Sensor Brackets 3D Printed for EVs and Autonomous Vehicles
Índice
The automotive industry is undergoing a seismic shift, driven by the twin revolutions of electrification (EVs) and autonomous driving. These advancements necessitate vehicles packed with sophisticated sensors – LiDAR, RADAR, cameras, ultrasonic – constantly perceiving the environment. Ensuring these sensors function optimally requires robust, precise, and often complex mounting solutions: sensor brackets. Traditionally manufactured through methods like CNC machining, casting, or stamping, these brackets face increasing challenges related to design complexity, weight optimization, and rapid development cycles demanded by the modern automotive landscape. This is where metal additive manufacturing (AM), commonly known as metal Impresión 3D, emerges as a transformative technology.
Metal 3D printing offers unparalleled design freedom, enabling the creation of intricate, topology-optimized, and lightweight sensor brackets that are simply not feasible with conventional methods. For Electric Vehicles (EVs), lightweighting is paramount to extend range, and AM delivers components with exceptional strength-to-weight ratios. In the realm of Autonomous Vehicles (AVs), the sheer number and variety of sensors, each requiring precise positioning and stability, create complex packaging challenges that AM can elegantly solve through part consolidation and customized geometries. This technology allows automotive engineers and procurement managers to move from concept to functional prototype to series production faster than ever before, accelerating innovation cycles and gaining a competitive edge.
This comprehensive guide delves into the specifics of using metal 3D printing for automotive sensor brackets, focusing on applications within the rapidly evolving EV and autonomous vehicle sectors. We will explore the functional requirements, the distinct advantages AM offers over traditional manufacturing, suitable high-performance materials like Aluminum alloys (specifically AlSi10Mg and A7075), critical design considerations (DfAM), achievable precision levels, necessary post-processing steps, potential challenges, and guidance on selecting the right manufacturing partner. Whether you are an engineer designing next-generation ADAS systems, a procurement manager sourcing reliable automotive component suppliers, or a production specialist exploring advanced manufacturing techniques, understanding the capabilities of metal AM for sensor brackets is crucial for navigating the future of automotive engineering. Companies like Met3dp, specializing in advanced metal powders and printing systems, are at the forefront, enabling this transition towards more efficient, customized, and high-performance automotive components.
Critical Functions: What Are Automotive Sensor Brackets Used For?
Automotive sensor brackets might seem like simple components, but they play a mission-critical role in the functionality and safety of modern vehicles, particularly EVs and those equipped with Advanced Driver-Assistance Systems (ADAS) and full autonomy features. Their primary purpose is to securely mount, precisely position, protect, and sometimes thermally manage the diverse array of sensors integrated throughout the vehicle. The reliability and accuracy of the data captured by these sensors are directly dependent on the stability and correct orientation provided by their brackets.
Key Functions and Applications:
- Secure Mounting: The most fundamental role is to firmly attach sensors to the vehicle’s chassis, bodywork, bumper, grille, roofline, or interior structures. The bracket must withstand constant vibrations, shocks, and environmental stresses encountered during vehicle operation without loosening or failing. Failure could lead to sensor misalignment, malfunction, or complete detachment.
- Precise Positioning and Alignment: ADAS and autonomous driving systems rely on sensors having a precise, fixed field of view. LiDAR scanners need stable platforms for accurate point cloud generation, RADAR units require specific angles for target detection and tracking, and cameras need exact alignment for image processing, lane-keeping, and object recognition. Sensor brackets are designed to maintain these critical orientations over the vehicle’s lifetime, often incorporating features for fine-tuning alignment during assembly or service. Even minor misalignments can drastically impair system performance and safety.
- Environmental Protection: Sensors, especially those mounted externally, are exposed to harsh conditions: moisture, dirt, debris, stone chips, temperature extremes, and cleaning chemicals. Brackets often form part of the sensor’s enclosure or work in conjunction with other protective elements to shield sensitive electronics and optics from damage or contamination. The material choice (like corrosion-resistant aluminum alloys) and design contribute significantly to this protective function.
- Amortiguación de vibraciones: Vehicle operation generates vibrations that can negatively impact sensor performance and longevity. Sensor brackets can be designed, sometimes incorporating specific geometries or materials, to dampen or isolate the sensor from harmful frequencies, ensuring clearer signals and reduced mechanical stress.
- Gestión térmica: Some sensors generate heat during operation, or are mounted near heat sources like powertrains or electronics. Brackets made from thermally conductive materials, such as aluminum alloys, can help dissipate heat away from the sensor, preventing overheating and ensuring stable operation within the specified temperature range. This is particularly relevant in densely packed EV electronic architectures.
- Integration and Packaging: In modern vehicles, space is at a premium. Sensor brackets facilitate the integration of sensors into complex assemblies, often navigating tight spaces and accommodating wiring harnesses and connectors. Metal AM allows for highly customized shapes that fit perfectly within the available design envelope, potentially consolidating multiple mounting points or features into a single printed part.
Specific Sensor Types and Bracket Requirements:
- LiDAR (Light Detection and Ranging): Often requires large, stable brackets capable of handling the sensor’s weight and maintaining precise angular orientation for 360-degree scanning. Vibration damping is critical for point cloud accuracy. Brackets may be mounted on the roof, grille, or fenders.
- RADAR (Radio Detection and Ranging): Needs brackets that ensure correct aiming (azimuth and elevation) and are often integrated behind grilles or bumpers. The bracket material must not interfere with radio wave transmission (though this is less of a concern for metal brackets mounted behind non-metallic fascias). Stability is key for adaptive cruise control and collision avoidance.
- Cameras (Visible, Infrared): Require extremely stable mounting to prevent image jitter, which affects lane departure warning, traffic sign recognition, and autonomous navigation. Brackets are often located behind the windshield, in side mirrors, grilles, or bumpers, needing precise alignment.
- Ultrasonic Sensors: Typically used for parking assistance and short-range object detection, these sensors are often mounted in bumpers. Brackets need to hold them securely and flush with the bumper surface, protecting them from minor impacts while allowing acoustic waves to propagate effectively.
- Inertial Measurement Units (IMUs): These sensors (gyroscopes, accelerometers) are critical for vehicle dynamics and autonomous navigation. Their brackets must provide a rigid, vibration-isolated connection to the vehicle chassis for accurate motion sensing.
The increasing complexity and number of sensors in EVs and AVs drive the need for more sophisticated, lightweight, and custom-designed brackets. Procurement managers looking for reliable sensor mounting solutions and wholesale automotive parts suppliers must recognize the growing demand for brackets that meet these advanced requirements, often best addressed by technologies like metal 3D printing.
Why Choose Metal 3D Printing for Sensor Bracket Production?
While traditional manufacturing methods like CNC machining, sheet metal fabrication (stamping and bending), and casting have long been used for producing automotive brackets, metal additive manufacturing presents a compelling suite of advantages, particularly for the complex, customized, and performance-driven sensor brackets required in EVs and autonomous vehicles. Opting for metal AM can lead to significant improvements in design, performance, production speed, and supply chain efficiency.
Key Advantages of Metal AM for Sensor Brackets:
- Unmatched Design Freedom & Complexity:
- Optimización de la topología: Algorithms can redesign brackets based on load paths, removing unnecessary material while maintaining structural integrity. This results in highly organic, lightweight shapes impossible to machine or cast economically. For sensor brackets, this means minimal weight addition to the vehicle (crucial for EV range) without compromising rigidity.
- Consolidación de piezas: Multiple components of a bracket assembly (e.g., mount, arm, fastener features) can potentially be redesigned and printed as a single, complex part. This reduces part count, assembly time, potential points of failure, and overall weight.
- Internal Channels & Complex Geometries: AM can create brackets with internal cooling channels (for sensors generating heat), integrated wiring pathways, or intricate lattice structures for optimal stiffness-to-weight ratios. These features are difficult or impossible with subtractive (machining) or formative (casting, stamping) methods.
- Creación rápida de prototipos e iteración:
- Velocidad: Functional metal prototypes of sensor brackets can be produced in days rather than the weeks or months often required for tooling setup in casting or complex CNC programming.
- Flexibilidad: Designs can be quickly modified and reprinted based on testing feedback without incurring significant re-tooling costs. This accelerates the development cycle for new sensor integrations in EVs and AVs, allowing engineers to iterate designs rapidly for optimal sensor placement and performance.
- Aligeramiento:
- As mentioned, topology optimization and the use of inherently strong but light materials like aluminum alloys (AlSi10Mg, A7075) allow for significant weight reduction compared to traditionally designed and manufactured brackets. Every gram saved contributes to better vehicle efficiency, handling, and, in the case of EVs, extended driving range.
- Customization and Low-Volume Production:
- Fabricación a la carta: AM is ideal for producing brackets tailored to specific vehicle models, sensor types, or mounting locations without the need for dedicated tooling. This is beneficial for niche vehicle platforms, aftermarket solutions, or accommodating variations in sensor hardware.
- Economical Small Batches: Producing small to medium volumes (tens to thousands) of brackets via AM can be more cost-effective than traditional methods, which often rely on economies of scale associated with high tooling costs. This aligns well with the often-lower initial volumes for specialized EV models or autonomous test fleets.
- Rendimiento del material:
- Metal AM utilizes high-performance engineering materials, including the recommended AlSi10Mg and A7075 aluminum alloys, which offer excellent strength, durability, corrosion resistance, and thermal properties suitable for demanding automotive environments. Companies specializing in impresión 3D en metal, like Met3dp, provide access to a wide range of certified powders optimized for these applications.
- Supply Chain Advantages for B2B:
- Plazos de entrega reducidos: Compared to sourcing complex machined parts or waiting for casting tooling, AM can drastically shorten lead times for both prototypes and production parts, improving responsiveness for automotive OEMs and Tier 1 suppliers.
- Decentralized Production: Digital design files can be sent electronically to certified AM service providers globally, enabling localized production closer to assembly lines, reducing shipping costs and logistical complexities.
- Reduced Tooling Dependency: Eliminating the need for expensive and time-consuming tooling mitigates supply chain risks associated with tool damage, maintenance, or supplier lock-in. On-demand printing provides greater flexibility.
Comparison Table: Metal AM vs. Traditional Methods for Sensor Brackets
Característica | Metal AM (e.g., PBF) | Mecanizado CNC | Casting (e.g., Die Casting) | Sheet Metal Stamping/Bending |
---|---|---|---|---|
Complejidad del diseño | Very High (Internal channels, lattices) | High (Limited by tool access) | Moderate (Draft angles req.) | Bajo a moderado |
Aligeramiento | Excellent (Topology optimization) | Good (Material removal) | Fair (Minimum wall thickness) | Fair (Material choice) |
Consolidación de piezas | High Potential | Bajo | Moderado | Bajo |
Velocidad de creación de prototipos | Very Fast (Days) | Moderate (Days/Weeks) | Slow (Weeks/Months – tooling) | Moderate (Tooling dependent) |
Coste de utillaje | Ninguno | Low (Fixtures may be needed) | High (Dies) | High (Dies) |
Low Volume Economics | Bien | Moderado | Pobre | Pobre |
Residuos materiales | Low (Powder reuse) | High (Subtractive) | Moderate (Gates/runners) | Moderate (Offcuts) |
Opciones de material | Growing Range (Al, Ti, Steel, etc.) | Wide Range | Good Range (Al, Zn, Mg) | Wide Range (Sheet form) |
Lead Time (Production) | Fast to Moderate | Moderado | Moderate to Slow | Moderado |
Exportar a hojas
While traditional methods remain suitable for very high-volume production of simple bracket designs where cost per part is the absolute primary driver, metal AM offers a compelling value proposition for the increasingly complex, performance-critical, and rapidly evolving sensor brackets integral to modern automotive technology, particularly for wholesale buyers, Tier 1 suppliers, and OEMs focused on innovation in the EV and autonomous vehicle space.
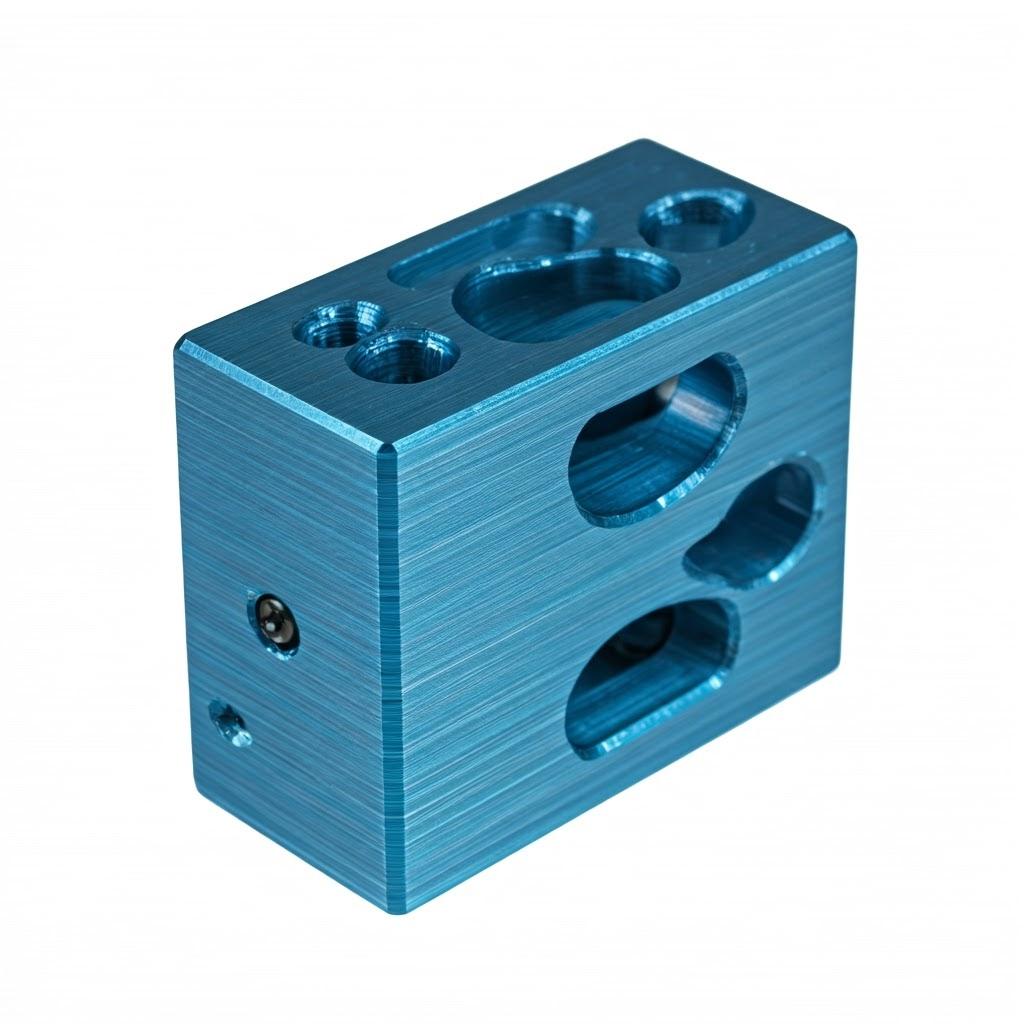
Material Selection Spotlight: AlSi10Mg and A7075 Aluminum Alloys
Choosing the right material is fundamental to the performance, durability, and cost-effectiveness of any manufactured component, and 3D printed automotive sensor brackets are no exception. For applications in EVs and autonomous vehicles, materials must often balance strength, low weight, corrosion resistance, thermal conductivity, and processability via additive manufacturing. Aluminum alloys are frequently favored for these applications, with AlSi10Mg and A7075 emerging as particularly relevant choices for metal AM. Understanding their distinct properties is key for engineers and procurement specialists making material decisions.
AlSi10Mg: The Versatile Workhorse
AlSi10Mg is one of the most common and well-characterized aluminum alloys used in metal powder bed fusion (PBF) processes like Selective Laser Melting (SLM) and Direct Metal Laser Sintering (DMLS). It’s essentially an aluminum casting alloy adapted for additive manufacturing.
- Composición: Primarily aluminum (Al), with significant amounts of Silicon (Si, approx. 9-11%) and Magnesium (Mg, approx. 0.2-0.45%). Silicon improves fluidity and castability (beneficial for the melting/solidification in AM), while Magnesium enables precipitation hardening through heat treatment.
- Propiedades clave:
- Buena relación resistencia-peso: Offers respectable mechanical strength, particularly after appropriate heat treatment, combined with the inherent low density of aluminum. Ideal for lightweighting initiatives in EVs.
- Excelente resistencia a la corrosión: Forms a passive oxide layer, providing good protection against atmospheric corrosion, crucial for brackets exposed to the elements.
- Good Thermal Conductivity: Helps dissipate heat away from sensors or surrounding electronics, contributing to thermal management strategies.
- Excellent Processability in AM: Widely available as powder, with well-established printing parameters leading to relatively high reliability and repeatability in PBF systems. It generally exhibits good weldability during the layer-by-layer melting process.
- Opciones de postprocesado: Responds well to standard post-processing steps like stress relief, T6 heat treatment (solutionizing and artificial aging) to significantly enhance mechanical properties (yield strength, tensile strength, hardness), machining, and surface finishing.
- Typical Applications for Sensor Brackets: Suitable for a wide range of brackets where a good balance of strength, weight, cost, and environmental resistance is required. Often used for brackets supporting cameras, ultrasonic sensors, and certain RADAR units where extreme strength is not the primary driver.
A7075 (Aluminum Alloy 7075): The High-Strength Contender
A7075 is a high-performance aluminum alloy known for its exceptional strength, comparable to many steels, but at a significantly lower density. Traditionally used in aerospace and high-stress structural applications, its adaptation for additive manufacturing is more recent and challenging than AlSi10Mg, but offers significant performance advantages. Zinc (Zn) is the primary alloying element, along with Magnesium (Mg) and Copper (Cu).
- Propiedades clave:
- Very High Strength-to-Weight Ratio: Significantly higher yield and tensile strength than AlSi10Mg, especially after heat treatment (e.g., T6 condition). This allows for even lighter bracket designs for the same load-bearing capacity or creating brackets for highly stressed applications.
- Excellent Fatigue Strength: Resists failure under cyclic loading, important for components subjected to constant vehicle vibration.
- Buena maquinabilidad: Although strong, it can be machined well in its heat-treated state for critical tolerance features.
- Challenges in AM Processability: A7075 is notoriously difficult to process reliably via laser-based PBF due to its wide freezing range and susceptibility to solidification cracking (hot cracking) and porosity. It requires carefully controlled process parameters, specialized machine capabilities, and often modified alloy compositions or processing strategies (e.g., using specific laser parameters or heated build platforms). Electron Beam Melting (EBM) or newer laser techniques might offer advantages.
- Resistencia a la corrosión: Generally lower general corrosion resistance compared to AlSi10Mg, particularly susceptible to stress corrosion cracking (SCC) in certain environments if not properly heat treated and protected (e.g., via anodizing or painting). Careful design and post-processing are essential.
- Typical Applications for Sensor Brackets: Best suited for highly loaded or safety-critical sensor brackets where maximum strength and stiffness are paramount, and weight saving is critical. Examples include brackets for heavy LiDAR units, structural mounting points for sensor clusters, or components subjected to significant dynamic loads, provided the AM processing challenges can be reliably overcome.
Material Comparison Table:
Propiedad | AlSi10Mg | A7075 (AM Processed) | Significance for Sensor Brackets |
---|---|---|---|
Elementos de aleación primarios | Si, Mg | Zn, Mg, Cu | Influences base properties and heat treatment response. |
Densidad | ~2,67 g/cm³ | ~2.81 g/cm³ | Both are lightweight; A7075 slightly denser but much stronger. |
Typical Yield Strength (T6) | ~230-280 MPa | ~450-500+ MPa | A7075 offers significantly higher strength for demanding loads. |
Typical Tensile Strength (T6) | ~300-350 MPa | ~520-570+ MPa | Higher tensile strength indicates better resistance to breaking under tension. |
Módulo elástico | ~70 GPa | ~71 GPa | Similar stiffness; design dictates rigidity more than material here. |
Resistencia a la corrosión | Good to Excellent | Fair (Susceptible to SCC if untreated) | AlSi10Mg better for general exposure; A7075 needs protection. |
Conductividad térmica | Good (~130-150 W/m·K) | Good (~130 W/m·K) | Both suitable for moderate heat dissipation needs. |
AM Processability (L-PBF) | Excellent / Well-Established | Challenging (Requires expertise/control) | AlSi10Mg is easier and more reliable to print currently. |
Coste relativo | Baja | Higher (Powder & Processing) | A7075 carries a premium due to difficulty and performance. |
Exportar a hojas
Leveraging High-Quality Powders:
The final quality and performance of the 3D printed sensor bracket heavily depend on the quality of the metal powder used. Sourcing powders with controlled particle size distribution (PSD), high sphericity, good flowability, and low oxygen/impurity content is crucial for achieving dense, defect-free parts with consistent mechanical properties.
Empresas como Met3dp specialize in producing high-performance metal powders for additive manufacturing using advanced techniques like Vacuum Induction Melting Gas Atomization (VIGA) and Plasma Rotating Electrode Process (PREP). Their expertise ensures powders like AlSi10Mg and potentially specialized variants suitable for high-strength applications meet the stringent requirements of the automotive industry. When selecting an AM service provider or procuring powders directly, verifying the powder quality, certification, and traceability is essential for reliable production of critical components like sensor brackets. Partnering with suppliers who demonstrate rigorous quality control over their advanced powder making systems ensures the foundation for a successful AM component.
In summary, both AlSi10Mg and A7075 offer compelling characteristics for 3D printed automotive sensor brackets. AlSi10Mg provides a robust, cost-effective, and easily processable solution for many applications. A7075 delivers superior strength for the most demanding scenarios, albeit with greater processing complexity and cost. The optimal choice depends on the specific performance requirements, load conditions, operating environment, and manufacturing feasibility for the target sensor bracket application in the EV and autonomous vehicle context. Sources and related content
Design for Additive Manufacturing (DfAM): Optimizing Sensor Brackets for 3D Printing
Simply taking a sensor bracket design intended for CNC machining or casting and sending it to a metal 3D printer rarely yields optimal results. To truly unlock the benefits of additive manufacturing – lightweighting, part consolidation, enhanced performance, and cost efficiency – engineers must embrace Design for Additive Manufacturing (DfAM). DfAM is a philosophy and set of methodologies focused on designing components specifically for the unique capabilities and constraints of AM processes, such as Powder Bed Fusion (PBF). For automotive sensor brackets, applying DfAM principles is critical for success, especially when targeting demanding applications in EVs and autonomous vehicles.
The DfAM Mindset: Thinking Additively
Instead of thinking subtractively (removing material) or formatively (shaping material in a mold), DfAM encourages engineers to think layer-by-layer. This involves considering factors like build orientation, support structure requirements, thermal management during the build, and the potential for creating complex internal and external features that were previously impossible. The goal is not just to replicate an existing bracket but to reimagine it for superior performance and manufacturability via AM.
Key DfAM Principles for Metal AM Sensor Brackets:
- Build Orientation Strategy:
- Impacto: How a part is oriented on the build platform significantly affects surface finish (up-facing vs. down-facing surfaces), the amount and location of required support structures, potential anisotropy (directional variation in mechanical properties), residual stress accumulation, and overall build time and cost.
- Considerations for Brackets: Orienting critical mounting surfaces or features that require high precision upwards can minimize stair-stepping effects and reduce the need for post-machining. However, this might increase the need for supports elsewhere. Minimizing the part’s height (Z-axis) generally reduces print time. Orienting to reduce large cross-sectional areas per layer can mitigate thermal stress. Often, a compromise is needed, balancing surface quality, support needs, and mechanical performance.
- Support Structure Minimization and Design:
- Necesidad: Metal PBF requires support structures for overhangs and bridges that exceed a certain self-supporting angle (typically around 45 degrees for aluminum alloys, though process-dependent). Supports anchor the part to the build plate, prevent warping, and conduct heat away from overhangs.
- DfAM Approach: The primary goal is to minimize the necesita for supports by designing features with self-supporting angles wherever possible. Where supports are unavoidable, they should be designed for:
- Easy Removal: Incorporating breakaway points, using lower-density support structures, ensuring tool access for removal.
- Minimal Surface Scarring: Optimizing the contact points between the support and the part.
- Effective Heat Transfer: Ensuring supports adequately prevent overheating and distortion of overhangs.
- Bracket Example: Instead of a sharp 90-degree overhang for a mounting flange, design it with a 45-degree chamfer or fillet. Round or teardrop shapes for horizontal holes can often make them self-supporting.
- Topology Optimization and Generative Design:
- Concept: These are powerful computational tools that optimize material distribution within a defined design space, subject to load conditions and constraints. Topology optimization removes material from low-stress areas, leaving behind an often organic-looking, load-bearing structure. Generative design explores multiple design solutions based on performance criteria.
- Application to Brackets: Ideal for lightweighting sensor brackets while maintaining or even increasing stiffness. Engineers define mounting points, keep-out zones (where the sensor sits or wires pass), and applied loads (vibration, static weight, shock). The software then generates an optimized bracket geometry, often achieving weight reductions of 30-70% compared to traditional designs. This is hugely beneficial for EV range and overall vehicle dynamics.
- Estructuras reticulares:
- Definición: Engineered porous structures composed of repeating unit cells. They can be integrated into solid part designs.
- Benefits for Brackets:
- Extreme Lightweighting: Filling less critical volumes of a bracket with a lattice structure significantly reduces weight and material consumption.
- Amortiguación de vibraciones: Certain lattice geometries can be designed to absorb or damp vibrations, potentially improving sensor signal quality and longevity.
- Rigidez a medida: Allows for tuning the bracket’s stiffness in different directions.
- Desafíos: Can be difficult to remove powder from complex internal lattices; require careful simulation to ensure structural integrity.
- Espesor de la pared y tamaño de las características:
- Minimums: AM processes have limitations on the minimum wall thickness and feature size they can reliably produce (often around 0.4-0.8 mm, machine and parameter dependent). Designing below these limits can lead to incomplete features or failure.
- Optimization: While thin walls save weight, they can be prone to warping or damage. DfAM involves finding the right balance – using thicker sections where stress is high and thinner sections elsewhere, often guided by topology optimization results. Smooth transitions between thick and thin sections are recommended to reduce stress concentrations.
- Hole Design:
- Small Holes: Very small vertical holes can sometimes self-close due to melt pool dynamics. Minimum recommended diameters are often around 0.5-1.0 mm.
- Horizontal Holes: Prone to drooping or requiring internal supports. Designing them with a teardrop or diamond shape allows the top portion to be self-supporting.
- Threaded Holes: While threads can sometimes be printed directly, they often lack the required precision and strength. The standard DfAM practice is to print pilot holes and then tap them during post-processing (machining).
- Consolidación de piezas:
- Goal: Reduce the number of components in an assembly by redesigning them into a single, integrated AM part.
- Sensor Bracket Example: Instead of a bracket made from multiple stamped, machined, or cast pieces bolted together, DfAM might enable a single printed part incorporating the sensor mount, chassis attachment points, and perhaps even features for cable management or heat dissipation. This reduces assembly labor, inventory, weight, and potential failure points.
- Thermal Management Considerations During Design:
- Desafío: Large, flat surfaces printed parallel to the build plate, or abrupt changes in cross-sectional area, can lead to excessive heat buildup and residual stress, causing warping or cracking.
- DfAM Solutions: Avoid large flat bases where possible, use ribs or corrugations to stiffen thin sections, ensure smooth transitions in geometry, and orient the part strategically (as discussed in point 1). Simulation tools can predict thermal behavior and guide design adjustments.
Collaboration and Tools:
Effective DfAM often requires specialized software tools (e.g., nTopology, Altair Inspire, Autodesk Fusion 360 generative design) and, crucially, collaboration between the design engineer and the AM production specialists or service provider. An experienced AM partner, like Met3dp, can provide invaluable feedback on design manufacturability, suggest optimizations based on their specific métodos de impresión and machine capabilities, and help avoid potential pitfalls. Engaging with the AM provider early in the design process is highly recommended for complex components like sensor brackets. Procurement managers should look for suppliers who offer DfAM support as part of their service.
By applying these DfAM principles, automotive companies can leverage metal AM to produce sensor brackets that are lighter, stronger, more functional, and faster to develop, gaining a significant advantage in the competitive EV and autonomous vehicle markets.
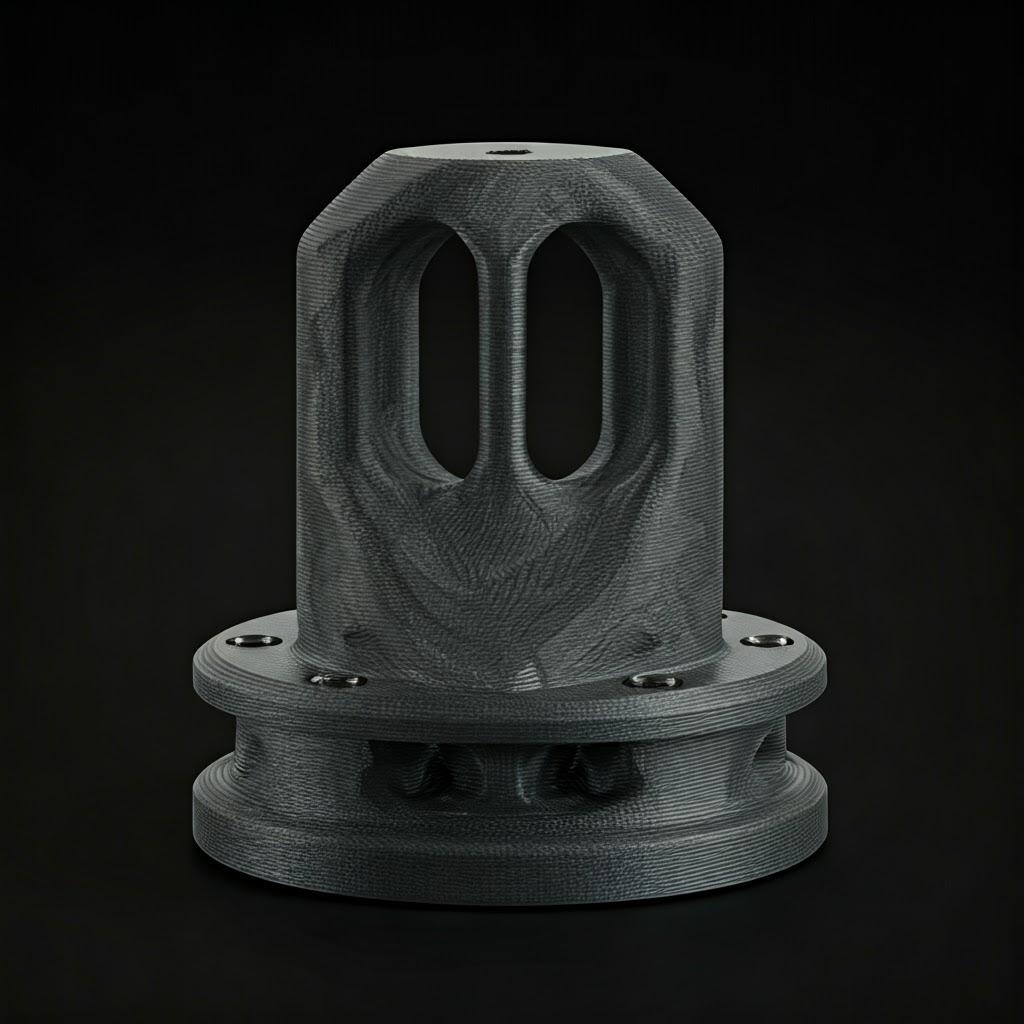
Achieving Precision: Tolerance, Surface Finish, and Dimensional Accuracy in AM Brackets
While metal additive manufacturing offers incredible design freedom, a common question from engineers and procurement managers concerns the level of precision achievable. Can 3D printed metal sensor brackets meet the tight tolerances and surface finish requirements often needed for automotive components, particularly for accurate sensor alignment and interfacing with other parts? Understanding the concepts of tolerance, surface finish, and dimensional accuracy, along with the factors influencing them in metal AM, is crucial.
Defining the Terms:
- Precisión dimensional: How closely the dimensions of the printed part match the nominal dimensions specified in the CAD model. It’s often expressed as a percentage deviation (e.g., ±0.1%) or an absolute deviation (e.g., ±0.1 mm).
- Tolerance: The permissible range of variation for a specific dimension. Engineering drawings define tolerances for critical features (e.g., mounting hole diameters, interface surface flatness) to ensure proper fit and function.
- Surface Finish (or Surface Roughness): Measures the texture and fine-scale irregularities of a part’s surface. Commonly quantified using parameters like Ra (arithmetic average roughness) or Rz (average maximum height of the profile). Lower Ra/Rz values indicate smoother surfaces.
Typical Precision Capabilities of Metal PBF (L-PBF):
For aluminum alloys like AlSi10Mg and A7075 processed using Laser Powder Bed Fusion (L-PBF), typical achievable values are generally in the following ranges:
- Precisión dimensional:
- General Accuracy: Often cited as ±0.1% to ±0.2% of the nominal dimension.
- Minimum Accuracy: For small features, an absolute minimum deviation of around ±0.05 mm to ±0.2 mm is often achievable, depending heavily on the feature, orientation, and machine calibration.
- Tolerances:
- As-Built: Tolerances achievable directly from the printer are typically in the range of ISO 2768-m (medium) or sometimes -f (fine) for certain features. Expect tolerances around ±0.1 mm to ±0.5 mm depending on the size and geometry.
- Post-Machined: For critical features requiring tighter tolerances (e.g., bearing fits, precise mounting faces), post-process CNC machining is standard practice. Machining can easily achieve tolerances common in automotive applications (e.g., ±0.01 mm to ±0.05 mm).
- Surface Finish (Ra):
- As-Built:
- Up-facing / Vertical Walls: Typically range from 6 µm to 15 µm Ra.
- Down-facing / Overhang Surfaces (supported): Generally rougher due to support contact points and partial sintering, often ranging from 15 µm to 25 µm Ra or more.
- Side Walls (relative to recoater): Can show layer lines.
- After Post-Processing:
- Bead Blasting: Can provide a uniform matte finish, typically 5-10 µm Ra, but doesn’t significantly smooth the surface microscopically.
- Tumbling/Acabado en masa: Can improve Ra, especially on external surfaces and edges, potentially reaching below 5 µm Ra.
- Machining/Polishing: Can achieve very smooth surfaces, well below 1 µm Ra if required.
- As-Built:
Factors Influencing Precision:
Achieving consistent accuracy and surface finish in metal AM is a complex interplay of various factors:
- AM System:
- Calibración de la máquina: Regular calibration of lasers, scanners (galvanometers), and motion systems is crucial.
- Machine Condition: Wear and tear, optics cleanliness, and build chamber environment (gas flow, oxygen levels) impact consistency.
- Technology Type: Different AM processes (L-PBF, EBM, Binder Jetting) have inherently different precision characteristics.
- Parámetros del proceso:
- Grosor de la capa: Thinner layers generally allow for finer features and better surface finish on sloped surfaces but increase build time.
- Laser Power, Scan Speed, Hatch Spacing: These core parameters influence melt pool size and stability, affecting density, surface texture, and residual stress (which impacts distortion). Optimized parameter sets are key.
- Estrategia de escaneo: How the laser scans each layer affects heat distribution and stress.
- Propiedades del material:
- Calidad del polvo: Consistent particle size distribution, sphericity, and flowability lead to uniform powder layers and predictable melting. Low-quality powder can cause defects affecting dimensions and finish.
- Propiedades térmicas: Coefficient of thermal expansion and conductivity influence shrinkage and warping during the heating/cooling cycles.
- Part Design (DfAM):
- Geometry: Complex shapes, thin walls, large flat areas, and sharp transitions can be more prone to distortion.
- Orientación: As discussed previously, orientation significantly impacts surface finish on different faces and the potential for warping.
- Estructuras de apoyo:
- Strategy: Density, contact points, and location influence part stability during the build and surface quality after removal. Inadequate support leads to distortion; overly dense supports are hard to remove and leave more significant witness marks.
- Post-procesamiento:
- Alivio del estrés: Essential for minimizing distortion after removal from the build plate. Inconsistent heat treatment can itself cause warping.
- Retirada del soporte: Careless removal can damage part surfaces.
- Mecanizado: Introduces its own set of tolerances but allows for achieving high precision on specific features.
Specification and Verification:
- Clear Drawings: It’s vital for engineers to clearly specify critical dimensions and tolerances on engineering drawings using Geometric Dimensioning and Tolerancing (GD&T). Indicate which tolerances are required “as-built” and which will be achieved via post-machining.
- Quality Agreements: For B2B supply chains, clear quality agreements between the customer and the AM service provider are essential. These should define acceptable tolerance ranges, surface finish requirements, inspection methods, and reporting standards.
- Metrology: Verification of dimensions and tolerances typically requires advanced metrology equipment:
- Coordinate Measuring Machines (CMMs): High accuracy point measurements for critical features.
- 3D Scanners (Laser or Structured Light): Capture the overall geometry of complex shapes for comparison against the CAD model.
- Perfilómetros de superficie: Measure surface roughness parameters like Ra and Rz.
In conclusion, while metal AM might not match the “out-of-the-box” precision of high-end CNC machining across all features, it can achieve accuracy suitable for many automotive applications, including sensor brackets, directly from the printer. By combining good DfAM practices, careful process control, and targeted post-processing (especially machining for critical interfaces), manufacturers can reliably meet stringent automotive tolerance and surface finish requirements. Procurement managers should engage with potential AM suppliers to understand their specific capabilities, quality control processes, and typical achievable precision for materials like AlSi10Mg and A7075.
Beyond the Print Bed: Essential Post-Processing for Metal Sensor Brackets
A common misconception about metal 3D printing is that parts come out of the machine ready to use. In reality, for functional engineering components like automotive sensor brackets made from AlSi10Mg or A7075, the printing process is just the first step. A series of essential post-processing operations are required to transform the raw printed part into a finished component that meets performance, durability, and safety standards. Understanding these steps is crucial for engineers specifying requirements and for procurement managers accounting for total cost and lead time.
Why is Post-Processing Necessary?
- Tensión residual: The rapid heating and cooling cycles inherent in PBF create internal stresses within the part. These must be relieved to prevent distortion or cracking later.
- Estructuras de apoyo: Overhangs and complex features require support structures during the build, which must be removed.
- Acabado superficial: As-built surfaces are typically rougher than required for sealing, interfacing, or aesthetic purposes.
- Propiedades mecánicas: As-built parts, especially with alloys like AlSi10Mg and A7075, often don’t possess their full potential strength or hardness. Heat treatments are needed to optimize these properties.
- Tolerances: While AM achieves reasonable accuracy, critical dimensions often require machining to meet tight engineering tolerances.
- Eliminación del polvo: Loose or partially sintered powder must be removed from internal channels and surface crevices.
Common Post-Processing Steps for Aluminum AM Sensor Brackets:
- Tratamiento térmico antiestrés:
- Propósito: Este suele ser el very first step after the build finishes, sometimes performed while the part is still attached to the build plate. It involves heating the part in a furnace to a specific temperature (below the alloy’s aging temperature, typically 200-300°C for AlSi10Mg) for a set duration, followed by slow cooling. This allows internal stresses to relax, significantly reducing the risk of warping or cracking during support removal or subsequent machining.
- Importancia: Absolutely critical for dimensional stability, especially for complex geometries or high-strength alloys like A7075 which are more prone to stress. Skipping or improperly performing stress relief can lead to part failure.
- Extracción de la pieza de la placa de montaje:
- Método: Parts are typically printed on a thick metal build plate. They need to be separated, usually using:
- Electroerosión por hilo (EDM): Precise, minimal force, good for delicate parts, but slower.
- Bandsaw: Faster, more common for robust parts, but requires careful handling and leaves a rougher surface at the cut line.
- Consideración: The removal method can influence subsequent finishing requirements for the base surface.
- Método: Parts are typically printed on a thick metal build plate. They need to be separated, usually using:
- Retirada de la estructura de soporte:
- Métodos: Depending on the support design (e.g., solid, lattice, conical points), removal can involve:
- Manual Breaking: Supports designed with breakaway points can sometimes be snapped off by hand or with simple tools.
- Herramientas de corte: Pliers, grinders, or specialized tools may be needed.
- Machining (Milling/Grinding): Sometimes required for supports in difficult-to-reach areas or where a clean surface finish is needed at the support interface.
- Desafíos: Can be labor-intensive, especially for complex internal supports. Risk of damaging the part surface if not done carefully. This highlights the importance of DfAM in minimizing support needs.
- Métodos: Depending on the support design (e.g., solid, lattice, conical points), removal can involve:
- Powder Removal / Cleaning:
- Propósito: Remove any loose or semi-sintered powder trapped in internal channels, lattice structures, or surface porosity.
- Métodos: Compressed air, bead blasting, ultrasonic cleaning baths.
- Importancia: Trapped powder can add weight, potentially contaminate systems, or become dislodged during operation. Thorough cleaning is essential, especially for parts with internal features.
- Solutionizing and Aging Heat Treatment (e.g., T6 Temper):
- Propósito: To achieve the desired final mechanical properties (hardness, yield strength, tensile strength) for precipitation-hardenable alloys like AlSi10Mg and A7075.
- Process (Typical T6 for Aluminum):
- Solución Tratamiento: Heat the part to a high temperature (e.g., ~500-540°C) to dissolve alloying elements into the aluminum matrix.
- Enfriamiento: Rapidly cool the part (e.g., in water or polymer) to trap the elements in a supersaturated solid solution.
- Artificial Aging: Re-heat the part to a lower temperature (e.g., ~150-190°C) for a specific time, allowing controlled precipitation of strengthening phases.
- Resultado: Significantly increases strength and hardness compared to the as-built or stress-relieved state. Specific parameters depend on the alloy and desired properties.
- Acabado superficial:
- Goal: Improve surface smoothness, provide a uniform appearance, deburr edges, or prepare for coating.
- Common Methods for Brackets:
- Abrasive Blasting (Bead, Sand, Grit): Uses high-pressure media to clean the surface, remove oxides, create a uniform matte texture, and can provide some peening effect improving fatigue life. Media type (glass beads, aluminum oxide, etc.) affects the final finish.
- Tumbling (Vibratory Finishing / Mass Finishing): Parts are placed in a tub with abrasive media and vibrated or tumbled. Good for deburring edges, improving Ra, and achieving a consistent finish on batches of parts, especially those with complex external shapes.
- Manual Deburring/Fettling: Hand finishing using files or grinders to remove support witnesses or sharp edges.
- Mecanizado CNC:
- Propósito: Achieve tight tolerances on critical features that cannot be met by the as-built AM process or other finishing methods.
- Applications for Brackets: Machining mounting holes to precise diameters and positional tolerances, creating flat mating surfaces for sensor mounting, machining interfaces for connectors or other components.
- Consideración: Requires appropriate fixturing; machinability can vary slightly depending on the AM process and heat treatment condition.
- Coating and Surface Treatments:
- Propósito: Enhance corrosion resistance, improve wear resistance, provide electrical insulation, or achieve a desired aesthetic/color.
- Options for Aluminum Brackets:
- Anodizing (Type II or Type III Hardcoat): Forms a controlled, durable oxide layer. Improves corrosion and wear resistance. Essential for A7075 in potentially corrosive environments. Can be dyed various colors (Type II).
- Chemical Conversion Coating (e.g., Alodine, Chromate/Non-Chromate): Provides corrosion protection and an excellent base for paint adhesion.
- Painting / Powder Coating: Provides corrosion protection and a wide range of color/finish options.
Integrating Post-Processing into Workflow:
The sequence and selection of post-processing steps depend heavily on the specific requirements of the sensor bracket.
Example Workflow (High-Precision Bracket): Build -> Stress Relief -> Wire EDM Removal -> Support Removal -> Bead Blast (Cleaning) -> Solution & Aging (T6) -> CNC Machining (Critical Features) -> Deburr -> Anodize -> Final Inspection.
Engineers must clearly define all necessary post-processing requirements on part drawings and specifications. Procurement managers need to ensure potential AM service providers have the capabilities (in-house or through trusted partners) to perform all required steps and must factor the associated costs and lead times into their sourcing decisions. A full-service provider offering end-to-end solutions from printing through final finishing and inspection can often streamline the process for B2B customers.
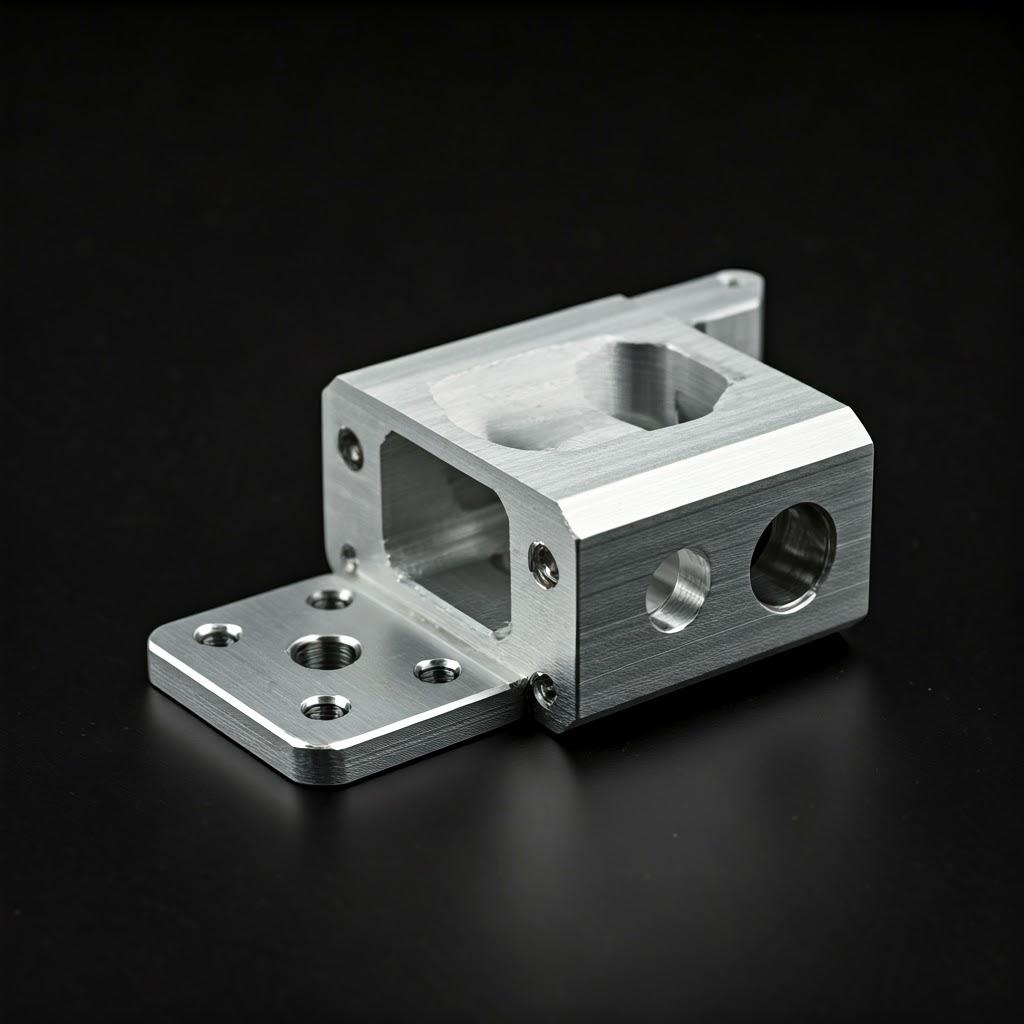
Navigating Potential Hurdles: Common Challenges in 3D Printing Sensor Brackets and Solutions
While metal additive manufacturing offers significant advantages for producing automotive sensor brackets, it’s not without its challenges. Understanding potential hurdles during the printing and post-processing stages allows engineers and manufacturers to proactively implement mitigation strategies, ensuring consistent quality and reliable production. Partnering with an experienced AM service provider is key to navigating these complexities.
Common Challenges and Mitigation Strategies:
- Deformación y distorsión:
- Causa: Uneven heating and cooling during the layer-by-layer fusion process create residual stresses within the part. As these stresses build up, they can overcome the anchoring effect of the supports or the part’s own stiffness, causing it to warp or distort, especially after removal from the build plate. Thin walls, large flat sections, and asymmetrical geometries are more susceptible.
- Mitigación:
- DfAM: Design for minimal thermal gradients (avoid abrupt thickness changes), add stiffening features (ribs).
- Orientación: Orient the part to minimize large cross-sections per layer and reduce unsupported overhangs.
- Estrategia de apoyo: Use robust support structures strategically placed to counteract shrinkage forces and anchor the part effectively.
- Parámetros del proceso: Optimize parameters (e.g., scan strategy, laser power) to minimize localized overheating.
- Simulación térmica: Use simulation software to predict high-stress areas and potential distortion, guiding design or support adjustments.
- Alivio del estrés: Perform stress relief heat treatment immediately after printing, before part removal.
- Tensión residual:
- Causa: Inherent to PBF due to rapid solidification and large thermal gradients. Even if warping is controlled, high internal stresses can remain.
- Impacto: Can lead to premature failure under load (especially fatigue), cracking during post-processing (machining), or long-term dimensional instability.
- Mitigación:
- Optimización de procesos: Fine-tuning parameters can influence stress levels.
- Tratamiento térmico antiestrés: The primary method for reducing residual stress to acceptable levels. Proper execution is crucial.
- Diseño: Smooth geometrical transitions reduce stress concentrations.
- Post-Processing Awareness: Understand that machining can release stresses, potentially causing minor dimensional changes. Sometimes machining is done partially before final stress relief.
- Porosity (Gas and Lack-of-Fusion):
- Causa:
- Porosidad del gas: Trapped gases (e.g., argon from the build chamber, hydrogen dissolved in the powder/melt) form small, typically spherical pores during solidification. Often caused by contaminated powder or inadequate chamber atmosphere control.
- Porosidad por falta de fusión: Insufficient energy input (laser power too low, scan speed too high) or poor powder layer uniformity prevents complete melting and fusion between layers or adjacent scan tracks, leaving irregular-shaped voids.
- Impacto: Reduces part density, degrades mechanical properties (especially fatigue strength and ductility), creates potential leak paths, and acts as crack initiation sites.
- Mitigación:
- Polvo de alta calidad: Use powder with controlled low gas content, good sphericity, and flowability. Sourcing from reputable suppliers like Met3dp, known for their advanced atomization processes (VIGA, PREP) ensuring powder purity and quality, is essential.
- Parameter Optimization: Develop and rigorously control validated process parameters for the specific material and machine.
- Machine Maintenance: Ensure proper inert gas flow, low oxygen levels in the build chamber, and clean optics.
- Manipulación de polvos: Implement proper storage and handling procedures to prevent moisture or gas absorption.
- Prensado isostático en caliente (HIP): A post-processing step involving high temperature and pressure, which can close internal pores (both gas and LoF), significantly improving density and mechanical properties. Often used for critical applications but adds cost and lead time.
- Ensayos no destructivos (END): Use methods like CT scanning to detect internal porosity in critical parts.
- Causa:
- Cracking (Solidification/Liquation):
- Causa: Particularly challenging in alloys with a wide freezing range, like A7075. Stresses induced during solidification can cause cracks to form along grain boundaries or in areas where lower-melting-point phases exist (liquation cracking). Rapid cooling rates exacerbate the issue.
- Mitigación:
- Alloy Modification: Sometimes slightly modified compositions are used for AM to improve weldability.
- Control de procesos: Precise control over laser parameters, potentially using pulsed lasers or specific scan strategies (e.g., melt pool temperature control).
- Gestión térmica: Using heated build platforms or optimized heating/cooling cycles.
- Alivio del estrés: Prompt stress relief can prevent stress-induced cracking after the build.
- HIP: Can sometimes help heal micro-cracks, but less effective on large solidification cracks. Requires careful parameter development by the AM provider.
- Support Removal Difficulties & Surface Quality:
- Causa: Poorly designed support structures (too dense, wrong type, bad placement) or overly complex part geometries requiring extensive internal supports.
- Impacto: Increased labor cost for removal, potential damage to the part surface (scratches, gouges), residual support material (stubs) affecting fit or aesthetics, poor surface finish at support interface points.
- Mitigación:
- DfAM Focus: Prioritize designing parts to be self-supporting or minimize support needs. Use fillets/chamfers instead of sharp overhangs.
- Optimized Support Generation: Use advanced support generation software features (e.g., block supports, tree supports, conical supports) designed for easier removal and minimal part contact.
- Skilled Technicians: Experienced technicians are better at carefully removing supports without damaging the part.
- Post-procesamiento: Plan for necessary finishing steps (machining, blasting, tumbling) to clean up support witness marks.
- Dimensional Inaccuracy:
- Causa: Cumulative effect of minor shrinkage variations, slight warping, parameter drift over long builds, or inaccuracies in compensating for thermal expansion/contraction.
- Impacto: Parts may not meet critical tolerances required for assembly or function.
- Mitigación:
- Machine Calibration & Monitoring: Ensure the AM system is accurately calibrated and stable. Implement in-process monitoring where available.
- Shrinkage Compensation: Apply appropriate scaling factors in the build preparation software based on empirical data for the specific material and machine.
- Control de procesos: Maintain tight control over all critical process parameters.
- Post-Machining: Accept that achieving the tightest tolerances often requires machining critical features after printing and heat treatment. Design parts with machining allowances where needed.
Asociarse para el éxito:
Successfully navigating these challenges requires a combination of robust design practices, meticulous process control, thorough post-processing, and rigorous quality assurance. For automotive OEMs and Tier 1 suppliers, partnering with a knowledgeable and experienced metal AM service provider is paramount. Look for providers who:
- Demonstrate expertise with the specific alloys (AlSi10Mg, A7075).
- Have well-maintained, calibrated equipment.
- Employ rigorous process control and quality management systems (e.g., ISO 9001, ideally IATF 16949 awareness/certification for automotive).
- Utilize high-quality, traceable powders.
- Offer DfAM guidance and feedback.
- Possess comprehensive post-processing capabilities (or strong partnerships).
- Provide robust inspection and reporting (metrology, NDT if required).
By understanding these potential hurdles and working with capable partners, companies can confidently leverage metal AM to produce high-performance, reliable sensor brackets for the demanding requirements of EVs and autonomous vehicles.
Partnering for Success: How to Choose the Right Metal 3D Printing Service Provider for Automotive Components
Leveraging metal additive manufacturing for critical components like automotive sensor brackets requires more than just access to a printer; it demands a strategic partnership with a capable and reliable service provider. The quality, performance, and consistency of your final parts are directly tied to the expertise, processes, and quality controls of your chosen supplier. For engineers and procurement managers in the demanding automotive sector, particularly those involved with EV and autonomous vehicle development, selecting the right metal AM partner is a crucial decision impacting project timelines, component reliability, and overall success. Making an informed choice requires careful evaluation based on a range of technical and operational criteria.
Key Evaluation Criteria for Selecting a Metal AM Service Provider:
- Technical Expertise and Application Experience:
- Material Specialization: Does the provider have demonstrable experience printing and post-processing the specific aluminum alloys you need (AlSi10Mg, A7075)? Ask for case studies or examples of similar parts they have produced.
- Automotive Focus: Do they understand the specific requirements and challenges of the automotive industry (e.g., durability, environmental testing, PPAP processes)? Experience with sensor brackets or similar structural components is a plus.
- Apoyo al DfAM: Can they provide expert feedback on your designs to optimize them for additive manufacturing, potentially improving performance and reducing cost? Do they have engineers who can actively collaborate on design optimization?
- Problem-Solving: Metal AM can present unexpected challenges. Does the provider have a team with the deep technical knowledge to troubleshoot issues related to printing, materials, or post-processing? Look for providers with a strong R&D background or collective expertise, like the team at Met3dp, whose background spans materials science and application development.
- Machine Capabilities and Technology:
- Process Suitability: Primarily L-PBF for aluminum, but do they have well-maintained, industrial-grade machines suitable for the required precision and material?
- Construir volumen: Can their machines accommodate the size of your sensor brackets?
- Funciones avanzadas: Do they utilize machines with process monitoring capabilities (e.g., melt pool monitoring, thermal imaging) that can help ensure build quality and consistency?
- Maintenance & Calibration: What are their procedures for machine maintenance and regular calibration to ensure consistent output?
- Material Quality, Control, and Traceability:
- Abastecimiento de polvo: Do they source high-quality metal powders from reputable manufacturers (or produce their own, like Met3dp)? What are their incoming powder inspection and testing procedures?
- Certificación: Can they provide material certifications (Certificates of Analysis/Conformance) for the specific powder batch used for your parts?
- Trazabilidad: Is there a robust system for tracking powder batches throughout the process, linking the final part back to the specific material used? This is critical for automotive accountability.
- Handling & Storage: Are proper procedures in place for storing and handling powders to prevent contamination (especially moisture for aluminum) and maintain flowability? How is powder reused/refreshed?
- Quality Management System (QMS):
- Certificación ISO 9001: This is a baseline requirement, indicating a documented system for quality control and continuous improvement.
- IATF 16949 Certification/Compliance: While full certification might be less common for pure AM service bureaus compared to Tier 1 suppliers, demonstrated compliance or alignment with IATF 16949 principles is a significant advantage for automotive projects. It shows commitment to rigorous process control, risk management (FMEA), traceability, and documentation practices (like APQP – Advanced Product Quality Planning, and PPAP – Production Part Approval Process). Ask about their familiarity and capability to support these automotive requirements.
- Capacidades de postprocesado:
- End-to-End Solutions: Does the provider offer a comprehensive suite of in-house post-processing services (stress relief, heat treatment specific to Al alloys, support removal, basic finishing)?
- Managed Partner Network: If services like precision CNC machining, advanced surface finishing (e.g., tumbling, polishing), or specialized coatings (anodizing, painting) are outsourced, does the provider have a network of qualified and audited partners? How do they manage quality control for these external processes?
- Experiencia: Is there specific expertise in heat treating aluminum AM parts (which can differ subtly from wrought/cast materials) and machining AM components?
- Metrology and Inspection:
- Equipamiento: Do they possess the necessary metrology equipment (CMMs, 3D scanners, surface profilometers) to verify dimensional accuracy and surface finish requirements?
- NDT Capabilities: If required for critical parts, can they perform or manage Non-Destructive Testing like CT scanning (for internal defects) or dye penetrant testing (for surface cracks)?
- Informar: What level of inspection reporting can they provide? Can they accommodate specific GD&T callouts and reporting formats?
- Capacity, Scalability, and Lead Times:
- Current Capacity: Can they meet your required volumes (prototype and potential production) within acceptable lead times? What is their typical machine utilization?
- Scalability Plan: If your volumes increase, how would they scale production? Do they have multiple machines or plans for expansion?
- On-Time Delivery: What is their track record for meeting quoted lead times? Ask for references or performance data if possible.
- Communication and Support:
- Capacidad de respuesta: Are they quick to respond to inquiries and provide quotes?
- Transparencia: Is communication clear regarding technical feasibility, potential challenges, costs, and timelines?
- Gestión de proyectos: Is there a dedicated point of contact for your projects?
- Rentabilidad:
- Transparent Pricing: Is the quoting clear and detailed, breaking down costs where possible?
- Value Proposition: Consider the total value, including technical support, quality assurance, reliability, and post-processing, not just the per-part price. The cheapest option may not offer the necessary quality or support for automotive applications.
Due Diligence:
Don’t rely solely on websites or brochures. Engage in technical discussions, ask detailed questions, request sample parts (perhaps printing a benchmark design), and, if feasible, conduct a facility audit (either in-person or virtual) to verify capabilities and processes. Building a strong, collaborative relationship with your AM service provider is key to successfully integrating 3D printed sensor brackets into your automotive projects.
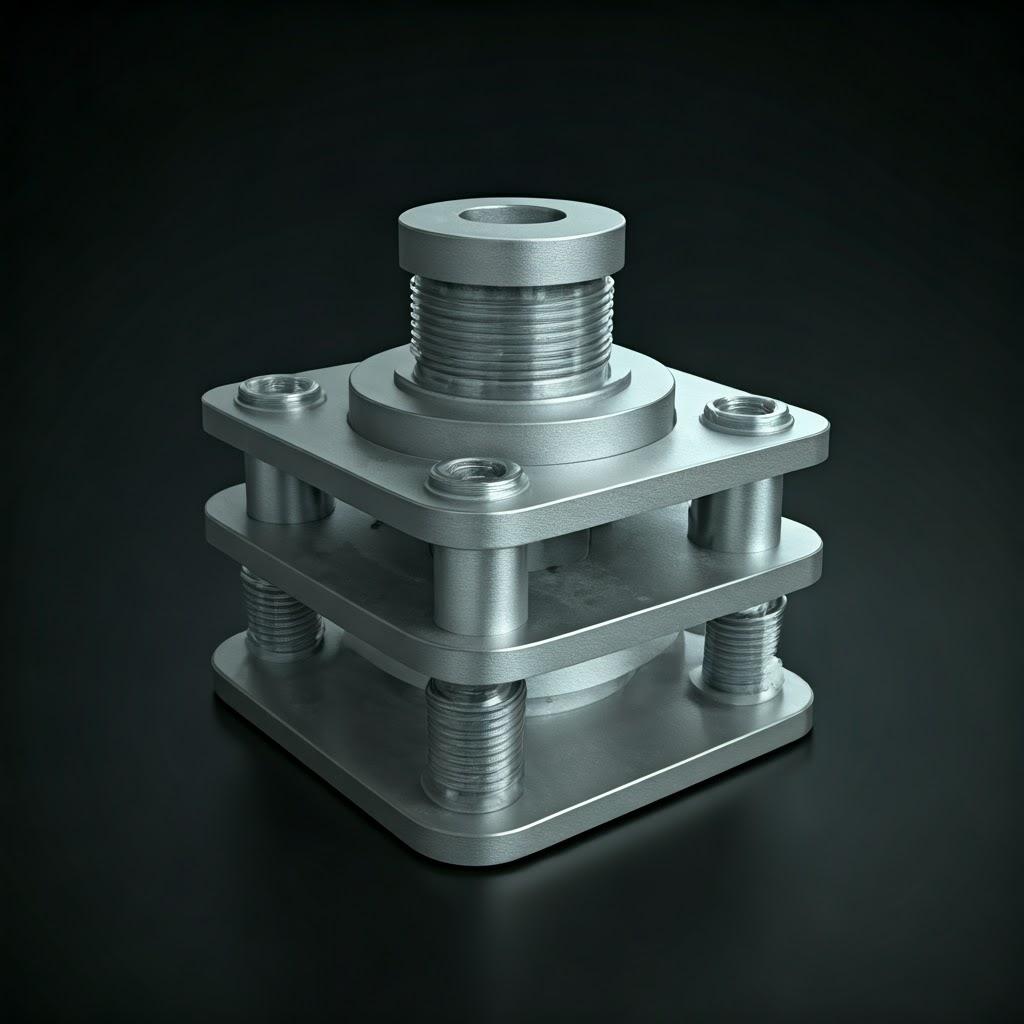
Understanding Investment: Cost Factors and Lead Times for 3D Printed Sensor Brackets
One of the most pressing questions for engineers and procurement managers considering metal additive manufacturing is: “What will it cost, and how long will it take?” While AM eliminates the significant upfront investment in tooling associated with casting or stamping, the cost structure and lead time calculation differ from traditional methods. Understanding the key drivers helps in budgeting, comparing quotes, and making informed decisions about adopting AM for sensor bracket production.
Key Cost Drivers for Metal AM Sensor Brackets:
The final price quoted by an AM service provider is typically a culmination of several factors:
- Coste del material:
- Powder Price: The cost per kilogram of the metal powder. High-performance alloys like A7075 are generally more expensive than standard AlSi10Mg due to raw material costs and processing difficulty. Powder quality (sphericity, purity, PSD) also influences price.
- Material Consumption: This includes the volume of the final part plus the volume of support structures needed. Designs optimized for minimal support (DfAM) directly reduce material cost.
- Powder Refresh/Recycling: While unused powder can often be sieved and reused, there are limits. A certain percentage of virgin powder typically needs to be mixed in (refresh rate), and some powder is inevitably lost or degraded over time. These factors are built into the provider’s material costing.
- Tiempo de máquina (tiempo de construcción):
- Primary Driver: This is often the most significant cost component. It’s primarily determined by the total height of the build (Z-height) because printing is layer-by-layer. Taller parts take longer.
- Other Factors: The total volume of material being melted per layer (related to part cross-section and density), the number of parts nested onto the build plate, and the specific process parameters (layer thickness, scan speed) all influence the total machine hours.
- Calculation: Service providers typically have an hourly rate for their machines, which includes depreciation, energy consumption, inert gas usage, maintenance, and operator overhead. Build simulation software estimates the total print time.
- Costes laborales:
- Preprocesamiento: File preparation, build layout optimization (nesting), support structure generation.
- Machine Setup & Operation: Loading powder, setting up the build, monitoring the process, unloading the build plate.
- Trabajo de postprocesado: This can be substantial, including part removal from the plate, powder removal/cleaning, manual support removal (often intricate and time-consuming), basic finishing, and inspection. The more complex the supports or internal features, the higher the labor cost.
- Post-Processing Costs (Beyond Basic Labor):
- Tratamiento térmico: Furnace time and energy for stress relief and/or solutionizing & aging (T6) cycles. These require specialized, calibrated equipment.
- Mecanizado: Costs depend heavily on the number of features to be machined, the required tolerances, complexity of fixturing, and machining time. Charged typically at an hourly rate for CNC machine time plus programming/setup.
- Acabado superficial: Costs for bead blasting, tumbling, anodizing, painting, etc., depend on the process, part size, batch size, and required specifications. Often performed by specialized third parties, adding margin and logistics costs.
- Inspection: Time for CMM measurements, 3D scanning, NDT, and report generation adds to the cost, especially for parts requiring extensive validation (e.g., PPAP level).
- Part Complexity and Design:
- Supports: More complex parts often require more extensive support structures, increasing material use, print time (slightly), and significantly increasing post-processing labor and risk.
- Internal Features: While AM enables complex internal channels or lattices, they can make powder removal and inspection more challenging and costly.
- Optimización de la topología: May reduce material volume (lowering material cost and potentially print time) but can result in complex shapes needing careful support strategies and potentially more finishing labor.
- Build Density (Nesting):
- Eficiencia: The ability to fit multiple sensor brackets efficiently onto a single build plate is crucial for reducing the cost per part. Service providers use software to optimize nesting. Small parts often benefit significantly from batch production.
- Quality Requirements:
- Stringency: Higher quality requirements (tighter tolerances, enhanced inspection, NDT, extensive documentation like full PPAP) naturally increase the labor, equipment, and potential scrap costs, leading to a higher price per part.
Typical Lead Times:
Lead times for metal AM sensor brackets can vary significantly based on similar factors to cost:
- Prototyping (1-10 units):
- Typical: 5 to 15 business days.
- Breakdown: 1-2 days for file prep/queuing, 1-4 days for printing (depending on height/complexity), 3-10+ days for post-processing (stress relief, support removal, basic finishing, potentially heat treatment and expedited machining).
- Low-to-Medium Volume Production (10s to 1000s units):
- Typical: 3 to 8+ weeks.
- Breakdown: Includes time for optimizing build layouts for batch production, potentially longer print runs, scheduling furnace time for batch heat treatments, coordinating batch machining or finishing, and performing batch inspections. Logistics and potential queuing at different process steps contribute.
- Factors Influencing Lead Time:
- Machine Availability/Backlog: Current workload at the service provider.
- Tiempo de construcción: Directly related to part height and number of parts per build.
- Post-Processing Complexity: Machining, specialized coatings, and extensive inspection are often the longest poles in the tent. Outsourced steps add logistical delays.
- Tamaño del lote: Larger batches might take longer overall but benefit from efficiencies at certain steps.
- Quality/Inspection Requirements: Rigorous inspection adds time.
- Envío: Transit time to the customer’s location.
B2B Considerations:
For ongoing B2B relationships involving wholesale or bulk orders, AM service providers may offer tiered pricing based on volume commitments or establish long-term agreements with fixed pricing structures. It’s crucial to discuss expected annual volumes and quality requirements upfront to get realistic quotes and establish reliable supply chain planning. Always compare quotes based on a detailed understanding of what is included in the price and lead time (e.g., which post-processing steps, level of inspection, shipping terms).
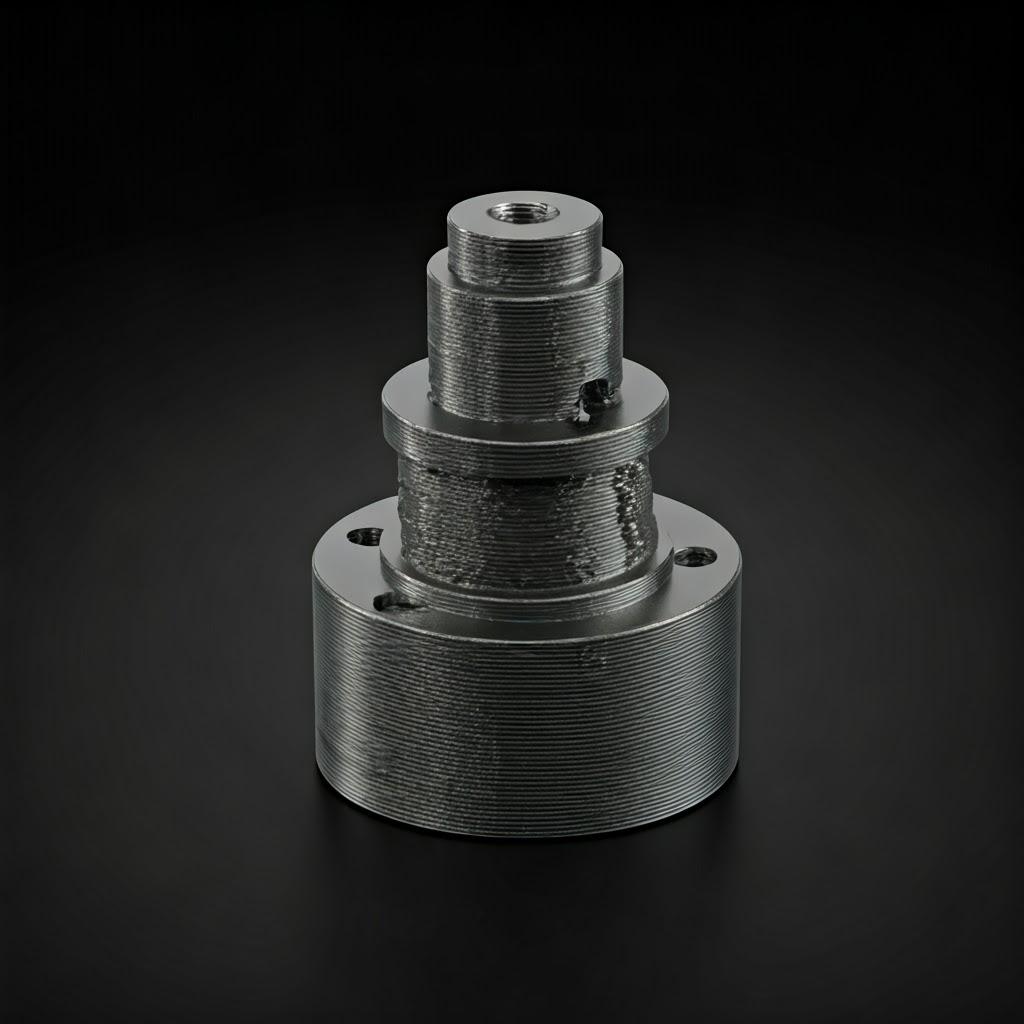
Frequently Asked Questions (FAQ) about 3D Printing Automotive Sensor Brackets
Here are answers to some common questions engineers and procurement managers have when considering metal additive manufacturing for automotive sensor brackets:
Q1: Is metal 3D printing cost-competitive with CNC machining or casting for sensor brackets?
A: It depends heavily on several factors:
- Complejidad: For highly complex, topology-optimized, or consolidated bracket designs that are difficult or impossible to machine or cast, AM can be cost-effective even at lower volumes because it avoids complex setups or tooling.
- Volumen:
- Low Volume (Prototypes, 1-100s): AM is often more cost-effective than casting (due to high tooling costs) and competitive with complex CNC machining (due to programming and setup time).
- Medium Volume (100s-10,000s): This is a gray area. Optimized AM production (good nesting, streamlined post-processing) can compete with machining, especially if DfAM enables significant lightweighting or performance gains. Casting becomes more competitive as volumes increase to amortize tooling, but only if the design is castable.
- High Volume (100,000s+): Traditional methods like die casting or stamping (for simpler designs) are generally more cost-effective per part, assuming the design is suitable.
- Plazo de entrega: If speed is critical (e.g., rapid prototyping, urgent replacement parts), AM’s ability to produce parts in days/weeks without tooling offers significant value that can outweigh a higher per-part cost.
- Total Cost of Ownership: Consider factors beyond the per-part price, such as tooling investment/maintenance (avoided with AM), potential weight savings (improving vehicle efficiency), assembly simplification (part consolidation), and faster time-to-market.
Q2: How does the strength of 3D printed aluminum brackets (AlSi10Mg, A7075) compare to traditional wrought or cast aluminum?
A: When processed and heat-treated correctly, metal AM aluminum parts can exhibit excellent mechanical properties:
- AlSi10Mg: Typically achieves properties comparable to or slightly better than A356/A360 cast aluminum alloys, especially after a T6 heat treatment. The rapid solidification in AM often leads to a finer microstructure, which can enhance strength.
- A7075: This is where AM can truly shine, if processed expertly. Properly printed and T6 heat-treated A7075 can achieve tensile and yield strengths comparable to wrought 7075-T6, which is significantly stronger than most cast aluminum alloys and AlSi10Mg. It offers a strength-to-weight ratio approaching that of some steels.
- Key Factors: Achieving these properties depends critically on using high-quality powder, optimized print parameters (to ensure high density >99.5%), and correct post-build heat treatments (stress relief and T6 cycle). Properties can also exhibit some anisotropy (variation with build direction), which must be considered in design and testing. Always request material property data from your AM provider based on their specific process.
Q3: Can metal AM scale to support automotive series production volumes for sensor brackets?
A: Yes, metal AM is increasingly being adopted for series production in automotive, although typically for niche or medium-volume applications (thousands to tens of thousands of parts per year) rather than mass-market vehicles (millions per year).
- Viability Factors: Scalability depends on the bracket’s size (smaller parts are easier to produce in volume), complexity, the number of printers available, and the efficiency of the entire workflow (including post-processing automation).
- Trends: Costs per part are decreasing as machines become faster, materials improve, and automation increases. AM is particularly attractive for applications where customization, high performance (lightweighting), or complex geometries provide significant value, such as in performance vehicles, EVs, autonomous systems, and the aftermarket.
- Hybrid Approaches: Sometimes AM is used for initial production runs or complex variants, while simpler, higher-volume versions might transition to traditional methods later.
Q4: What kind of quality documentation can I expect from a reputable metal AM supplier for automotive parts?
A: For automotive applications, traceability and documentation are critical. A reputable supplier committed to automotive quality standards should be able to provide:
- Certificación de materiales: Certificate of Analysis (CoA) or Certificate of Conformance (CoC) for the specific powder batch used, confirming its chemical composition and key characteristics.
- Build Report: A log detailing the build parameters used, machine ID, build date, part placement on the build plate, and any significant events during the build.
- Post-Processing Records: Confirmation and parameters used for stress relief and heat treatment cycles (e.g., furnace charts). Traceability for any outsourced processes (machining, coating).
- Dimensional Inspection Report: Measurement results for critical dimensions specified in the drawing (e.g., CMM report, 3D scan comparison report). The level of detail should match the agreed quality plan.
- Non-Destructive Testing (NDT) Report: If required (e.g., CT scan report for porosity, dye penetrant report for surface cracks).
- PPAP Documentation: For series production, suppliers familiar with IATF 16949 should be capable of providing elements of the Production Part Approval Process documentation as required by the customer (e.g., Process Flow Diagram, PFMEA, Control Plan, Measurement System Analysis, dimensional results, material certifications). Clarify PPAP requirements early in the engagement.
Conclusion: Accelerating Automotive Innovation with Metal Additive Manufacturing for Sensor Brackets
The relentless evolution of the automotive industry towards electrification and autonomy hinges on the seamless integration and flawless performance of an ever-increasing array of sophisticated sensors. Providing stable, precise, and reliable mounting for these critical components is paramount, and automotive sensor brackets have evolved from simple fixtures to complex, performance-driven parts. Metal additive manufacturing has emerged as a key enabling technology, offering a transformative approach to designing and producing these vital components.
By leveraging the power of metal AM, particularly with high-performance aluminum alloys like the versatile AlSi10Mg and the high-strength A7075, automotive engineers can overcome the limitations of traditional manufacturing. The unparalleled design freedom afforded by AM unlocks possibilities for:
- Aligeramiento significativo: Through topology optimization and lattice structures, reducing vehicle mass for improved EV range and handling.
- Geometrías complejas: Creating intricate shapes that perfectly package sensors within tight spaces and integrate multiple functions.
- Consolidación de piezas: Reducing assembly complexity, weight, and potential failure points by combining multiple components into a single printed part.
- Creación rápida de prototipos e iteración: Accelerating development cycles for new sensor integrations and vehicle platforms.
- Personalización: Enabling tailored bracket designs for specific sensor models, vehicle variants, or aftermarket applications without tooling constraints.
However, realizing these benefits requires a holistic approach. Success hinges on embracing Design for Additive Manufacturing (DfAM) principles, understanding the critical role of meticulous post-processing (including stress relief and heat treatment) to achieve desired properties and dimensional accuracy, and navigating potential process challenges through careful control and expertise.
Crucially, partnering with the right metal AM service provider is essential. Choosing a supplier with proven expertise in automotive applications, robust quality management systems, advanced material and processing capabilities, and comprehensive post-processing solutions ensures that the final sensor brackets meet the stringent demands of the industry. Companies like Met3dp, offering a synergy of advanced metal powder production, state-of-the-art printing systems, and deep application knowledge, represent the type of integrated partner capable of supporting automotive companies in their AM journey.
Metal 3D printing is no longer just a prototyping tool; it is a viable and increasingly compelling manufacturing solution for complex, high-value automotive components. For sensor brackets in EVs and autonomous vehicles, AM offers a pathway to enhanced performance, accelerated innovation, and a competitive edge in shaping the future of mobility.
Ready to explore how metal additive manufacturing can revolutionize your automotive sensor bracket designs? Contact Met3dp today to discuss your specific requirements and discover how our comprehensive AM solutions can drive your projects forward.
Compartir
MET3DP Technology Co., LTD es un proveedor líder de soluciones de fabricación aditiva con sede en Qingdao, China. Nuestra empresa está especializada en equipos de impresión 3D y polvos metálicos de alto rendimiento para aplicaciones industriales.
Solicite información para obtener el mejor precio y una solución personalizada para su empresa.
Artículos relacionados
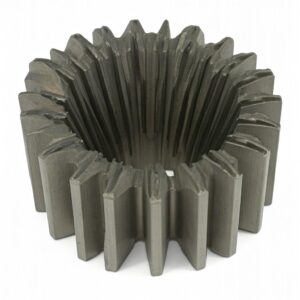
Segmentos de álabe de tobera de alto rendimiento: Revolucionando la eficiencia de las turbinas con la impresión metálica en 3D
Leer Más "Acerca de Met3DP
Actualización reciente
Nuestro producto
CONTACTO
¿Tiene alguna pregunta? ¡Envíenos un mensaje ahora! Atenderemos su solicitud con todo un equipo tras recibir su mensaje.
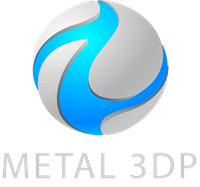
Polvos metálicos para impresión 3D y fabricación aditiva
PRODUCTO
cONTACT INFO
- Ciudad de Qingdao, Shandong, China
- [email protected]
- [email protected]
- +86 19116340731