Protective Sensor Housings for Aerospace via Additive Manufacturing
İçindekiler
Introduction: The Critical Role of Sensor Protection in Aerospace and Additive Manufacturing’s Solution
In the demanding realm of aerospace engineering, sensors are the unsung heroes. They are the nerve endings of an aircraft, spacecraft, or satellite, constantly gathering critical data about temperature, pressure, vibration, strain, proximity, and countless other parameters. This data is fundamental for flight control, structural health monitoring (SHM), environmental awareness, and overall operational safety. However, these sensitive instruments operate in some of the most extreme conditions imaginable – facing drastic temperature fluctuations, intense vibrations, high G-forces, potential impacts, corrosive atmospheres, and electromagnetic interference. Failure is not an option, and therefore, the housings designed to protect these vital sensors are just as critical as the sensors themselves.
Traditionally, manufacturing protective sensor housings involved subtractive methods like CNC machining from billet materials or complex casting processes. While effective, these approaches often come with limitations: significant material waste (buy-to-fly ratio), constraints on geometric complexity, long lead times for design iterations and production, and potentially higher costs, especially for low-volume, highly customized parts. This is where the transformative power of metal katkılı üretim (AM), commonly known as metal 3D baskı, enters the picture.
Metal AM offers a paradigm shift in how we design and produce intricate components like aerospace sensor housings. By building parts layer by layer directly from fine metal powders, guided by a digital model, AM unlocks unprecedented design freedom. Engineers can create highly optimized, lightweight structures with complex internal channels for cooling or wiring, integrated mounting features, and organic shapes perfectly tailored to the specific sensor and its surrounding environment. This capability is particularly crucial in aerospace, where every gram saved translates to fuel efficiency or increased payload capacity.
Furthermore, metal 3D printing significantly shortens development cycles. Prototypes can be produced in days rather than weeks or months, allowing for rapid testing and refinement. On-demand manufacturing becomes feasible, reducing the need for large inventories and enabling the production of replacement parts or customized solutions quickly. Companies seeking reliable aerospace component suppliers are increasingly turning to AM specialists who can deliver high-performance parts with agility.
Met3dp stands at the forefront of this technological revolution. Leveraging decades of collective expertise and cutting-edge technologies like Selective Electron Beam Melting (SEBM) and advanced powder atomization (Gas Atomization and PREP), Met3dp provides comprehensive metal 3D baskı solutions. Our industry-leading printers ensure the accuracy and reliability required for mission-critical aerospace sensor housings, while our high-quality metal powders guarantee superior material properties. We partner with aerospace manufacturers, offering not just parts, but end-to-end solutions from design optimization to final post-processing, ensuring that the protective enclosures for your critical sensors meet the highest standards of performance and reliability. This article explores the specific applications, advantages, material choices, design considerations, and procurement strategies for leveraging metal AM in the production of protective sensor housings for the aerospace industry.
What Are Aerospace Sensor Protectors Used For? Key Applications and Industries
Aerospace sensor protectors, or housings, are essential components designed to shield sensitive electronic, optical, or mechanical sensing elements from the harsh operating environments encountered during flight and space missions. Their primary function is to ensure the sensor’s accuracy, reliability, and longevity by providing a robust barrier against physical damage, environmental extremes, and electromagnetic interference. The specific design and material of a housing depend heavily on the sensor type and its precise location and function within the aircraft or spacecraft. Key applications and the industries relying on these components include:
1. Aerospace Industry (Commercial & General Aviation): * Engine Monitoring: Protecting temperature (EGT), pressure (EPR), vibration, and speed sensors located within or near jet engines or turboprops, environments characterized by extreme heat, vibration, and potential debris. * Air Data Systems: Housing Pitot tubes, static ports, angle-of-attack (AoA) vanes, and temperature probes that measure critical flight parameters (airspeed, altitude, air temperature). These require aerodynamic efficiency and resistance to icing and impact. * Structural Health Monitoring (SHM): Enclosing strain gauges, accelerometers, acoustic sensors, and fiber optic sensors used to monitor the integrity of the airframe, wings, and landing gear, detecting fatigue, stress, or damage. Housings must ensure intimate contact and survive long-term exposure. * Flight Control Surfaces: Protecting position sensors (LVDTs, RVDTs) that monitor the deflection of ailerons, elevators, rudders, and flaps. These housings need to withstand aerodynamic loads and vibration. * Fuel Systems: Shielding fuel quantity probes, temperature sensors, and pressure sensors within fuel tanks, requiring chemical compatibility and leak-proof designs. * Environmental Control Systems (ECS): Protecting temperature, pressure, and humidity sensors within the cabin and bleed air systems. * İniş takımları: Housing proximity sensors (for gear up/down status), brake temperature sensors, and tire pressure sensors, demanding high impact resistance.
2. Defence Sector (Military Aircraft & UAVs): * Enhanced Sensor Suites: Military platforms often carry more sophisticated and numerous sensors for surveillance, targeting, electronic warfare (EW), and reconnaissance. Their housings require not only environmental protection but potentially stealth characteristics (specific materials and coatings) and resistance to ballistic or shrapnel impact. * Harsh Operating Theatres: Housings must withstand extreme conditions found in diverse global operational zones, including sand, dust, salt spray, and potential chemical/biological contaminants. * UAV/Drone Payloads: Protecting sensitive camera gimbals, LiDAR scanners, SIGINT receivers, and other mission-specific sensors on unmanned aerial vehicles. Weight is often a primary constraint, making lightweight AM designs highly advantageous. Wholesale sensor housing suppliers specializing in UAV components find AM particularly beneficial.
3. Space Applications (Satellites, Launch Vehicles, Exploration Probes): * Aşırı Sıcaklıklar: Housings must protect sensors from the intense heat during launch and atmospheric re-entry, as well as the extreme cold of deep space. Thermal management features are often integrated into the housing design. * Radiation Hardening: Sensors and their enclosures must withstand high levels of cosmic radiation without degradation. Material selection and potentially additional shielding layers are critical. * Vacuum Stability: Materials used must exhibit low outgassing properties to prevent contamination of sensitive optics or mechanisms in the vacuum of space. * Launch Stresses: Housings must endure the severe vibration, acoustic loads, and G-forces experienced during rocket launches.
4. Related Industrial Applications: * While the focus is aerospace, similar protective housings are needed in demanding industrial environments like high-temperature furnaces, chemical processing plants, or heavy machinery, where sensors monitor critical process parameters. Lessons learned and materials used in aerospace often find application here.
The need for reliable, often customized, sensor protection applications spans across these critical sectors. Procurement managers and engineers are constantly seeking aerospace component suppliers ve distributors who can deliver robust, lightweight, and rapidly manufactured solutions. Metal additive manufacturing provides a powerful tool to meet these diverse and challenging requirements.
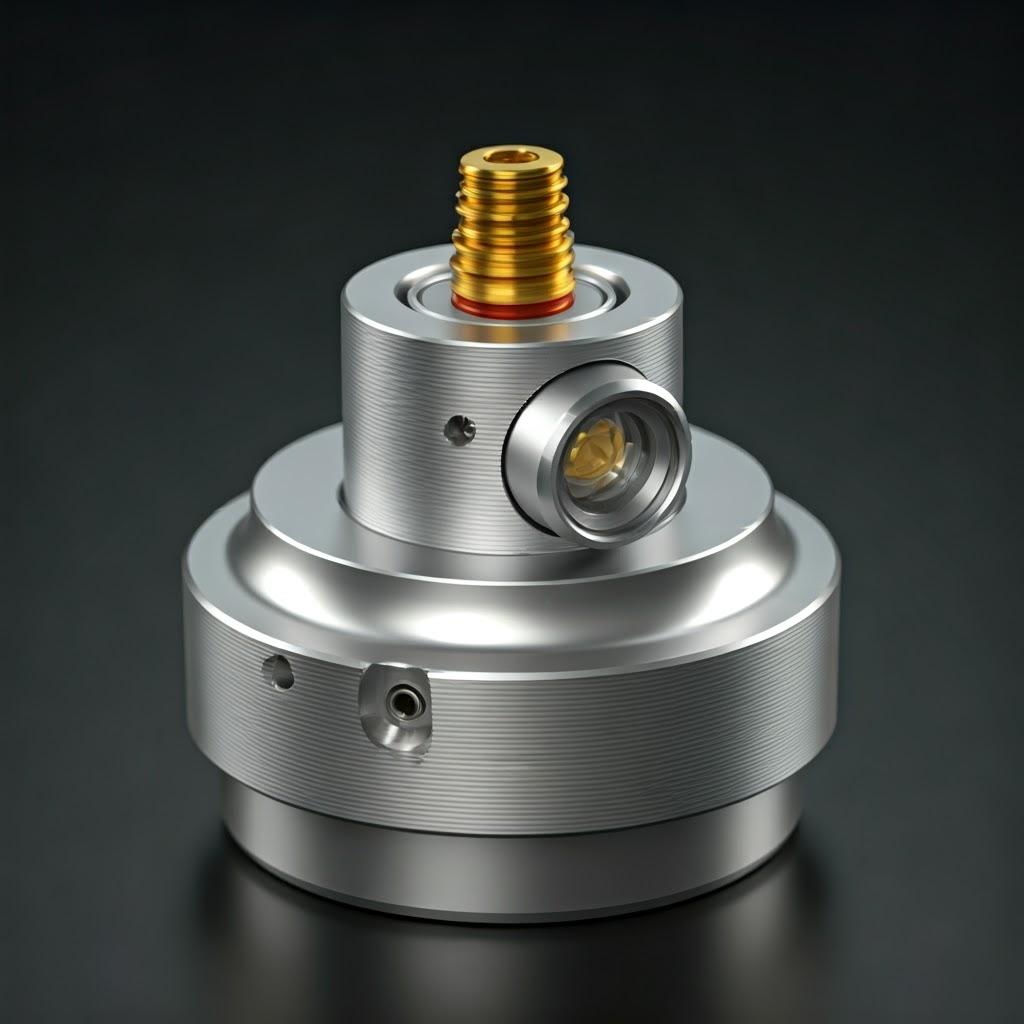
Why Use Metal 3D Printing for Aerospace Sensor Housings? Advantages Over Traditional Methods
The decision to adopt metal additive manufacturing (AM) for producing aerospace sensor housings stems from a convergence of compelling advantages that directly address the limitations of traditional methods like CNC machining, casting, or sheet metal fabrication. For engineers and procurement managers focused on performance, cost, and lead time, metal AM offers a transformative approach.
Key Advantages of Metal AM for Sensor Housings:
- Unmatched Design Freedom & Complexity:
- Traditional: Machining is constrained by tool access, requiring multi-axis setups for complex parts, and often necessitating assembly of multiple simpler components. Casting requires expensive tooling (molds) and has limitations on wall thickness and intricate features.
- Metal AM: Builds parts layer-by-layer, enabling the creation of highly complex internal and external geometries impossible or prohibitively expensive to achieve otherwise. This allows for:
- Conformal Cooling/Heating Channels: Integrating channels directly within the housing walls that perfectly follow the contours of the sensor or internal electronics for highly efficient thermal management.
- Internal Wiring Pathways: Designing enclosed pathways for wiring, providing inherent shielding and protection.
- Integrated Mounting Features: Brackets, bosses, and attachment points can be built directly into the housing, reducing part count, assembly time, and potential failure points.
- Organic Shapes & Topology Optimization: Utilizing software to remove material from non-critical areas, resulting in lightweight yet strong structures perfectly optimized for their specific load conditions.
- Significant Weight Reduction:
- Traditional: Often results in bulkier designs due to manufacturing constraints or the need to machine from standard stock material sizes. Achieving lightweighting typically involves extensive, time-consuming machining passes.
- Metal AM: Enables topology optimization and the use of internal lattice structures to drastically reduce mass while maintaining or even increasing structural integrity. This is paramount in aerospace, where reducing the “buy-to-fly” ratio and overall component weight directly impacts fuel efficiency, payload capacity, and performance.
- Rapid Prototyping and Accelerated Development Cycles:
- Traditional: Creating prototypes via machining or casting involves setup time, potentially tooling creation, and longer machining cycles. Design iterations can take weeks or months.
- Metal AM: Allows engineers to go directly from a CAD model to a physical metal part in a matter of days. This facilitates rapid design validation, functional testing, and iterative refinement, significantly shortening the overall product development timeline. Multiple design variations can be printed simultaneously in a single build.
- Parçaların Birleştirilmesi:
- Traditional: Complex sensor assemblies might consist of a housing body, lid, brackets, fasteners, and internal supports, all manufactured separately and then assembled.
- Metal AM: Offers the possibility to consolidate multiple components into a single, monolithic printed part. This reduces assembly labor, eliminates potential leak paths or failure points at joints, simplifies inventory management, and often improves overall structural performance.
- On-Demand Manufacturing & Reduced Inventory:
- Traditional: Often relies on minimum order quantities to justify tooling costs or setup times, leading to large inventories of spare parts that may never be used.
- Metal AM: Ideal for low-to-medium volume production and “spare parts on demand.” Housings can be printed as needed, reducing warehousing costs and minimizing waste associated with obsolete inventory. This is particularly valuable for legacy aircraft or highly customized applications. Companies acting as aerospace parts distributors can leverage AM for agile fulfillment.
- Malzeme Verimliliği:
- Traditional: Subtractive manufacturing, especially CNC machining, starts with a larger block of material and removes significant portions, generating substantial waste (chips/swarf). The buy-to-fly ratio can be very high (e.g., 10:1 or more).
- Metal AM: An additive process that uses only the material needed to build the part and its supports. Unfused powder within the build chamber can typically be recycled and reused for subsequent builds, leading to much higher material utilization and a significantly lower buy-to-fly ratio.
- Customization and Bespoke Solutions:
- Traditional: Customization often requires unique tooling or extensive reprogramming, making it costly and time-consuming for small batches.
- Metal AM: Highly adaptable to producing unique or customized sensor housing designs without the need for dedicated tooling. Each part can potentially be unique, tailored to specific sensor dimensions, mounting locations, or performance requirements.
Comparative Overview: AM vs. Traditional for Sensor Housings
Özellik | Metal Katmanlı Üretim (AM) | Traditional Manufacturing (CNC, Casting) | Aerospace Relevance |
---|---|---|---|
Tasarım Karmaşıklığı | Very High (internal channels, lattices, organics) | Moderate to High (tool access, mold limits) | Enables optimized, multifunctional designs |
Ağırlık Azaltma | Excellent (topology optimization, lattices) | Good (requires extensive machining) | Critical for fuel efficiency & payload |
Lead Time (Proto) | Fast (Days) | Slow (Weeks/Months) | Accelerates development & testing |
Parça Konsolidasyonu | High Potential | Low (often requires assembly) | Reduces complexity, weight, failure points |
Malzeme Atıkları | Low (additive process, powder recycling) | Yüksek (eksiltici süreç) | Lowers buy-to-fly ratio, more sustainable |
Kalıp Maliyeti | None (digital manufacturing) | High (molds, jigs, fixtures) | Economical for low volumes & customization |
Özelleştirme | Easy & Cost-Effective | Costly & Time-Consuming | Enables bespoke solutions for unique needs |
Min. Order Qty | Low (often single piece feasible) | Often High (to amortize setup/tooling) | Facilitates on-demand production & spares |
Potential Supplier | AM Service Bureaus (e.g., Met3dp), In-house AM | Machine Shops, Foundries | Requires specialized expertise & equipment (AM) |
Export to Sheets
While traditional methods remain viable for certain high-volume or less complex housings, the distinct advantages of metal 3D baskı make it an increasingly compelling choice for demanding aerospace sensor housing applications, offering superior performance, faster development, and greater design flexibility.
Recommended Materials (316L & Ti-6Al-4V) and Why They Excel in Aerospace Applications
Choosing the right material is paramount for aerospace components, and sensor housings are no exception. The material must withstand the harsh operating environment, provide necessary structural support, be compatible with the sensor elements, and meet stringent aerospace certification requirements. Metal additive manufacturing offers a growing portfolio of qualified materials, but two stand out as workhorses for aerospace applications, including sensor housings: 316L Paslanmaz Çelik ve Ti-6Al-4V Titanyum Alaşımı.
Met3dp, leveraging its advanced powder production capabilities including Gas Atomization and Plasma Rotating Electrode Process (PREP), manufactures high-sphericity, high-flowability powders of these and other critical alloys (like CoCrMo, Nickel superalloys, and specialized Titanium grades including TiNi, TiTa, TiAl, TiNbZr), ensuring optimal performance in demanding AM processes like SEBM and LPBF. As a leading metal tozu tedarikçisi and AM service provider, we understand the nuances of these materials.
1. 316L Stainless Steel (UNS S31603): The Versatile Choice
316L is an austenitic chromium-nickel stainless steel containing molybdenum, which significantly enhances its corrosion resistance, particularly against chlorides and other industrial solvents. It is widely used across many industries, including aerospace, for its excellent combination of properties and processability via AM.
- Key Properties & Benefits for Aerospace Sensor Housings:
- Mükemmel Korozyon Direnci: Resists atmospheric corrosion, oxidation, and attack from various chemicals, crucial for housings exposed to jet fuel, de-icing fluids, hydraulic oils, and marine environments (for naval aircraft or coastal operations).
- Good Strength and Ductility: Offers a good balance of tensile strength (typically 500-600 MPa after printing and stress relief) and ductility (elongation >30%), providing robustness against vibration and mechanical loads.
- Weldability & Machinability: Can be easily welded (if post-assembly is needed, though AM aims to consolidate) and machined for critical tolerance features or surface finishes.
- Biocompatibility (for certain grades/finishes): While less relevant for typical sensor housings, its use in medical implants highlights its inertness.
- Maliyet-Etkinlik: Generally less expensive than titanium alloys, making it a suitable choice when the extreme weight savings or high-temperature performance of titanium is not strictly required.
- İyi Basılabilirlik: Well-characterized and relatively easy to process using Laser Powder Bed Fusion (LPBF) and other AM techniques, yielding dense, reliable parts.
- Typical Aerospace Applications: Housings for sensors in less temperature-critical areas, brackets, enclosures in cabins or cargo bays, components requiring high corrosion resistance, ground support equipment interfaces.
- Sınırlamalar:
- Relatively high density (~8.0 g/cm³) compared to titanium or aluminum alloys.
- Lower strength-to-weight ratio than titanium.
- Maximum service temperature is generally limited to around 425°C (800°F) for continuous service, though higher intermittent exposure may be possible.
Material Properties Overview: 316L (Typical AM)
Mülkiyet | Tipik Değer | Significance for Sensor Housings |
---|---|---|
Yoğunluk | ~8,0 g/cm³ | Higher weight compared to Ti |
Nihai Çekme Dayanımı | 500 - 600 MPa | Good structural integrity |
Akma Dayanımı | 200 - 450 MPa | Resistance to permanent deformation |
Kopma Uzaması | > 30% | Good ductility, toughness against vibration/impact |
Sertlik (HRC) | ~20-25 HRC (annealed) | Orta derecede aşınma direnci |
Max Service Temp. | ~425°C (Continuous) | Suitable for many, but not extreme heat areas |
Korozyon Direnci | Mükemmel | Key benefit for harsh environments |
Export to Sheets
2. Ti-6Al-4V (Grade 5 Titanium Alloy): The High-Performance Standard
Ti-6Al-4V (often called Ti64) is the most widely used titanium alloy, accounting for more than 50% of all titanium tonnage worldwide. Its combination of high strength, low density, excellent corrosion resistance, and high-temperature capability makes it a premier choice for demanding aerospace applications, including critical sensor housings.
- Key Properties & Benefits for Aerospace Sensor Housings:
- Mükemmel Güç-Ağırlık Oranı: Offers strength comparable to many steels but at nearly half the density. This is a primary driver for its use in aerospace, enabling significant weight savings.
- Yüksek Mukavemet: Exhibits high tensile strength (typically >900 MPa, often exceeding 1000 MPa after heat treatment) even at elevated temperatures.
- Outstanding Corrosion Resistance: Forms a stable, protective oxide layer providing exceptional resistance to atmospheric corrosion, saltwater, and many industrial chemicals. Superior to 316L in many aggressive environments.
- High-Temperature Capability: Can operate at temperatures up to approximately 400°C (750°F), and potentially higher for short durations, making it suitable for housings near engines or in high-speed flight applications.
- Biyouyumluluk: Widely used for medical implants due to its excellent biocompatibility.
- İyi Basılabilirlik: Can be successfully processed using LPBF and Electron Beam Melting (EBM/SEBM) techniques. SEBM, a capability offered by Met3dp, is often preferred for titanium alloys due to its vacuum environment and higher processing temperatures, which can reduce residual stresses and minimize contamination.
- Typical Aerospace Applications: Engine sensor housings, housings for components in hot zones, structural components where weight is critical, airframe sensor mounts, high-performance UAV components, space applications requiring low outgassing and temperature resistance.
- Sınırlamalar:
- Higher material cost compared to stainless steel or aluminum alloys.
- More challenging to machine than steel due to its reactivity and low thermal conductivity.
- Requires careful control during AM processing (often in inert atmospheres or vacuum) to prevent oxygen/nitrogen pickup, which can embrittle the material.
Material Properties Overview: Ti-6Al-4V (Typical AM, Stress Relieved)
Mülkiyet | Tipik Değer | Significance for Sensor Housings |
---|---|---|
Yoğunluk | ~4,43 g/cm³ | Excellent weight savings potential |
Nihai Çekme Dayanımı | 900 - 1100 MPa | Very high structural integrity |
Akma Dayanımı | 800 - 1000 MPa | High resistance to permanent deformation |
Kopma Uzaması | 10 – 18% | Sufficient ductility for most applications |
Sertlik (HRC) | ~30-36 HRC | İyi aşınma direnci |
Max Service Temp. | ~400°C (Continuous) | Suitable for higher temperature applications |
Korozyon Direnci | Olağanüstü | Ideal for highly corrosive or marine environments |
Export to Sheets
Choosing Between 316L and Ti-6Al-4V:
The selection depends on the specific requirements of the sensor housing:
- Choose 316L when:
- Cost is a major driver.
- Extreme weight saving is not the primary goal.
- Operating temperatures are moderate (< 400°C).
- Good general corrosion resistance is sufficient.
- Choose Ti-6Al-4V when:
- Weight reduction is critical.
- High strength is required.
- Operating temperatures are elevated (up to ~400°C).
- Superior corrosion resistance is necessary.
- The higher cost is justified by performance gains.
Both 316L and Ti-6Al-4V are readily available from specialized metal tozu tedarikçileri like Met3dp, optimized for additive manufacturing processes. Consulting with an experienced AM provider like Met3dp can help engineers and procurement managers select the optimal material and process for their specific aerospace sensor protector needs, ensuring parts meet the demanding performance and quality standards of the industry.
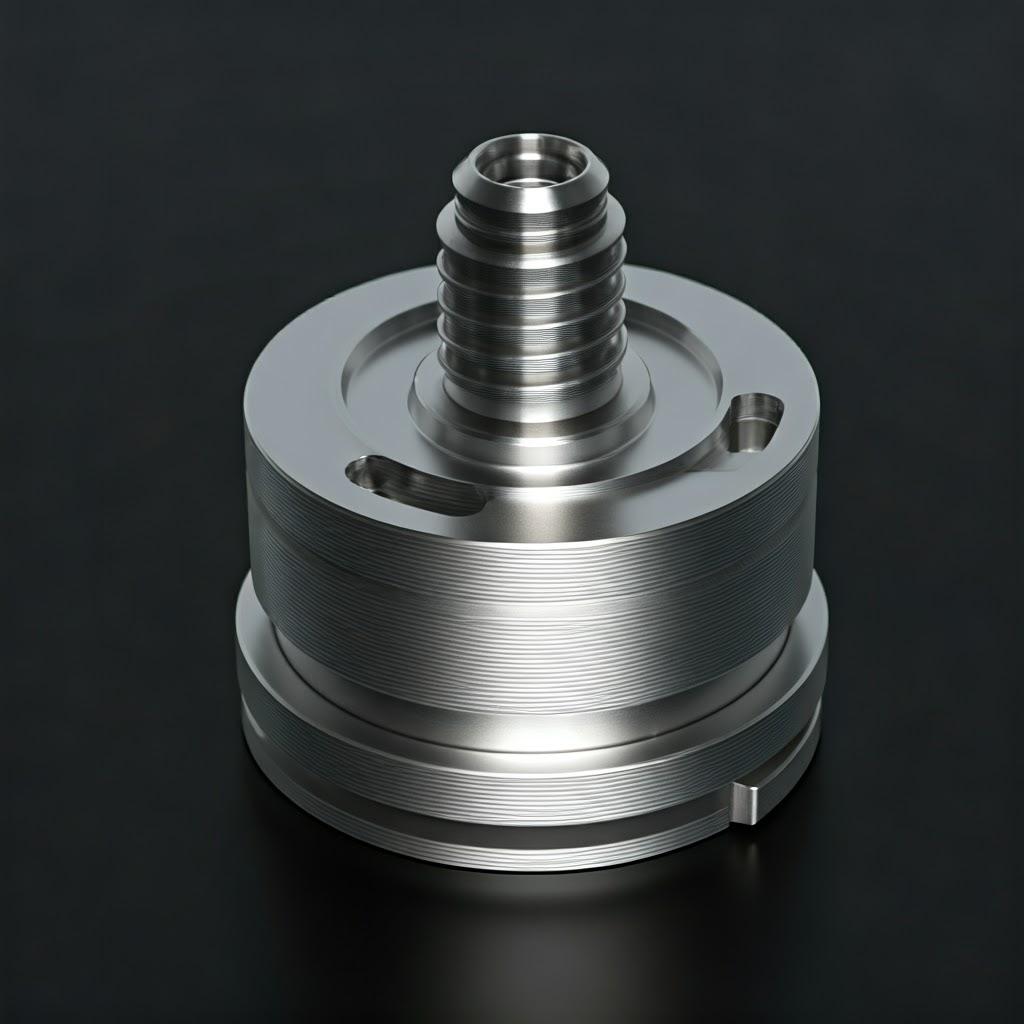
Design Considerations for Additive Manufacturing of Sensor Housings
Successfully leveraging metal additive manufacturing (AM) for aerospace sensor housings goes beyond simply replicating a design intended for machining. To truly harness the benefits of AM – lightweighting, part consolidation, enhanced functionality – engineers must embrace Katmanlı Üretim Tasarımı (DfAM) principles. DfAM involves rethinking component design to capitalize on the layer-by-layer build process while accounting for its unique constraints. For critical components like sensor protectors, thoughtful DfAM is essential for performance, reliability, and cost-effectiveness. Partnering with experienced B2B design services or AM providers like Met3dp, who possess deep DfAM expertise, can significantly streamline this process.
Key DfAM Principles for Sensor Housings:
- Topology Optimization:
- Concept: Using specialized software algorithms to iteratively remove material from areas of a design that do not experience significant stress, while adding material where it’s needed most. The result is an organic, often skeletal-looking structure that meets or exceeds the original strength requirements but with substantially less mass.
- Application for Sensor Housings: Ideal for mounting brackets, external support features, or the main body itself, especially when using high-strength materials like Ti-6Al-4V. It ensures the housing is robust enough to protect the sensor and withstand vibration/ G-loads, but without carrying unnecessary weight.
- Düşünceler: Optimized designs can be complex and difficult/impossible to manufacture traditionally. They often require careful orientation during the AM build process and potentially more complex support structures.
- Lattice Structures and Infill:
- Concept: Replacing solid sections of a part with internal, repeating geometric patterns (lattices). These structures can significantly reduce weight and material consumption while maintaining high compressive strength and stiffness. Different lattice types (e.g., cubic, octet-truss, gyroid) offer varying mechanical properties, thermal conductivity, and permeability.
- Application for Sensor Housings: Can be used internally within thicker walls or baseplates of the housing to reduce mass. Certain lattice types can also enhance vibration damping or allow for fluid/air flow if needed for thermal management or pressure equalization.
- Düşünceler: Lattice density and cell size must be carefully chosen based on load requirements and the resolution capabilities of the AM process. Ensuring powder removal from complex internal lattices can be challenging and requires specific design features (e.g., drain holes).
- Parça Konsolidasyonu:
- Concept: Redesigning an assembly of multiple components (e.g., housing body, lid, gasket groove, mounting bracket, fasteners) into a single, monolithic part.
- Application for Sensor Housings: Combining the housing body with its mounting features (brackets, flanges) eliminates fasteners and assembly steps, reducing weight, potential leak paths, and failure points. Integrating features like internal channels or sensor alignment guides becomes feasible.
- Düşünceler: Consolidated designs can be more complex to model initially. Access for internal post-processing (like machining critical surfaces) needs to be considered during the design phase.
- Optimizing for Build Orientation and Support Structures:
- Concept: The orientation in which a part is built significantly impacts surface finish, dimensional accuracy, build time, residual stress, and the amount/location of required support structures. Support structures are temporary features printed alongside the part to anchor overhanging sections and prevent warping.
- Application for Sensor Housings: Orient the part to minimize overhangs, especially on critical surfaces or internal features where support removal might be difficult. Self-supporting angles (typically >45 degrees from the horizontal) should be utilized where possible. Flat surfaces benefit from being oriented horizontally or vertically for better finish.
- Düşünceler: Support structures add to material cost and build time, and their removal requires post-processing effort, potentially leaving witness marks. Designing features to be self-supporting or minimizing steep overhangs is crucial. Met3dp’s engineers work closely with clients to determine optimal build orientation based on the specific geometry and functional requirements.
- Wall Thickness and Feature Size:
- Concept: AM processes have minimum achievable wall thicknesses and feature sizes dictated by the laser/electron beam spot size, powder particle size, and process physics.
- Application for Sensor Housings: Ensure walls are thick enough for structural integrity and to be reliably printed (typically >0.4-0.5 mm, depending on process and material). Small features like pins, bosses, or thin walls need careful consideration to ensure they resolve correctly.
- Düşünceler: Very thick sections can accumulate residual stress and increase print time/cost. Consider using internal lattices or topology optimization instead of large solid volumes.
- Incorporating Functional Features:
- Concept: Leveraging design freedom to embed functionality directly into the housing.
- Application for Sensor Housings:
- Conformal Cooling/Heating Channels: Design channels that precisely follow the sensor’s shape or critical electronic components for efficient thermal control.
- Integrated Wiring Channels/Conduits: Create internal pathways to route and protect wiring.
- Built-in Seals or Gasket Grooves: Design features for O-rings or other sealing mechanisms directly into the part.
- Text/Labeling: Emboss or engrave part numbers, serial numbers, or logos directly onto the surface during the build.
- Designing for Post-Processing:
- Concept: Anticipating required post-processing steps (machining, polishing, coating) during the design phase.
- Application for Sensor Housings: Add extra stock material (machining allowance) to surfaces requiring high precision or specific finishes. Ensure accessibility for tools if internal features need machining. Design features that facilitate handling during heat treatment or coating (e.g., temporary handling points). Incorporate access holes for powder removal from internal cavities or lattice structures.
By integrating these DfAM principles, engineers can move beyond simply replacing traditionally manufactured sensor housings and create next-generation components that are lighter, stronger, more functional, and often produced faster and more cost-effectively, especially for complex or customized designs.
Achievable Tolerance, Surface Finish, and Dimensional Accuracy in Metal AM Sensor Housings
A common question from engineers and procurement managers evaluating metal AM concerns the level of precision achievable. While metal 3D printing creates near-net-shape parts, it’s crucial to understand the typical tolerances, surface finishes, and dimensional accuracy inherent to the process and how post-processing can refine these attributes to meet stringent aerospace requirements. Relying on a precision manufacturing supplier with calibrated equipment and robust quality control processes, like Met3dp, is vital.
1. Dimensional Accuracy: * As-Built Accuracy: The dimensional accuracy of a part directly after printing (and stress relief, if applied) depends on several factors: * AM Süreci: Farklı baskı yöntemleri (e.g., LPBF, SEBM) have slightly different typical accuracies. SEBM, often used by Met3dp for materials like Ti-6Al-4V, generally produces parts with lower internal stresses due to the higher build chamber temperature, potentially improving overall dimensional stability. * Malzeme: Thermal expansion and contraction characteristics vary between materials (e.g., Ti-6Al-4V vs. 316L). * Part Size & Geometry: Larger parts or those with complex geometries and varying cross-sections are more susceptible to thermal distortion during the build. * Build Orientation & Supports: Strategic orientation and effective support structures help mitigate warping and ensure features form correctly. * Machine Calibration: Regular calibration of the AM system is essential for consistent accuracy. * Typical Values: As a general guideline, as-built tolerances for metal AM are often in the range of ±0.1 mm to ±0.3 mm or ±0.1% to ±0.2% of the nominal dimension, whichever is greater. However, this can vary significantly. Critical dimensions should always be discussed with the AM provider. * Achieving Tighter Tolerances: For features requiring higher precision (e.g., mating interfaces, sensor mounting bores, sealing surfaces), post-process CNC machining is typically employed. Tolerances achievable via machining are much tighter, often down to ±0.01 mm or better, depending on the operation.
2. Surface Finish (Roughness): * As-Built Surface Finish: The surface finish of AM parts is inherently rougher than machined surfaces due to the layer-by-layer process and the partially melted powder particles adhering to the surface. It is typically measured using Ra (average roughness). * Factors Influencing Ra: * Yapı Oryantasyonu: Surfaces built parallel to the build plate (upward/downward-facing) tend to be smoother than vertical walls. Angled surfaces, especially shallow “stair-stepped” ones, are often the roughest. Support contact points also leave rougher areas (witness marks) after removal. * Katman Kalınlığı: Thinner layers generally result in a smoother finish but increase build time. * Toz Özellikleri: Finer, more spherical powders (like those produced using Met3dp’s advanced atomization) can contribute to better surface finishes. * Process Parameters: Laser/beam power, scan speed, etc., influence the melt pool dynamics and resulting surface texture. * Typical Values (Ra): As-built surface roughness for metal AM typically ranges from 5 µm to 25 µm Ra. Downward-facing surfaces requiring support are often rougher. * Yüzey İşleminin İyileştirilmesi: Various post-processing techniques can significantly improve the surface finish: * Abrasive Blasting (Bead/Sand Blasting): Provides a uniform matte finish, typically reducing Ra to 3-10 µm. Good for general smoothing and cleaning. * Tumbling/Vibratory Finishing: Uses abrasive media in a tumbling barrel or vibratory bowl to smooth surfaces and deburr edges. Can achieve Ra values down to 1-5 µm. * CNC Machining: Used for critical surfaces requiring very smooth finishes (Ra < 1 µm possible). * Polishing (Manual or Automated): Can achieve mirror-like finishes (Ra < 0.1 µm) but is often labor-intensive and reserved for specific areas. * Electropolishing: An electrochemical process that removes a thin layer of material, resulting in a very smooth, clean, and often more corrosion-resistant surface, particularly effective on stainless steels like 316L.
3. Quality Control in Aerospace: * Importance: Ensuring dimensional accuracy and surface finish meet specifications is critical for aerospace components. Failure to meet tolerance can prevent proper assembly, affect sensor alignment, or compromise sealing. Poor surface finish can impact fatigue life or aerodynamic performance. * Metrology: Advanced metrology techniques are used to verify dimensions and roughness: * Coordinate Measuring Machines (CMMs): High-precision measurement of complex geometries. * 3D Scanning (Laser/Structured Light): Captures the full part geometry for comparison against the original CAD model. * Surface Profilometers: Measure surface roughness (Ra, Rz, etc.). * Supplier Capability: Choosing an AM supplier with robust quality control aerospace procedures, including process monitoring, material traceability, dimensional inspection, and NDT (Non-Destructive Testing) capabilities (like CT scanning for internal defects), is essential.
Summary Table: Precision in Metal AM Sensor Housings
Parametre | As-Built Typical Range | Post-Processed Potential | Önemli Hususlar |
---|---|---|---|
Dimensional Tol. | ±0.1 to ±0.3 mm / ±0.1-0.2% | Down to ±0.01 mm (Machining) | Process, material, size, geometry, machine calibration |
Surface Finish (Ra) | 5 µm to 25 µm | < 1 µm (Machining/Polishing) | Orientation, layer thickness, supports, post-process |
1-5 µm (Tumbling) | |||
3-10 µm (Blasting) |
Export to Sheets
Engineers designing sensor housings should clearly define critical tolerances and surface finish requirements on their drawings, indicating which features require post-processing to achieve the necessary precision beyond the standard as-built capabilities of metal AM.
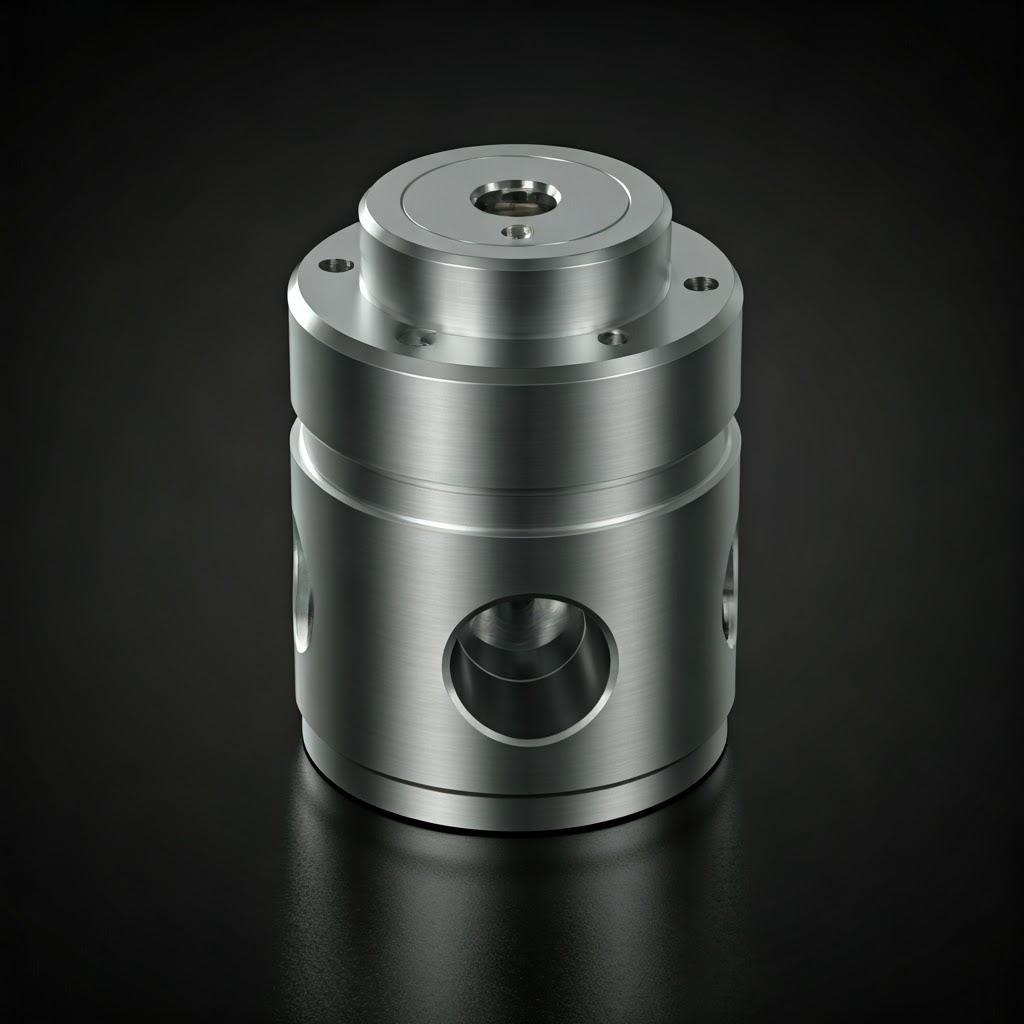
Essential Post-Processing Requirements for Aerospace Sensor Protectors
Metal additive manufacturing rarely produces a finished part straight out of the machine, especially for demanding aerospace applications. A series of post-processing steps are typically required to transform the near-net-shape printed component into a functional, flight-ready sensor housing. These steps enhance mechanical properties, achieve critical tolerances and surface finishes, remove temporary structures, and ensure compliance with aerospace standards. Engaging with suppliers offering comprehensive component finishing services is often necessary.
Common Post-Processing Steps:
- Stress Relief / Heat Treatment:
- Amacımız: The rapid heating and cooling cycles inherent in AM processes (especially LPBF) induce internal stresses within the printed part. These stresses can lead to distortion or cracking during or after the build, or compromise the part’s mechanical performance and fatigue life. Heat treatment relieves these residual stresses and can also homogenize the microstructure and optimize mechanical properties (e.g., strength, ductility, hardness) according to material specifications (like AMS standards for Ti-6Al-4V or 316L).
- Süreç: Parts are typically heated in a controlled atmosphere (vacuum or inert gas like argon) furnace to a specific temperature (below the annealing or solutionizing temperature), held for a duration, and then slowly cooled. Specific cycles depend on the material (e.g., Ti-6Al-4V often undergoes stress relief around 650-800°C, sometimes followed by aging treatments). SEBM processes, operating at higher temperatures, inherently reduce stress compared to LPBF, potentially simplifying heat treatment needs.
- Requirement: Virtually always required for critical aerospace components to ensure dimensional stability and optimal mechanical performance.
- Part Removal from Build Plate:
- Amacımız: Separating the printed part(s) from the metal build plate they were fused to during the AM process.
- Yöntemler: Typically done using wire EDM (Electrical Discharge Machining) or band sawing. Care must be taken to avoid damaging the part.
- Destek Yapısının Kaldırılması:
- Amacımız: Removing the temporary support structures printed to anchor overhangs and dissipate heat.
- Yöntemler: Supports are usually designed with weaker connection points to the main part. Removal can be done manually (using pliers, cutters), via CNC machining, or sometimes using electrochemical methods. This step can be labor-intensive, especially for complex internal supports. Access for removal must be considered during the DfAM phase. Witness marks where supports were attached often require further finishing.
- Powder Removal:
- Amacımız: Ensuring all unfused metal powder is removed from the part, especially from internal channels, cavities, or lattice structures. Trapped powder can add weight, potentially become loose debris, or interfere with function.
- Yöntemler: Compressed air blow-off, vibration, ultrasonic cleaning, and specialized powder removal systems. Designing adequate drainage/access holes during DfAM is crucial for effective powder removal from internal features.
- Yüzey İşlemi:
- Amacımız: Achieving the required surface roughness (Ra) for functional requirements (e.g., sealing surfaces, aerodynamic smoothness) or cosmetic appearance.
- Yöntemler: As discussed previously – bead blasting, tumbling, machining, polishing, electropolishing. The method(s) chosen depend on the target Ra value, material, geometry, and cost considerations.
- CNC Machining:
- Amacımız: Achieving tight tolerances on critical dimensions (mating faces, bore diameters, threads) that cannot be met by the as-built AM process. Also used to create very smooth surface finishes on specific features.
- Süreç: Transferring the AM part to a CNC milling machine or lathe to precisely machine designated features. Requires careful fixture design to hold the often complex AM part accurately. Adding machining stock during the DfAM stage is essential.
- Cleaning & Inspection:
- Amacımız: Ensuring the part is free from contaminants (powder residue, cutting fluids, handling debris) and meets all dimensional, surface, and structural integrity specifications.
- Yöntemler: Ultrasonic cleaning, visual inspection, dimensional inspection (CMM, 3D scanning), surface roughness measurement, NDT (e.g., Fluorescent Penetrant Inspection (FPI) for surface crack detection, CT scanning for internal void detection). Aerospace certification requirements often mandate specific inspection protocols.
- Optional Coating or Treatments:
- Amacımız: Enhancing specific properties like wear resistance, corrosion resistance, thermal barrier, or electrical insulation.
- Yöntemler:
- Anodizing (for Titanium): Creates a hard, wear-resistant oxide layer, can be colored for identification.
- Passivation (for Stainless Steel): Enhances corrosion resistance by removing free iron and promoting the formation of the passive oxide layer.
- Specialized Coatings: PVD/CVD coatings for wear resistance, thermal barrier coatings (TBCs) for high-temperature applications, chemical conversion coatings for corrosion protection.
The specific sequence and necessity of these post-processing steps depend heavily on the chosen material, AM process, part complexity, and the final application requirements defined by the aerospace customer.
Common Challenges in 3D Printing Sensor Housings and Mitigation Strategies
While metal AM offers significant advantages, it is not without its challenges, particularly when producing high-precision, critical components like aerospace sensor housings. Understanding these potential issues and implementing effective mitigation strategies during design, simulation, printing, and post-processing is key to achieving successful outcomes. Effective troubleshooting metal AM requires expertise and process control.
Challenge 1: Warping and Distortion * Cause: Uneven heating and cooling during the layer-wise fusion process create internal residual stresses. If these stresses exceed the material’s yield strength or the anchoring force of supports, the part can warp or distort, deviating from the intended geometry. This is more common in large parts, thin features, or materials with high thermal expansion. * Mitigation Strategies: * Thermal Simulation: Using FEA software to predict stress accumulation and distortion before printing. * Optimized Build Orientation: Orienting the part to minimize large flat areas parallel to the build plate and reduce thermal gradients across layers. * Robust Support Structures: Designing sufficient supports to anchor the part firmly to the build plate and manage thermal stresses. Specific support types (e.g., block, conical) can be chosen based on simulation results. * Süreç Parametre Optimizasyonu: Fine-tuning laser/beam power, scan speed, and scan strategy (e.g., checkerboard pattern) to manage heat input. * Stress Relief Heat Treatment: Applying appropriate heat treatment immediately after printing to relax internal stresses before support removal. * Process Choice: SEBM, with its heated build chamber, often results in lower residual stress compared to LPBF, particularly beneficial for crack-sensitive or large titanium parts.
Challenge 2: Porosity Control * Cause: Small voids or pores can form within the printed material due to gas entrapment (dissolved gases in the powder or shielding gas) or lack-of-fusion between layers or scan tracks. Porosity can degrade mechanical properties, particularly fatigue life. * Mitigation Strategies: * High-Quality Powder: Using powders with low internal gas porosity, controlled particle size distribution, and high sphericity/flowability (like Met3dp’s Gas Atomized or PREP powders). Proper powder handling and storage are crucial to prevent moisture pickup. See Met3dp’s product offerings. * Optimized Process Parameters: Developing robust parameter sets (power, speed, layer thickness, hatch spacing) validated to achieve >99.5% density for the specific material and machine. * Controlled Atmosphere: Maintaining a high-purity inert gas atmosphere (Argon or Nitrogen for LPBF) or vacuum (SEBM) to minimize contamination and gas pickup during printing. * Sıcak İzostatik Presleme (HIP): A post-processing step where the part is subjected to high temperature and isostatic pressure, effectively closing internal pores and achieving near 100% density. Often required for critical fatigue-limited aerospace parts. * NDT Inspection: Using CT scanning to detect and quantify internal porosity.
Challenge 3: Support Removal Difficulties & Surface Quality Impacts * Cause: Supports are necessary but can be difficult and time-consuming to remove, especially from complex internal geometries or delicate features. Removal processes can potentially damage the part or leave undesirable witness marks on the surface. * Mitigation Strategies: * DfAM for Reduced Supports: Designing parts with self-supporting angles (>45°) and minimizing overhangs where possible. * Optimized Support Design: Using specialized support generation software to create supports that are strong enough during the build but easier to remove (e.g., perforated supports, supports with smaller contact points). Orienting the part to place supports on non-critical surfaces. * Accessible Design: Ensuring adequate access for tools or processes needed for support removal, particularly for internal channels (requires careful DfAM). * Appropriate Removal Techniques: Selecting the right method (manual, machining, EDM) based on support location, part material, and feature delicacy. * Yüzey İşlemi: Planning for subsequent finishing steps (blasting, tumbling, machining) to remove support witness marks from critical areas.
Challenge 4: Powder Contamination Prevention * Cause: Cross-contamination between different metal powders can significantly alter the alloy composition and degrade material properties. Contamination can occur during powder loading, unloading, recycling, or if machines are not thoroughly cleaned between builds with different materials. * Mitigation Strategies: * Dedicated Machines (Ideal): Using separate AM machines dedicated to specific materials or material families (e.g., one for Titanium, one for Steel). * Rigorous Cleaning Protocols: Implementing strict cleaning procedures for the build chamber, powder handling equipment, and sieving stations when changing materials. * Powder Lot Traceability & Testing: Maintaining careful tracking of powder batches and performing chemical analysis to verify composition before use. * Controlled Powder Handling Environment: Managing powder in controlled humidity environments to prevent moisture pickup.
Challenge 5: Achieving Tight Tolerances and Surface Finish * Cause: As discussed, the inherent nature of AM results in tolerances and surface finishes that may not meet all aerospace requirements directly. * Mitigation Strategies: * Realistic Expectations: Understanding the as-built capabilities of the chosen AM process and material. * DfAM for Post-Processing: Designing parts with machining allowances on critical surfaces. * Integrated Manufacturing Approach: Planning for and utilizing appropriate post-processing steps (machining, polishing, etc.) as an integral part of the manufacturing workflow. * Clear Specification: Clearly defining tolerance and finish requirements on drawings and collaborating closely with the AM service provider.
Addressing these challenges requires a combination of design expertise, material science knowledge, process control, robust quality assurance, and effective post-processing capabilities. Partnering with an experienced provider like Met3dp, who understands these nuances and has implemented mitigation strategies throughout their workflow, is crucial for successfully manufacturing reliable aerospace sensor housings via metal AM.
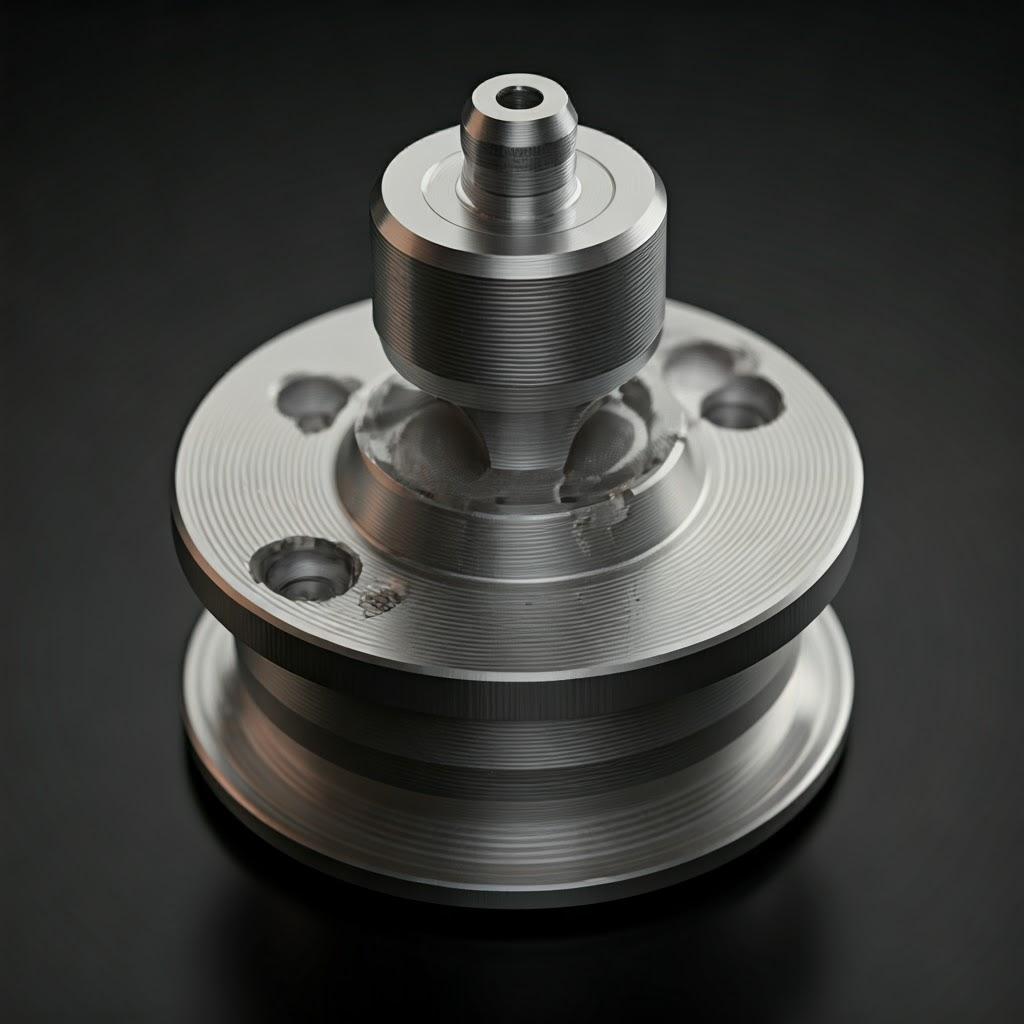
How to Choose the Right Metal 3D Printing Service Provider for Aerospace Components
Selecting the right additive manufacturing (AM) partner is as critical as the technology itself, especially when dealing with aerospace components like sensor housings where performance, reliability, and traceability are non-negotiable. The right AM supplier acts as more than just a parts printer; they are a technology partner offering expertise from design through to finished, certified components. For procurement managers and engineers navigating the AM landscape, here are key criteria for evaluating potential service providers:
1. Aerospace Certifications and Quality Management System (QMS): * Requirement: This is often the primary filter. Look for providers holding relevant aerospace certifications, most notably AS9100. This certification demonstrates a robust QMS specifically tailored to the stringent requirements of the aerospace industry, covering aspects like process control, traceability, risk management, and continuous improvement. ISO 9001 is a foundational quality standard, but AS9100 is the aerospace benchmark. * Verification: Ask for proof of certification and understand the scope. Ensure their QMS covers the specific AM processes (e.g., LPBF, SEBM) and materials (e.g., Ti-6Al-4V, 316L) you require.
2. Technical Expertise and Engineering Support: * Requirement: The provider should possess deep technical knowledge of AM processes, materials science, and Design for Additive Manufacturing (DfAM). Look for a team with experienced engineers who can provide valuable input on design optimization, material selection, build orientation strategy, support structure generation, and post-processing requirements. * Evaluation: Review case studies, inquire about their team’s background and experience (especially with aerospace applications), and assess their willingness and ability to collaborate on design challenges. Do they offer DfAM consultation? Can they perform thermal or stress simulations? Met3dp prides itself on its decades of collective expertise in metal AM, offering comprehensive uygulama geliştirme hizmetleri alongside printing.
3. Equipment Capabilities and Technology: * Requirement: The provider should operate state-of-the-art, industrial-grade AM equipment suitable for the materials and precision required. This includes not just the printers themselves but also necessary ancillary equipment for powder handling, post-processing (heat treatment furnaces, CNC machines), and quality inspection (CMMs, NDT equipment). * Evaluation: Inquire about their specific machine models, build envelope sizes, and maintenance/calibration schedules. Do they offer the optimal technology for your material (e.g., SEBM for low-stress Titanium parts)? Met3dp utilizes industry-leading printers, including advanced SEBM systems, known for accuracy and reliability in producing mission-critical aerospace parts.
4. Material Expertise and Traceability: * Requirement: A top-tier provider will have proven expertise with the specific aerospace alloys you need (e.g., Ti-6Al-4V, 316L, Inconel). Crucially, they must have rigorous procedures for material handling, storage, testing, and traceability, ensuring that the powder used meets specifications and its lineage can be traced back to the source. This includes managing powder recycling and preventing cross-contamination. * Evaluation: Ask about their powder sourcing (do they manufacture their own high-quality powders, like Met3dp?), powder testing procedures (chemistry, particle size distribution, flowability), and how they document material traceability throughout the production process.
5. Post-Processing Capabilities: * Requirement: Since most aerospace AM parts require significant post-processing, an ideal supplier will offer a wide range of in-house or tightly managed external capabilities. This includes stress relief/heat treatment, support/powder removal, CNC machining, surface finishing (blasting, tumbling, polishing), and NDT inspection. * Evaluation: Determine which post-processing steps they perform in-house versus outsource. In-house capabilities often allow for better control over quality, lead times, and cost. Assess their expertise in machining complex AM parts and their range of surface finishing options.
6. Proven Track Record and Case Studies: * Requirement: Look for evidence of successful projects, particularly within the aerospace sector or involving similar components (e.g., complex housings, high-precision parts). * Evaluation: Request relevant case studies, testimonials, or references. An established provider should be able to demonstrate their ability to deliver high-quality parts that meet demanding specifications.
7. Lead Time Guarantees and Communication: * Requirement: While AM enables rapid prototyping, understanding realistic production lead times, including post-processing, is crucial for project planning. The provider should offer clear communication, provide reliable lead time estimates, and keep you informed of progress. * Evaluation: Discuss typical lead times for parts of similar complexity and material. Evaluate their responsiveness and communication style during the quoting process.
8. Cost-Effectiveness (Value, Not Just Price): * Requirement: While cost is always a factor, the focus should be on overall value. The cheapest quote may not account for robust quality control, necessary post-processing, or expert engineering support, potentially leading to higher costs down the line due to delays or part failures. * Evaluation: Ensure quotes are comprehensive, detailing all included steps (printing, materials, supports, heat treatment, basic finishing, QC). Compare quotes based on the provider’s capabilities, certifications, and expertise, not just the bottom-line price.
Choosing the right AM service provider is a strategic decision. By carefully evaluating potential partners against these criteria, aerospace companies can build relationships with suppliers like Met3dp who possess the necessary certifications, technological capabilities, material expertise, and commitment to quality required to successfully implement metal additive manufacturing for critical components like sensor housings.
Cost Factors and Lead Time Estimation for AM Aerospace Sensor Housings
Understanding the factors that influence the cost and lead time of metal additive manufacturing is essential for effective aerospace component procurement and project budgeting. While AM offers potential cost savings compared to traditional methods for complex or low-volume parts, it’s important to recognize the key drivers.
Key Cost Factors:
- Material Type and Consumption:
- Malzeme Maliyeti: High-performance aerospace alloys like Ti-6Al-4V or nickel superalloys are inherently more expensive than materials like 316L stainless steel. The raw powder cost significantly impacts the final price. Met3dp’s advanced powder production aims for quality and consistency, which is factored into the value.
- Part Volume & Density: The actual volume of material used to print the part directly correlates with cost. Larger or denser parts consume more powder.
- Destek Yapıları: Material used for support structures also adds to the cost. Optimized designs minimizing supports can reduce material consumption.
- Powder Recycling Efficiency: While unfused powder is often recycled, some losses occur during handling and processing, slightly impacting overall material cost efficiency.
- Machine Time (Build Time):
- Part Volume & Height: Larger volume parts or taller parts (requiring more layers) take longer to print, directly increasing the machine time cost component. Industrial AM machines represent significant capital investment, and their hourly operating rate is a major cost driver.
- Part Complexity & Features: Very intricate details or thin walls may require slower printing speeds or specific scan strategies, potentially increasing build time.
- Nesting & Build Density: Service providers aim to “nest” multiple parts efficiently within a single build volume to maximize machine utilization and reduce per-part cost. The ability to fill a build plate influences cost, making toplu sipariş fiyatlandırması potentially more favorable.
- Labor and Engineering:
- File Preparation & Setup: Time spent by engineers to review the CAD file, optimize orientation, generate support structures, and prepare the build layout contributes to the cost.
- Machine Operation: Labor involved in setting up the machine, loading powder, monitoring the build, and unloading parts.
- DfAM Support: If significant design consultation or optimization is required, this engineering time will be factored in.
- İşlem Sonrası Gereksinimler:
- Complexity & Extent: This can be a significant cost component. Extensive support removal, mandatory heat treatments (especially complex cycles or HIP), high-precision CNC machining on multiple surfaces, advanced surface finishing (polishing, electropolishing), and required coatings all add substantially to the final price.
- Labor Intensity: Many post-processing steps, like manual support removal or polishing, are labor-intensive.
- Quality Control and Inspection:
- Level of Scrutiny: Basic dimensional checks are standard, but comprehensive inspection required for aerospace (e.g., CMM reports on dozens of features, NDT like CT scanning or FPI) adds significant cost due to specialized equipment and technician time.
- Certification & Documentation: Generating material certifications, certificates of conformity, and detailed inspection reports requires administrative time.
- Overheads and Provider Markup:
- Includes facility costs, machine depreciation, software licenses, administrative overhead, and provider profit margin.
Lead Time Estimation:
Lead time encompasses the entire process from order placement to shipment of the finished part.
- Typical Components:
- Quoting & Order Processing: 1-5 days
- File Preparation & Scheduling: 1-3 gün
- Printing (Build Time): Highly variable – hours (for small parts) to several days or even weeks (for very large parts or full build plates).
- Cool Down & Depowdering: 1 day
- Isıl İşlem: 1-3 days (including furnace time and cooling)
- Support Removal & Basic Finishing (e.g., Blasting): 1-3 gün
- İşleme: 2-10 days (highly dependent on complexity and machine availability)
- Advanced Finishing/Coating: 2-7 days
- Inspection & Shipping: 1-3 gün
- Estimated Total Lead Time: For a moderately complex aerospace sensor housing requiring standard post-processing (heat treatment, blasting, some machining), typical lead times might range from 2 ila 6 hafta. Prototyping with minimal post-processing can sometimes be faster (1-2 weeks), while highly complex parts requiring extensive machining and multiple finishing steps could extend beyond 6 weeks.
- Factors Influencing Lead Time: Part complexity, build time, nesting opportunities, provider’s current backlog, extent of post-processing, and required inspection levels all impact the final delivery schedule. Expedited services are often available at a premium cost.
Clear communication with the AM service provider regarding both cost expectations and lead time requirements is crucial for successful project execution. Providing detailed drawings, specifications, and desired quantities allows the provider to generate accurate quotes and realistic timelines.
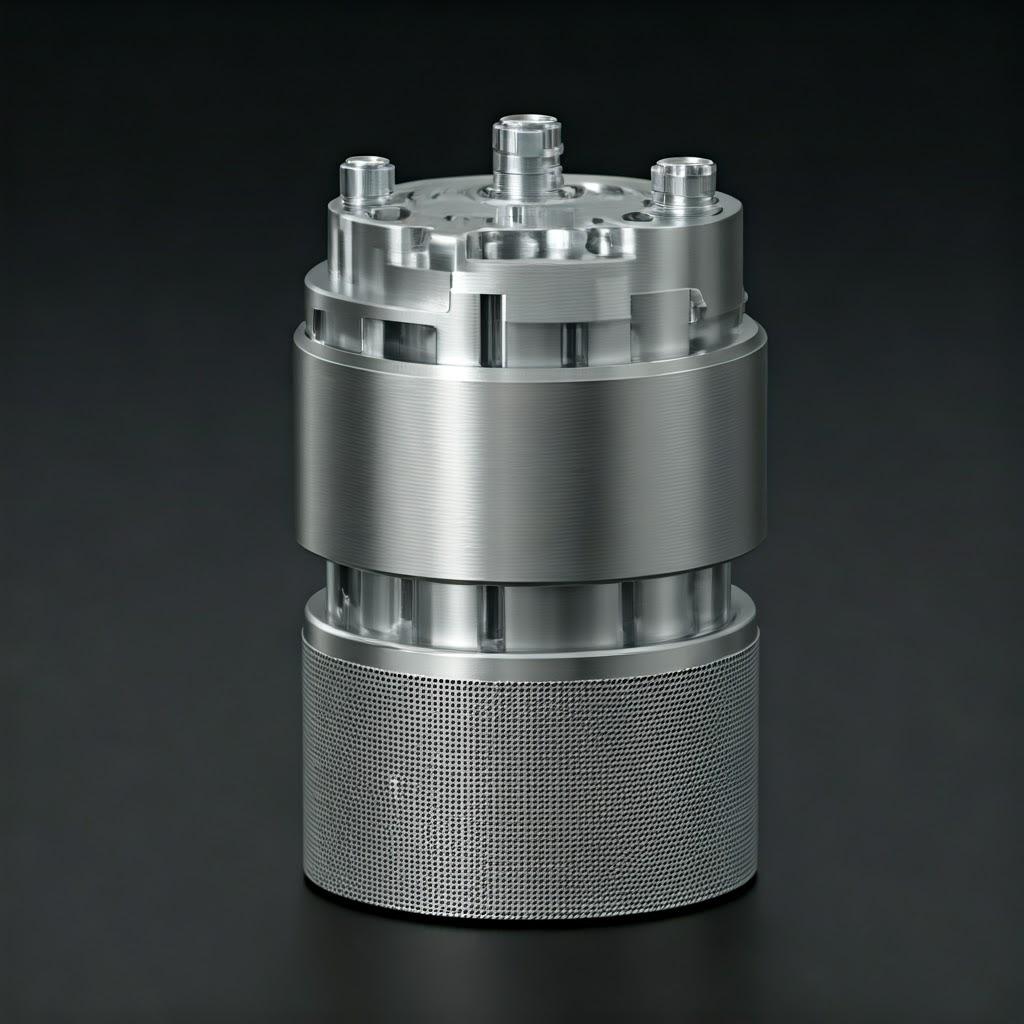
Frequently Asked Questions (FAQ) about 3D Printed Aerospace Sensor Housings
Here are answers to some common questions engineers and procurement managers have about using metal AM for aerospace sensor housings:
1. Are 3D printed metal sensor housings as strong and durable as machined ones? * Cevap: Yes, when processed correctly, metal AM parts can meet or even exceed the mechanical properties of wrought or cast counterparts. Key factors include using high-quality, aerospace-grade powders (like Ti-6Al-4V or 316L from reputable suppliers like Met3dp), optimizing AM process parameters to achieve full density (>99.5%), and applying appropriate post-process heat treatments to relieve stress and achieve the desired material temper (meeting AMS or other relevant specifications). Processes like Hot Isostatic Pressing (HIP) can be used to eliminate any remaining micro-porosity for fatigue-critical applications, resulting in properties equivalent to wrought materials. The layer-wise nature does introduce some anisotropy (direction-dependent properties), which experienced AM engineers account for during design and orientation planning.
2. Can 3D printed housings meet the strict material certification and traceability requirements of the aerospace industry? * Cevap: Absolutely. Established AM service providers catering to the aerospace industry, particularly those with AS9100 certification like Met3dp aspires to exemplify, have rigorous quality management systems in place. This includes: * Powder Certification: Sourcing powder from qualified suppliers with batch-specific chemical and physical analysis, or producing powders in-house under strict controls. * Material Traceability: Documenting the specific powder batch used for each build and maintaining traceability throughout the manufacturing process. * Süreç Kontrolü: Monitoring and logging critical process parameters during the build. * Test: Performing tensile tests, chemical analysis, and microstructural evaluation on representative samples built alongside the parts. * Documentation: Providing comprehensive material certifications and certificates of conformity with each shipment, meeting standards like AMS or customer-specific requirements.
3. How does the cost of a 3D printed sensor housing compare to CNC machining, especially for low volumes or complex designs? * Cevap: The cost comparison depends heavily on part complexity, volume, and material. * For simple geometries and high volumes: CNC machining is often still more cost-effective due to faster cycle times per part once set up. * For complex geometries: Metal AM becomes highly competitive, even at low volumes. Features like internal channels, lattice structures, or topology-optimized shapes are extremely difficult or impossible to machine, making AM the enabling technology or significantly cheaper than multi-part machined assemblies. * For low volumes (including prototypes and single units): AM avoids the high initial setup and tooling costs associated with casting and the programming/fixturing costs of complex machining setups, making it very cost-effective for producing small quantities or prototypes rapidly. * Malzeme atıkları: AM’s lower buy-to-fly ratio can also lead to cost savings, especially with expensive materials like titanium. * General Guideline: For one-offs or very small batches (<10-20 units) of complex sensor housings, AM is often more economical. For simpler designs in larger quantities, machining may be cheaper. A detailed quote comparison is always recommended.
Conclusion: Elevating Aerospace Sensor Protection with Met3dp’s Additive Manufacturing Expertise
The aerospace industry operates at the pinnacle of performance and safety, demanding components that withstand extreme conditions without failure. Protective sensor housings, though often small, play a vital role in ensuring the reliability of critical data acquisition systems. As we’ve explored, metal katkılı üretim offers a powerful suite of tools to revolutionize how these essential components are designed and produced.
By embracing AM technologies like Selective Electron Beam Melting (SEBM) and Laser Powder Bed Fusion (LPBF), and utilizing high-performance materials such as Ti-6Al-4V ve 316L, aerospace engineers can create sensor housings that are:
- Lighter: Through topology optimization and lattice structures, reducing overall aircraft weight.
- More Functional: Integrating complex features like conformal cooling channels or consolidated mounting points.
- Produced Faster: Especially during prototyping and for low-volume, on-demand requirements.
- Highly Customized: Tailored precisely to specific sensor geometries and operational needs.
However, realizing these benefits requires navigating the intricacies of DfAM, understanding material properties, controlling process parameters, implementing thorough post-processing, and ensuring stringent quality control. This is where partnering with a knowledgeable and capable AM provider becomes paramount.
Met3dp, headquartered in Qingdao, China, is a leader in metal additive manufacturing, offering a comprehensive ecosystem of solutions tailored for demanding industries like aerospace. Our capabilities include:
- Advanced AM Systems: Industry-leading SEBM printers delivering exceptional accuracy and reliability for mission-critical parts.
- Yüksek Kaliteli Metal Tozları: Manufacturing superior spherical powders (including Ti-6Al-4V, 316L, CoCrMo, TiNi, TiTa, superalloys, and more) using cutting-edge Gas Atomization and PREP technologies.
- End-to-End Services: Providing expert support from DfAM consultation and simulation through printing, post-processing, and quality assurance.
- Deep Expertise: Decades of collective experience in metal AM application development.
We are committed to helping aerospace manufacturers leverage the transformative potential of additive manufacturing to produce next-generation sensor housings and other critical components. Whether you are exploring AM for the first time or seeking a reliable aerospace component supplier for ongoing production, Met3dp has the technology, materials, and expertise to support your goals.
Elevate your sensor protection solutions and embrace the future of aerospace manufacturing. Contact Met3dp today to discuss your project requirements and discover how our additive manufacturing capabilities can accelerate your innovation and enhance your component performance.
Paylaş
Facebook
Twitter
LinkedIn
WhatsApp
E-posta
MET3DP Technology Co, LTD, merkezi Qingdao, Çin'de bulunan lider bir katmanlı üretim çözümleri sağlayıcısıdır. Şirketimiz, endüstriyel uygulamalar için 3D baskı ekipmanları ve yüksek performanslı metal tozları konusunda uzmanlaşmıştır.
İşletmeniz için en iyi fiyatı ve özelleştirilmiş Çözümü almak için sorgulayın!
İlgili Makaleler
Met3DP Hakkında
Son Güncelleme
Bizim Ürünümüz
BİZE ULAŞIN
Herhangi bir sorunuz var mı? Bize şimdi mesaj gönderin! Mesajınızı aldıktan sonra tüm ekibimizle talebinize hizmet edeceğiz.
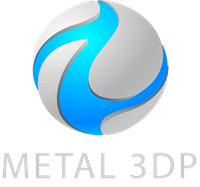
3D Baskı ve Katmanlı Üretim için Metal Tozları
ÜRÜN
cONTACT BİLGİLERİ
- Qingdao Şehri, Shandong, Çin
- [email protected]
- [email protected]
- +86 19116340731