitigating risks and ensuring successful outcomes. Addressing these challenges proactively is key to delivering reliable, high-performance components expected by B2B clients.
1. Warping and Residual Stress:
- Desafío: The intense, localized heating and rapid cooling inherent in LPBF/SEBM create thermal gradients that lead to internal stress buildup. If stresses exceed the material’s yield strength at elevated temperatures, or if they are relieved unevenly upon removal from the build plate, the part can warp or distort, compromising dimensional accuracy. Warping metal 3D printing is a primary concern, especially for large or complex parts.
- Solutions & Best Practices:
- Process Simulation: Especializada AM process simulation software predicts stress accumulation and distortion based on geometry, material, and scan strategy, allowing for upfront optimization.
- Optimized Build Orientation: Orienting the part to minimize large flat surfaces parallel to the build plate, reduce thermal mass concentrations, and minimize unsupported overhangs.
- Robust Support Strategy: Using well-designed support structures to anchor the part firmly, conduct heat away, and counteract shrinkage forces.
- Optimized Scan Strategy: Using techniques like island scanning or checkerboard patterns to manage heat distribution and reduce long, continuous melt tracks.
- Mandatory Stress Relief: Performing a proper stress relief heat treatment before removing the part from the build plate is non-negotiable for most metal AM parts, especially aluminum alloys.
2. Support Structure Removal Difficulties:
- Desafío: Supports, while necessary, must be removed. This can be difficult, time-consuming, and risk damaging the part, especially for:
- Internal Supports: Supports inside channels or lattice structures can be nearly impossible to access and remove completely.
- Delicate Features: Removing supports attached to thin fins or intricate details requires great care.
- Tough Materials: Supports made from strong alloys can be harder to break away or machine.
- Solutions & Best Practices:
- DfAM for Support Minimization: The best approach is to design the part to be self-supporting as much as possible (using angles >45°, designing optimal orientation).
- Designing for Access: If internal supports are unavoidable, design access ports or pathways for removal tools or flushing.
- Smart Support Design: Use support types with minimal contact points (e.g., tapered or perforated connections) that break away more easily. Tree supports often offer better accessibility.
- Appropriate Removal Techniques: Utilizing the right tools (manual, EDM, machining) based on support location and material. Experienced technicians are crucial.
3. Porosity Control:
- Desafío: Small voids or pores can sometimes form within the printed material due to trapped gas, incomplete fusion between layers, or powder inconsistencies. Porosity can negatively impact:
- Conductividad térmica: Pores disrupt the heat flow path, lowering the effective conductivity.
- Resistencia mecánica: Acts as stress concentrators, reducing fatigue life and overall strength.
- Leak Tightness: Can create leak paths in liquid-cooled channels.
- Solutions & Best Practices:
- High-Quality Powder: Using powder with high sphericity, controlled particle size distribution, low internal gas content, and proper handling to avoid moisture contamination (Met3dp’s VIGA/PREP powders excel here).
- Optimized Print Parameters: Developing and strictly controlling process parameters (laser/beam power, speed, layer thickness, atmosphere control) validated for specific material/machine combinations to ensure full melting and fusion. Met3dp invests heavily in process optimization.
- Prensado isostático en caliente (HIP): For critical applications demanding near-100% density, HIP post-processing (applying high temperature and isostatic gas pressure) can effectively close internal pores.
- Control de calidad: Utilizing NDT methods like CT scanning to detect and quantify porosity if required by the application. Porosity control AM relies on rigorous process management.
4. Powder Removal from Complex Geometries:
- Desafío: Unmelted powder can get trapped within intricate lattice structure heat sinks or long, narrow internal channels. Complete removal is vital for performance and to prevent loose powder contamination later.
- Solutions & Best Practices:
- DfAM for Depowdering: Designing escape holes, ensuring channel connectivity, avoiding dead ends, and using smooth internal curves.
- Effective Cleaning Methods: Employing appropriate techniques like compressed air/inert gas purging, vibration, ultrasonic cleaning, or specialized solvent/fluid flushing systems.
- Inspection: Verifying powder removal through visual inspection (borescope), weighing the part, or CT scanning if necessary. Powder removal complex parts requires forethought in design.
5. Achieving Consistent Surface Finish:
- Desafío: As-built surface finish varies depending on the surface’s angle relative to the build direction. This inconsistency might be unacceptable for aesthetic or functional reasons (e.g., uniform airflow).
- Solutions & Best Practices:
- Strategic Orientation: Prioritize critical surfaces by orienting them vertically or upwards if possible.
- Consistent Post-Processing: Employing surface finishing techniques like bead blasting or tumbling provides a more uniform finish across all surfaces.
- Acceptance Criteria: Clearly define acceptable surface finish requirements for different part features.
6. Thermal Performance Validation:
- Desafío: Ensuring the final, printed, and post-processed heat sink performs thermally as predicted by simulations or design calculations. Discrepancies can arise from variations in material properties (affected by porosity or heat treatment), geometric deviations, or surface finish impacts.
- Solutions & Best Practices:
- Accurate Material Data: Using validated material property data (including thermal conductivity after specific heat treatments) in simulations.
- Correlated Simulation: Calibrating CFD/FEA models with empirical test data where possible.
- Thermal Testing: Performing laboratory tests (e.g., using thermal test vehicles, infrared thermography) or in-situ testing to measure actual thermal resistance and temperature profiles under operational loads.
- Collaboration: Working closely with a reliable AM supplier like Met3dp, who understands the nuances of AM part performance and can provide guidance on expected outcomes and validation methods. Thermal performance validation closes the loop between design and reality.
By anticipating these challenges and implementing robust solutions grounded in DfAM principles, rigorous process control, comprehensive post-processing, and thorough quality assurance, metal additive manufacturing can reliably deliver custom heat sinks that meet and often exceed the demands of challenging electronic applications. Partnering with an experienced provider like Met3dp significantly de-risks the adoption of this transformative technology for critical thermal management needs. Understanding and troubleshooting metal AM issues is part of the value an expert partner brings.
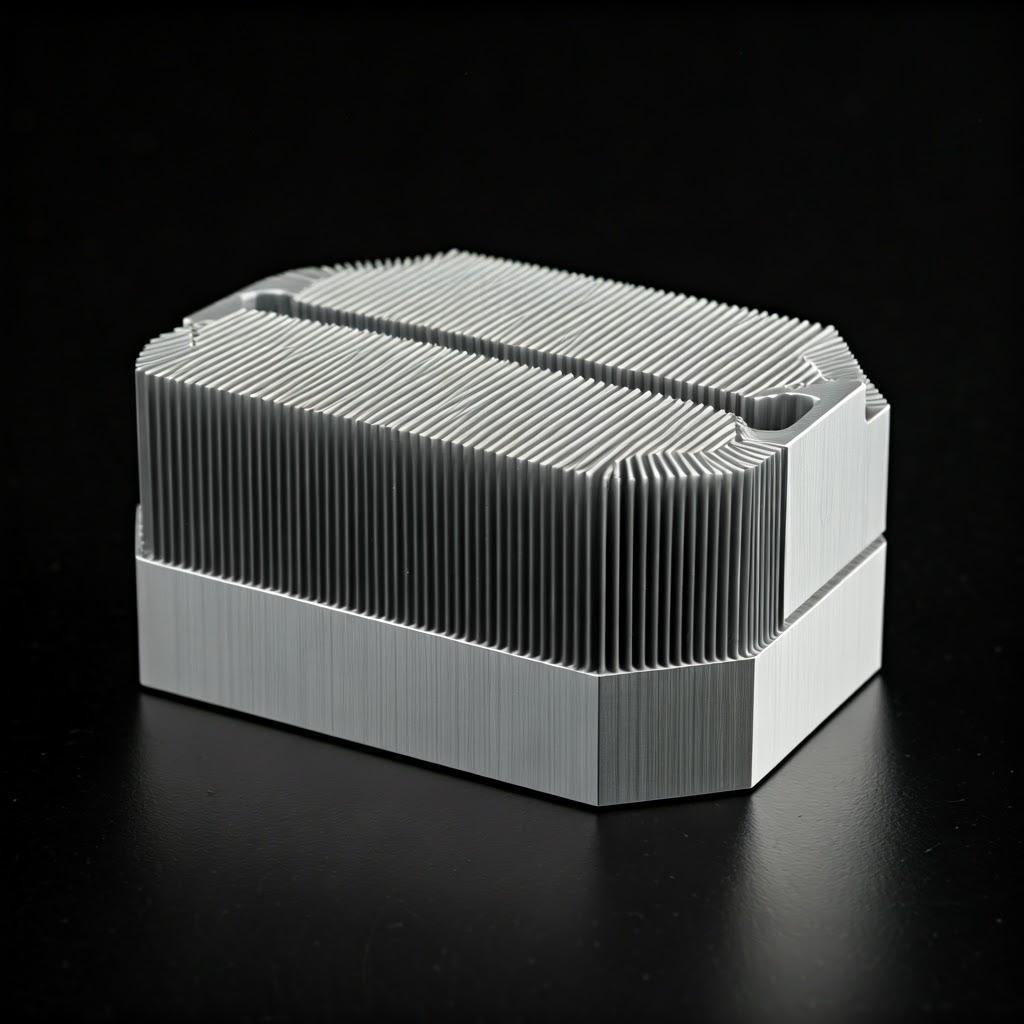
Choosing Your Partner: Selecting the Right Metal 3D Printing Service Provider for Heat Sinks
Embarking on a project involving 3D printed custom heat sinks requires more than just access to a metal printer; it demands a strategic partnership with a service provider possessing the right blend of technology, materials science knowledge, process control, and application-specific expertise. The quality, performance, and reliability of your final thermal management solution are directly linked to the capabilities of the partner you choose. For procurement managers and engineers evaluating potential suppliers, conducting a thorough metal AM service bureau evaluation is critical. Not all providers are created equal, especially when dealing with the complexities of thermal components.
Here are key criteria to consider when selecting your heat sink manufacturing supplier using additive manufacturing:
1. Proven Expertise in Thermal Management & Relevant Materials:
- Look Beyond General Printing: Does the provider have demonstrable experience printing heat sinks or other complex thermal components? Ask for case studies, examples, or data related to thermal applications.
- Material Specialization: Do they routinely process the materials crucial for heat sinks, namely AlSi10Mg and high-conductivity copper alloys like CuCrZr? Understand their level of expertise in optimizing parameters and post-processing for these specific metals to achieve desired thermal and mechanical properties.
- Application Understanding: Do their engineers understand the nuances of heat transfer, fluid dynamics (for liquid cooling), and the specific challenges of electronics cooling in your industry (e.g., aerospace, automotive)?
2. Advanced Technology & Equipment:
- Machine Portfolio: Do they operate state-of-the-art, industrial-grade printers (LPBF, SEBM)? What is the build volume, accuracy, and reliability record of their equipment? Providers like Met3dp invest in industry-leading print volume, accuracy and reliability to handle demanding applications.
- Control de procesos: What measures are in place for process monitoring (e.g., melt pool monitoring, environmental controls)? Consistent process control is key to repeatable quality.
- Software Tools: Do they utilize advanced software for build preparation, simulation (thermal/stress), and support generation?
3. Material Quality, Control, and Traceability:
- Powder Sourcing & Quality: This is paramount. Does the provider manufacture their own high-quality powders using advanced methods like VIGA or PREP (as Met3dp does), or do they have stringent controls for sourcing from qualified suppliers? Ask about powder characterization (sphericity, particle size distribution, purity) and batch consistency.
- Handling & Recycling: What are their procedures for storing, handling, sieving, and recycling metal powders to prevent contamination and ensure consistent feedstock quality?
- Material Traceability: Can they provide full trazabilidad del material from raw powder batch to final part, including certifications? This is often a requirement in regulated industries.
4. Comprehensive Post-Processing Capabilities:
- Integrated Services: Does the provider offer the necessary post-processing steps in-house or through a tightly managed network? This includes stress relief, support removal, heat treatment (with calibrated furnaces for specific cycles like T6 for AlSi10Mg or aging for CuCrZr), CNC machining for critical features, various surface finishing options (heat sink anodizing, polishing, blasting), and thorough cleaning/depowdering.
- Experiencia: Do they have the expertise to perform these steps correctly without damaging the part and achieving the specified properties and finishes?
5. Robust Quality Management System (QMS) & Certifications:
- Base Certification: ISO 9001 certification demonstrates a commitment to quality processes and continuous improvement.
- Industry-Specific Certifications: Depending on your application, certifications like AS9100 (Aerospace), ISO 13485 (Medical), or IATF 16949 (Automotive) may be required. Even if not strictly required, providers holding these certifications often have more rigorous quality control, documentation, and process validation procedures, making them a preferred aerospace certified AM or medical-grade supplier.
6. Engineering & DfAM Support:
- Collaborative Partnership: Does the provider offer Design for Additive Manufacturing (DfAM) consultation? Can their engineers review your design, suggest optimizations for printability, performance, support reduction, and cost-effectiveness? This experiencia en fabricación aditiva is invaluable.
- Simulation Capabilities: Can they assist with thermal or stress simulation to predict performance or identify potential issues before printing?
- Problem Solving: Do they have experienced engineers who can help troubleshoot design or manufacturing challenges?
7. Communication, Transparency, and Project Management:
- Clear Quoting: Is the quoting metal 3D printing process straightforward and transparent, clearly outlining all costs and steps?
- Responsiveness: Is the team responsive to inquiries and proactive in communication throughout the project?
- Project Tracking: Can they provide updates on project status and estimated completion times?
8. Capacity, Lead Times, and Scalability:
- Prototyping Speed: Can they offer quick turnaround times for prototypes?
- Capacidad de producción: Do they have the machine capacity and workflow management to handle potential series production volumes if needed?
- Reliable Lead Times: Do they have a track record of meeting promised delivery dates?
Why Met3dp Excels: Met3dp is purpose-built to meet these demanding criteria. With decades of collective expertise, we offer comprehensive solutions encompassing:
- Advanced AM Systems: Industry-leading SEBM and LPBF printers.
- Polvos de alta calidad: In-house manufacturing using VIGA and PREP technologies for superior AlSi10Mg, CuCrZr, Ti-alloys, and more.
- End-to-End Services: From DfAM consultation and simulation support to printing, full post-processing, and stringent quality assurance.
- Proven Track Record: Successfully serving mission-critical applications in aerospace, medical, automotive, and industrial sectors.
- Learn more about our company, values, and commitment to quality on our Quiénes somos.
Choosing the right partner is an investment in the success of your project. By carefully evaluating potential suppliers against these criteria, you can find a provider like Met3dp that possesses the necessary Met3dp capabilities and acts as a true partner in innovation, helping you leverage the full potential of metal AM for your custom heat sink needs.
Understanding Cost Factors and Lead Times for 3D Printed Heat Sinks
One of the primary considerations for adopting any manufacturing technology is understanding the associated costs and production timelines. Metal additive manufacturing, while offering unique capabilities, has its own set of cost drivers and lead time factors that differ from traditional methods. Providing transparency in metal 3D printing cost estimation y AM production lead time helps engineers and procurement managers make informed decisions and evaluate the overall value proposition.
Key Cost Factors for 3D Printed Heat Sinks:
- Material Type and Consumption:
- Coste del polvo: The raw material cost varies significantly. High-performance copper alloys like CuCrZr are considerably more expensive than aluminum alloys like AlSi10Mg. Exotic alloys would increase costs further.
- Volumen: The total volume of the part plus any necessary support structures directly impacts the amount of powder consumed and fused. Larger, denser parts cost more. DfAM techniques like topology optimization and lattice structures can significantly reduce material usage and thus cost.
- Machine Time (Build Time):
- Primary Driver: Build time is largely determined by the height of the part(s) in the Z-axis (number of layers) and the total area that needs to be scanned by the laser or electron beam for each layer.
- Complejidad: Highly complex geometries might require more intricate scanning paths, slightly increasing time per layer.
- Nesting & Platform Utilization: Printing multiple parts simultaneously (nesting) on a single build platform drastically improves machine utilization and reduces the cost per part. Maximizing the number of parts within the build volume is key for economies of scale AM.
- Costes laborales:
- Pre-Processing: CAD file preparation, orientation optimization, support structure generation, and build file setup require skilled technician time.
- Machine Operation: Setting up the printer, monitoring the build (though often highly automated), and removing the build platform.
- Post-procesamiento: This can be a significant labor component, especially for support removal from complex parts, manual finishing, and inspection.
- Estructuras de apoyo:
- Material Usage: Supports consume material, adding to the cost.
- Removal Effort: The time and effort required to remove supports (manual or machining) directly impact labor costs. Minimizing supports through DfAM is crucial for cost reduction.
- Requisitos de postprocesamiento:
- Tratamiento térmico: Costs associated with furnace time, energy consumption, and handling for stress relief and/or aging cycles.
- Mecanizado: CNC machining time for critical features adds cost based on complexity and time required.
- Acabado superficial: Costs vary depending on the method (blasting, polishing, tumbling, anodizing) and the level of finish required.
- HIP (Hot Isostatic Pressing): If required for maximum density, HIP adds a significant cost component.
- Quality Control & Inspection:
- The level of inspection needed (visual, dimensional checks with CMM/scanning, NDT like CT scanning for internal integrity) impacts labor and equipment costs. Requirements vary based on application criticality.
- Setup & Engineering Fees:
- Some providers may charge initial setup fees, especially for complex projects or if significant DfAM support is required.
Typical Lead Times:
Lead times for 3D printed metal heat sinks can vary widely:
- Prototipos: For single parts or very small batches, lead times can range from a few business days to 2-3 weeks, depending on complexity, material, machine availability, and required post-processing. Expedited services may be available at a premium.
- Producción de bajo volumen: For batches of tens to hundreds of parts, lead times typically range from 3 to 8 weeks, heavily influenced by total machine time required, post-processing complexity, and overall capacity/scheduling.
- Factors Influencing Lead Time:
- Current Order Queue: Service bureau workload is a major factor.
- Tiempo de construcción: As calculated based on part height and volume.
- Post-Processing Complexity: Multi-step or intricate post-processing adds significant time.
- Disponibilidad de material: Ensuring the specific powder is in stock.
- Inspection Requirements: Extensive testing adds time.
Return on Investment (ROI) Considerations:
Mientras que el cost per part analysis for AM might sometimes appear higher than traditional methods for simple geometries in high volumes, the ROI additive manufacturing offers becomes compelling when considering:
- Complexity for Free: AM handles high geometric complexity with little to no added cost compared to machining, where complexity drives cost exponentially.
- No Tooling Costs: Eliminates the significant upfront investment and lead time associated with molds or dies, making it ideal for custom parts and low-to-mid volumes.
- Performance Gains: Superior thermal performance achieved through optimized designs can lead to better product reliability, efficiency, or capability, providing indirect ROI.
- Aligeramiento: Reduced component weight translates to fuel savings (automotive/aerospace) or improved handling.
- Tiempo de comercialización más rápido: Rapid prototyping and quicker transition to production for complex parts can provide a competitive advantage.
- Flexibilidad de la cadena de suministro: On-demand production reduces inventory needs and allows for design updates.
Understanding these cost drivers and lead time expectations allows for accurate budgeting and project planning. For businesses considering wholesale pricing 3D printing or regular production, discussing volume requirements and optimization strategies with a provider like Met3dp is essential for achieving the best possible value. The choice of specific métodos de impresión (like LPBF vs SEBM) might also have subtle cost implications depending on the provider and application, although material and geometry are usually dominant factors.
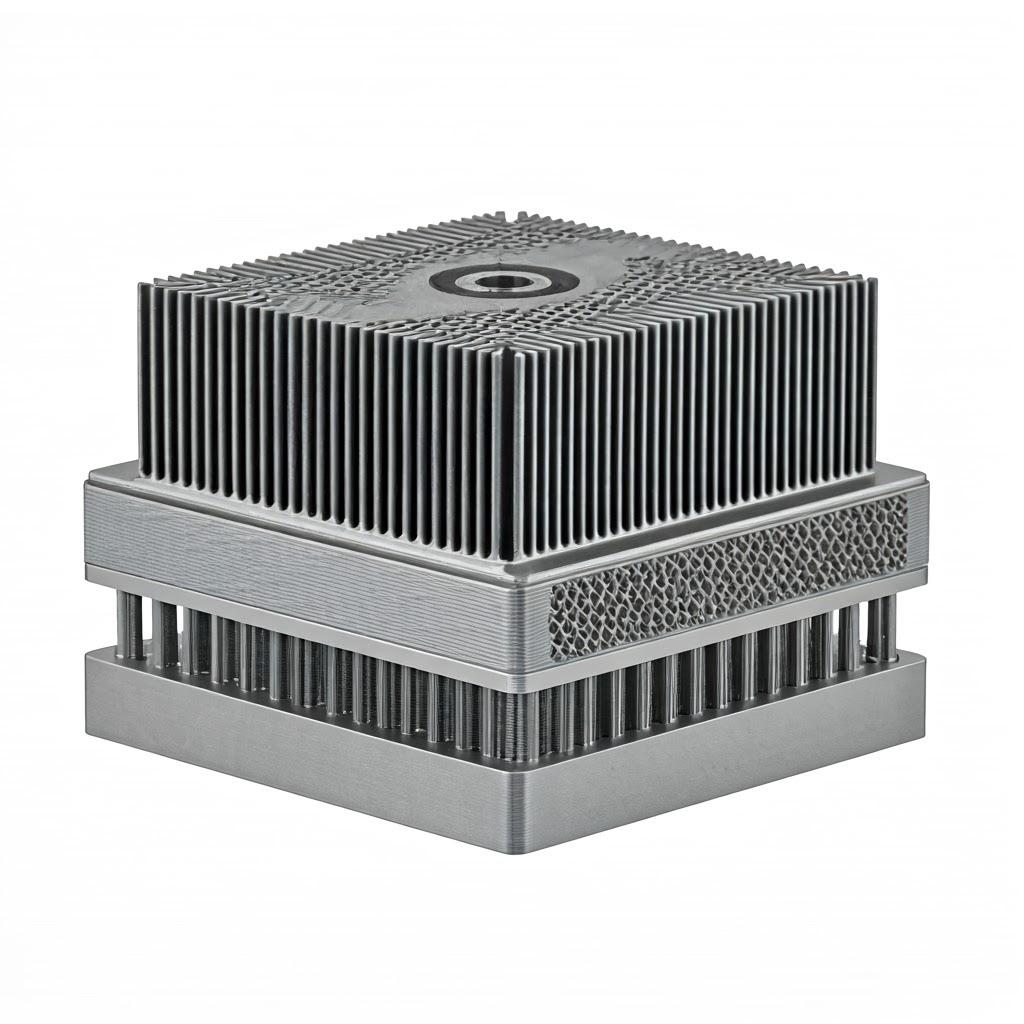
Frequently Asked Questions (FAQ) about 3D Printed Custom Heat Sinks
As metal additive manufacturing becomes more established for producing functional components like heat sinks, engineers, designers, and procurement specialists often have specific questions. Here are answers to some frequently asked questions:
Q1: How does the thermal performance of a 3D printed heat sink compare to a machined one of the same design and material?
A: Assuming the 3D printed part achieves full density (typically >99.5%, often achievable with proper process control and potentially HIP for critical cases) and undergoes appropriate heat treatment to optimize thermal conductivity, its performance should be very similar to a machined counterpart of the exact same geometry. The key advantage of AM, however, is not just replicating traditional designs, but enabling geometrically optimized designs (using lattices, complex channels, topology optimization) that significantly superan a traditional designs constrained by manufacturability. So, while a simple block fin heat sink might perform similarly whether printed or machined, a 3D printed heat sink performance shines when AM-specific designs are utilized to maximize surface area, improve flow paths, or conform perfectly to heat sources.
Q2: What is the typical cost range for a prototype 3D printed metal heat sink?
A: Providing a single cost range is difficult as it’s highly dependent on several factors specific to each project. Key variables include:
- Size & Volume: Larger parts consume more material and machine time.
- Material: CuCrZr is significantly more expensive than AlSi10Mg.
- Complejidad: While AM handles complexity well, very intricate features or extensive support needs can increase post-processing labor.
- Post-procesamiento: Required steps like machining, polishing, or specific coatings add cost.
- Cantidad: A single prototype will have a higher per-part cost than a small batch where setup costs are amortized. Generally, prototype costs can range from several hundred to several thousand dollars (USD or equivalent). The best approach is always to submit a 3D CAD model (e.g., STEP file) and detailed requirements to a provider like Met3dp for an accurate, project-specific quote. This cost comparison AM vs traditional often favors AM for complex prototypes due to the avoidance of tooling.
Q3: What information do I need to provide to get an accurate quote for a 3D printed heat sink?
A: To ensure an accurate and timely quote, please provide the following:
- 3D CAD File: A high-quality 3D model in a standard format (STEP is preferred; STL is also common but lacks some metadata).
- Material Specification: Clearly state the desired alloy (e.g., AlSi10Mg, CuCrZr) or performance requirements.
- Quantity Required: Number of parts needed (for prototype or production run).
- Critical Tolerances & Features: Identify any dimensions or surfaces with specific tolerance requirements tighter than standard AM capabilities (±0.1-0.2mm). Indicate surfaces needing specific roughness (Ra) values.
- Requisitos de postprocesamiento: Specify necessary heat treatments (e.g., T6 for AlSi10Mg), surface finishes (e.g., bead blast, anodize, polish contact surface), machining needs (e.g., tapped holes), and any required testing or certifications.
- Application Context (Optional but helpful): Briefly describing the application can help the provider offer DfAM suggestions or confirm material suitability.
Q4: Can you 3D print heat sinks with integrated heat pipes or vapor chambers?
A: Directly 3D printing functional, sealed heat pipes or vapor chambers complete with working fluid using standard metal AM processes is currently highly experimental and generally not commercially feasible. These devices rely on specific internal structures (wicking structures) and phase-change physics that are difficult to replicate monolithically with AM. However, metal AM plays a crucial role in enabling advanced thermal solutions incorporating these technologies. For example, AM can be used to print:
- Complex outer casings or condensers for heat pipes/vapor chambers, allowing them to be integrated seamlessly into confined spaces or conform to heat sources.
- Heat sinks with precisely designed internal channels where miniature heat pipes can be inserted and bonded during assembly.
- Manifolds and structures for advanced liquid or two-phase cooling systems. AM allows the enclosure y integration aspects of these advanced thermal technologies to be customized and optimized in ways previously impossible.
Q5: What are the typical minimum order quantities (MOQs) for production runs of 3D printed heat sinks?
A: One of the significant advantages of additive manufacturing is its flexibility regarding batch sizes. Unlike traditional methods requiring expensive tooling (like extrusion dies or casting molds), AM has virtually no tooling cost. This means that minimum order quantity AM requirements are often very low or non-existent. It’s economically feasible to print single prototypes, small batches of tens or hundreds, or even scale to thousands of parts. The cost per part generally decreases with larger batches due to better machine utilization (nesting) and amortization of setup/programming costs. This makes AM ideal for customized B2B solutions, niche applications, and industries requiring high-mix, low-volume production. Discuss your volume forecasts with your AM partner to optimize production planning and pricing.
Conclusion: The Future of Electronics Cooling is Customized and Additively Manufactured
The relentless march towards smaller, more powerful, and more integrated electronics presents thermal management challenges that increasingly push traditional manufacturing methods to their limits. As we’ve explored, metal additive manufacturing offers a powerful alternative, enabling a paradigm shift in how we design and produce custom heat sinks and other critical thermal components. The ability to create highly complex geometries, leverage topology optimization for lightweighting and performance, integrate features, and utilize advanced materials like AlSi10Mg and CuCrZr provides engineers with unprecedented tools to solve demanding cooling problems across aerospace, automotive, medical, computing, and industrial sectors.
En benefits of AM heat sinks are clear:
- Libertad de diseño sin igual: Enabling geometries optimized for thermal performance, not just manufacturability.
- Rendimiento mejorado: Through features like lattice structures, optimized fin arrays, and conformal cooling channels.
- Aligeramiento: Critical for applications where mass is a penalty.
- Consolidación de piezas: Reducing assembly complexity and potential failure points.
- Customization & Speed: Ideal for bespoke solutions, rapid prototyping, and on-demand production without tooling constraints.
However, realizing these benefits requires more than just access to a printer. It demands a holistic approach encompassing Design for Additive Manufacturing (DfAM), careful material selection, meticulous process control, comprehensive post-processing, and rigorous quality assurance. Overcoming challenges like residual stress, support removal, and porosity requires expertise and best practices.
Choosing the right partner is paramount. Met3dp stands as a leader in providing advanced manufacturing solutions, combining cutting-edge SEBM and LPBF printing technology with in-house production of high-quality, specialized metal powders and decades of collective expertise in metal AM. Our commitment extends beyond printing to offer end-to-end support, from DfAM consultation to final part validation, ensuring our clients receive functional, reliable, high-performance components. We see ourselves as a key enabler of next-generation cooling tecnologías.
The journey towards optimized thermal management increasingly involves additive manufacturing. Whether you are developing high-power density electronics, seeking lightweight solutions for aerospace, or designing compact medical devices, metal AM offers possibilities that were previously unattainable. The future thermal management landscape will undoubtedly be shaped by the ability to create highly customized, performance-driven components on demand.
We invite you to explore how a Met3dp partnership can elevate your thermal management strategy. If you are facing complex cooling challenges or wish to investigate the potential of 3D printed custom electronics components for your applications, contact Met3dp today. Visit our main website at https://met3dp.com/ to learn more about our capabilities or reach out to our expert team to discuss your specific project requirements. Let us help you unlock the future of electronics cooling – customized, optimized, and additively manufactured.
Compartir
Facebook
Twitter
LinkedIn
WhatsApp
Correo electrónico
MET3DP Technology Co., LTD es un proveedor líder de soluciones de fabricación aditiva con sede en Qingdao, China. Nuestra empresa está especializada en equipos de impresión 3D y polvos metálicos de alto rendimiento para aplicaciones industriales.
Solicite información para obtener el mejor precio y una solución personalizada para su empresa.
Artículos relacionados
Acerca de Met3DP
Actualización reciente
Nuestro producto
CONTACTO
¿Tiene alguna pregunta? ¡Envíenos un mensaje ahora! Atenderemos su solicitud con todo un equipo tras recibir su mensaje.
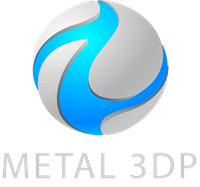
Polvos metálicos para impresión 3D y fabricación aditiva
PRODUCTO
cONTACT INFO
- Ciudad de Qingdao, Shandong, China
- [email protected]
- [email protected]
- +86 19116340731