3D-gedruckte Drohnenschalen aus leichtem Aluminium
Inhaltsübersicht
Einleitung: Die entscheidende Rolle fortschrittlicher Drohnenhüllen für die Leistung von UAVs
Der Himmel wird zunehmend bevölkert. Unbemannte Luftfahrzeuge (UAVs), gemeinhin als Drohnen bekannt, haben ihren Ursprung in militärischen Nischenanwendungen hinter sich gelassen und sind zu unverzichtbaren Werkzeugen in einem breiten Spektrum von Branchen geworden. Von der Aufnahme atemberaubender Filmaufnahmen und der Lieferung wichtiger medizinischer Güter bis hin zur Überwachung riesiger landwirtschaftlicher Felder und der Inspektion komplexer Infrastrukturen - Drohnen verändern die betriebliche Effizienz und eröffnen völlig neue Möglichkeiten. Im Mittelpunkt dieser Revolution steht das ständige Streben nach höherer Leistung: längere Flugzeiten, größere Nutzlastkapazitäten, verbesserte Manövrierfähigkeit und höhere Haltbarkeit. Entscheidend für diese Fortschritte ist die Hülle oder das Flugwerk der Drohne - das Skelett und die Haut, die ihre Fähigkeiten definieren.
Die Hülle der Drohne ist weit mehr als nur eine äußere Hülle. Sie erfüllt mehrere wichtige Funktionen:
- Strukturelle Integrität: Sie bildet das Kerngerüst, das alle Komponenten, einschließlich Motoren, Batterien, Sensoren, Navigationssysteme und Nutzlasten, trägt. Sie muss den Belastungen von Flug, Start, Landung und Umweltfaktoren standhalten.
- Aerodynamischer Wirkungsgrad: Die Form und die Oberfläche der Hülle haben einen erheblichen Einfluss auf den Luftwiderstand, den Auftrieb und die Stabilität, was sich direkt auf die Flugdauer, die Geschwindigkeit und den Batterieverbrauch auswirkt. Eine optimierte Aerodynamik ist entscheidend für die Maximierung der Leistung.
- Schutz von Bauteilen: Die Hülle schützt die empfindliche Elektronik und die Nutzlasten vor Umwelteinflüssen wie Staub, Feuchtigkeit, Stößen und Temperaturschwankungen.
- Integration der Nutzlast: Es bietet Befestigungspunkte und Platz für Kameras, Sensoren, Liefermechanismen und andere missionsspezifische Ausrüstung, die eine präzise Konstruktion und robuste Unterstützung erfordert.
- Wärmemanagement: Bei Hochleistungsdrohnen kann die Hülle dazu beitragen, die von den Motoren und der Elektronik erzeugte Wärme abzuleiten, eine Überhitzung zu verhindern und die Betriebssicherheit zu gewährleisten.
Traditionell wurden Drohnenschalen mit Methoden wie Spritzguss (hauptsächlich für Kunststoffe) oder CNC-Bearbeitung (für Metalle und einige Kunststoffe) hergestellt. Diese Methoden eignen sich zwar für die Massenproduktion einfacher Designs, stoßen aber oft an ihre Grenzen, wenn Spitzenleistungen angestrebt werden, insbesondere in Bezug auf Gewicht und Designkomplexität. Das Spritzgießen erfordert teure Werkzeuge, was die Anpassung an Kundenwünsche und die Produktion von Kleinserien kostspielig macht, und ist oft auf Materialien angewiesen, bei denen die Festigkeit auf Kosten des Gewichts geht. Die CNC-Bearbeitung ist zwar in der Lage, starke Metallteile herzustellen, kann aber sehr subtraktiv sein, was zu Materialabfall führt, und hat Probleme mit hochkomplexen Geometrien oder internen Merkmalen, die oft für eine maximale Gewichtsreduzierung und Komponentenintegration gewünscht werden.
Hier ist Additive Fertigung von Metall (AM)gemeinhin als Metall bekannt 3D-Druckentwickelt sich zu einer transformativen Kraft. Technologien wie Laser Powder Bed Fusion (LPBF) - die das selektive Laserschmelzen (SLM) und das direkte Metall-Lasersintern (DMLS) umfassen - ermöglichen den schichtweisen Aufbau von hochkomplexen Metallteilen direkt aus digitalen Entwürfen. Dieses Verfahren befreit die Konstrukteure von vielen traditionellen Fertigungsbeschränkungen und ebnet den Weg für radikal optimierte Drohnenhüllen.
Insbesondere bei Anwendungen, die eine hohe Festigkeit und ein geringes Gewicht erfordern, liegt der Schwerpunkt dieser Entwicklung zunehmend auf leichte Aluminiumlegierungen. Werkstoffe wie AlSi10Mg und der Hochleistungswerkstoff Scalmalloy® bieten ein außergewöhnliches Verhältnis von Festigkeit zu Gewicht, eine gute Korrosionsbeständigkeit und sind für die hohen Anforderungen moderner Drohnen gut geeignet. In Kombination mit der geometrischen Freiheit, die das Metall-AM bietet, ermöglichen diese Materialien die Herstellung von Drohnenhüllen, die deutlich leichter, stärker und funktionell integrierter sind als je zuvor.
Dieser Artikel taucht in die Welt der 3D-gedruckten Drohnenschalen ein und konzentriert sich dabei auf die Verwendung von leichten Aluminiumlegierungen wie AlSi10Mg und Scalmalloy®. Wir erkunden die verschiedenen Anwendungen, die von dieser Technologie profitieren, erläutern die überzeugenden Vorteile der Metall-AM gegenüber herkömmlichen Methoden, untersuchen die wichtigsten Materialeigenschaften und geben Einblicke in Designüberlegungen, Nachbearbeitung, Qualitätskontrolle und Lieferantenauswahl. Für Ingenieure, die die Grenzen des UAV-Designs ausloten, und für Beschaffungsmanager, die zuverlässige, leistungsstarke Anbieter von Drohnenkomponenten
ist das Verständnis der Möglichkeiten von 3D-gedrucktem Aluminium von größter Bedeutung. Unternehmen wie Met3dpmit ihrem umfassenden Fachwissen im Bereich der additiven Fertigungsanlagen für Metalle, fortschrittliche Systeme zur Pulverherstellung
und hochleistungsfähige Metallpulver stehen an vorderster Front und liefern die Werkzeuge und Materialien, die für die Realisierung der nächsten Generation von UAV-Leistungen erforderlich sind. Seien Sie dabei, wenn wir erkunden, wie diese Synergie aus fortschrittlichen Materialien und Fertigungstechnologien leichtere, stärkere und leistungsfähigere Drohnen für eine Vielzahl von Anwendungen ermöglicht.
Anwendungen: Wo werden die leichten Aluminium-Drohnenhüllen eingesetzt?
Die Vorteile leichter, hochfester und geometrisch komplexer Drohnenschalen, die mit 3D-gedruckten Aluminiumlegierungen hergestellt werden, sind nicht nur theoretisch, sondern werden in einer rasch wachsenden Zahl anspruchsvoller UAV-Anwendungen aktiv genutzt. Die Möglichkeit, Designs für spezifische Missionen zuzuschneiden, komplexe Funktionen zu integrieren und erhebliche Gewichtseinsparungen zu erzielen, macht diese Technologie besonders attraktiv, wenn Leistung, Langlebigkeit und individuelle Anpassung entscheidend sind. Beschaffungsmanager, die sich für Großhandel Drohne Teile
oder spezialisierte Komponenten finden, dass AM Lösungen bietet, die zuvor unerreichbar waren.
Im Folgenden finden Sie eine Aufschlüsselung der wichtigsten Sektoren und Anwendungen, in denen 3D-gedruckte Drohnenschalen aus Aluminium einen bedeutenden Einfluss haben:
1. Luft- und Raumfahrt & Verteidigung: In diesem Sektor, der oft der Schmelztiegel für Spitzentechnologie ist, werden in großem Umfang fortschrittliche UAVs eingesetzt, bei denen Leistung und Zuverlässigkeit nicht verhandelbar sind.
- Überwachung und Aufklärung (ISR): Drohnen, die für die Nachrichtengewinnung eingesetzt werden, benötigen eine lange Lebensdauer (geringes Gewicht), Tarnkappen (möglicherweise komplexe Formen zur Verringerung der Radarsignatur) und die Fähigkeit, anspruchsvolle Sensornutzlasten zu tragen. 3D-gedruckte Aluminiumschalen ermöglichen hochgradig optimierte aerodynamische Formen, integrierte Sensorhalterungen, die für spezifische Hardware entwickelt wurden, und robuste Strukturen, die auch anspruchsvollen Einsatztempi standhalten. Die hohe Festigkeit von Scalmalloy® ist besonders für kritische Strukturelemente von Vorteil.
- Taktische UAVs: Kleinere, schnell einsetzbare Drohnen, die von Bodentruppen eingesetzt werden, müssen robust, tragbar und flexibel einsetzbar sein. AM ermöglicht die Herstellung langlebiger, stoßfester Gehäuse mit integrierten Griffen, modularen Nutzlastschächten und optimierten Innenstrukturen, um die erforderliche Ausrüstung kompakt unterzubringen. Die Konsolidierung von Bauteilen reduziert potenzielle Fehlerquellen in rauen Umgebungen.
- Ziel-Drohnen: Zieldrohnen, die manchmal als Wegwerfartikel angesehen werden, müssen bestimmte Flugeigenschaften und Radarsignaturen genau nachbilden. AM ermöglicht die kosteneffiziente Herstellung komplexer aerodynamischer Formen in geringeren Stückzahlen, möglicherweise unter Verwendung von AlSi10Mg, um ein ausgewogenes Verhältnis zwischen Leistung und Kosten zu erreichen.
- Kundenspezifische Militärplattformen: Für spezielle Einsätze sind oft einzigartige Flugzeugkonstruktionen erforderlich. Metall-AM macht teure Werkzeuge für Sonderanfertigungen überflüssig und ermöglicht die schnelle Entwicklung und den Einsatz von UAVs, die auf spezifische Verteidigungsanforderungen zugeschnitten sind.
2. Gewerbliche und industrielle Tätigkeiten: Effizienz, Zuverlässigkeit und spezielle Funktionen treiben die Einführung von Drohnen in der kommerziellen Welt voran.
- Inspektion der Infrastruktur: Drohnen, die Stromleitungen, Windturbinen, Brücken, Pipelines und Eisenbahnlinien inspizieren, brauchen Stabilität, präzise Manövrierbarkeit (oft unter windigen Bedingungen) und robuste Halterungen für hochauflösende Kameras und Sensoren. Leichte Aluminiumschalen tragen zur Stabilität bei und ermöglichen längere Inspektionsrouten. In komplexe Geometrien können schwingungsdämpfende Merkmale integriert werden, um eine bessere Bildqualität zu erreichen.
- Präzisionslandwirtschaft: UAVs überwachen den Gesundheitszustand von Pflanzen, kartieren Felder und führen gezielte Sprüharbeiten durch. Die Gehäuse müssen gegen Umwelteinflüsse (Staub, Feuchtigkeit, landwirtschaftliche Chemikalien) resistent sein und verschiedene Sensoren (Multispektral- und Hyperspektralsensoren) sowie potenzielle Sprühgeräte aufnehmen können. 3D-gedrucktes Aluminium bietet Haltbarkeit und die Möglichkeit, individuelle Halterungen und Gehäuse zu erstellen.
- Logistik und Lieferung: Der aufstrebende Bereich der Drohnenzustellung erfordert aerodynamische Effizienz für Geschwindigkeit und Reichweite, strukturelle Integrität für den Transport von Paketen unterschiedlicher Größe und Gewichte sowie Haltbarkeit für häufige Starts und Landungen. Topologieoptimierte Aluminiumschalen bieten das erforderliche Verhältnis von Festigkeit zu Gewicht und maximieren die Nutzlastkapazität und die Batterielebensdauer.
Herstellung kundenspezifischer Drohnen
ist hier unerlässlich, da die Lieferplattformen oft sehr spezialisiert sind. - Kinematographie und Luftbildaufnahmen: Professionelle Kameradrohnen erfordern extreme Stabilität, Vibrationsdämpfung und die Fähigkeit, schwere, hochwertige Kameranutzlasten zu tragen. Leichte, steife Aluminiumrahmen, die möglicherweise komplexe, mittels AM entwickelte Gitterstrukturen enthalten, minimieren Vibrationen und ermöglichen flüssigere Aufnahmen. Kundenspezifische Halterungen können direkt in das Gehäusedesign integriert werden.
- Kartierung und Vermessung: Ähnlich wie bei Inspektionen benötigen diese Drohnen stabile Plattformen für die genaue Datenerfassung mit LiDAR- oder Photogrammetriesensoren. Die Gewichtsreduzierung verlängert die Flugzeit und ermöglicht die Abdeckung größerer Gebiete pro Einsatz.
3. Notdienste und öffentliche Sicherheit: Drohnen werden zu wichtigen Hilfsmitteln für Ersthelfer.
- Suche und Rettung (SAR): SAR-Drohnen werden in schwierigem Gelände und unter schwierigen Wetterbedingungen eingesetzt. Die Schalen müssen robust und wetterfest sein und Wärmebildkameras, Scheinwerfer und möglicherweise Kommunikationsrelais oder kleine medizinische Geräte tragen können. 3D-gedrucktes Aluminium sorgt für die nötige Widerstandsfähigkeit und ermöglicht die Integration spezieller Halterungen für die Ausrüstung.
- Katastrophenhilfe und Überwachung: Nach Naturkatastrophen bieten Drohnen ein schnelles Situationsbewusstsein. Robuste, leicht einsetzbare Drohnen mit anpassungsfähiger Nutzlast sind unerlässlich. AM erleichtert die schnelle Herstellung von Ersatzteilen oder maßgeschneiderten Konfigurationen nach Bedarf.
Zusammenfassende Tabelle: Anwendungen und Vorteile von 3D-gedruckten Aluminiumschalen
Anwendungsbereich | Spezifische Beispiele für Anwendungsfälle | Wichtige Shell-Anforderungen | Wie 3D-gedrucktes Aluminium (AlSi10Mg/Scalmalloy®) die Anforderungen erfüllt |
---|---|---|---|
Luft- und Raumfahrt & Verteidigung | ISR, taktische UAVs, Zieldrohnen | Geringes Gewicht, hohe Festigkeit, Langlebigkeit, Tarnfunktionen, Sensorintegration, individuelle Anpassung | Optimiertes Verhältnis von Festigkeit zu Gewicht, komplexe Geometrien für Aerodynamik/Tarnkappe, integrierte Halterungen, Rapid Prototyping/Anpassung |
Kommerziell/Industriell | Infrastruktur-Inspektion, Landwirtschaft, Lieferung, Kino | Stabilität, Nutzlastkapazität, Langlebigkeit, Umweltbeständigkeit, aerodynamische Effizienz | Gewichtsreduzierung für Langlebigkeit/Nutzlast, kundenspezifische/integrierte Halterungen, robuste Konstruktion, optimierte Formen für Effizienz |
Notfalldienste | Suche und Rettung, Katastrophenhilfe | Robustheit, Witterungsbeständigkeit, Nutzlastflexibilität, schnelle Einsetzbarkeit | Langlebige Materialien, Designfreiheit für integrierte Funktionen/Dichtungen, Teilekonsolidierung für Zuverlässigkeit, Produktion auf Abruf |
In Blätter exportieren
Die Vielfalt dieser Anwendungen unterstreicht die Vielseitigkeit und das Hochleistungspotenzial von Drohnenschalen, die mit additiver Fertigung und modernen Aluminiumlegierungen hergestellt werden. Als Ingenieure und Lieferanten von Drohnenteilen
Durch weitere Innovationen wird 3D-gedrucktes Aluminium zunehmend zu einer Standardlösung, um die Grenzen der UAV-Fähigkeiten in allen Sektoren zu erweitern.
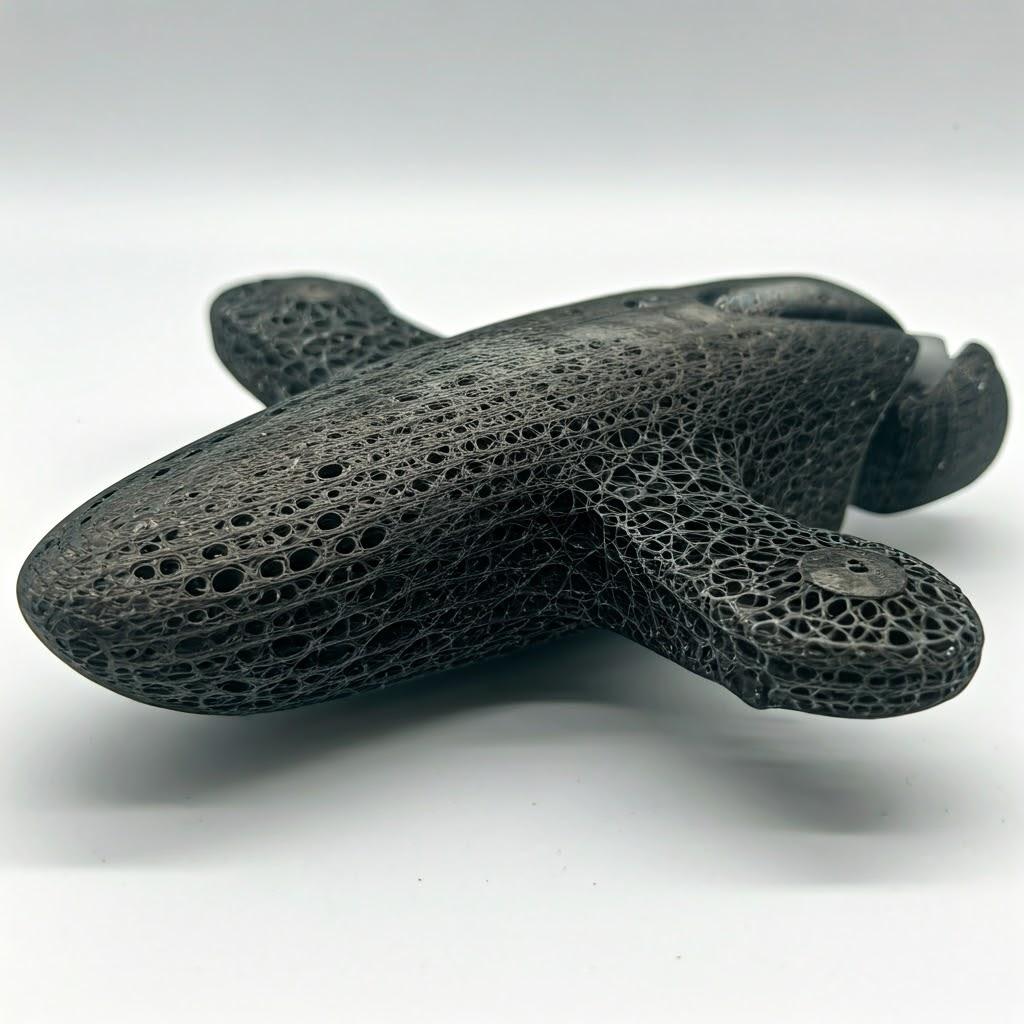
Warum 3D-Metalldruck für Drohnenschalen? Designfreiheit und Leistung freisetzen
Die Entscheidung für den Einsatz der additiven Fertigung von Metallen, insbesondere der Laser Powder Bed Fusion (LPBF), zur Herstellung von Drohnengehäusen beruht auf einer Reihe von überzeugenden Vorteilen, die die Grenzen herkömmlicher Fertigungsmethoden direkt ansprechen und perfekt mit den Zielen der modernen Drohnenentwicklung übereinstimmen: geringeres Gewicht, höhere Leistung und größere Designflexibilität. Für Ingenieure, die nach Innovation streben, und für Beschaffungsmanager, die nach innovativen, zuverlässigen Komponenten suchen, bietet Metal AM einen Paradigmenwechsel. Sehen wir uns die wichtigsten Gründe an, warum diese Technologie für Hochleistungsdrohnenstrukturen unverzichtbar wird.
1. Unerreichte Gestaltungsfreiheit und geometrische Komplexität: Dies ist wohl der transformativste Vorteil von AM. Im Gegensatz zu subtraktiven Verfahren (CNC-Bearbeitung), bei denen das Material abgetragen wird, oder zu formgebenden Verfahren (Gießen, Formen), die auf vordefinierte Formen oder Gesenke angewiesen sind, baut AM Teile Schicht für Schicht auf. Dieser additive Ansatz erschließt das Potenzial für:
- Hochkomplexe Geometrien: Die Konstrukteure können komplizierte Formen, interne Kanäle (für Kühlung oder Verkabelung), konforme Strukturen und organische Formen schaffen, die auf herkömmliche Weise nur schwer oder gar nicht zu realisieren sind. Dies ermöglicht eine extreme aerodynamische Optimierung, die auf bestimmte Flugbereiche zugeschnitten ist.
- Topologie-Optimierung: Ausgefeilte Softwarealgorithmen können Lastpfade und Spannungen innerhalb einer Drohnenschalenkonstruktion analysieren und Material aus unkritischen Bereichen entfernen, während hochbelastete Regionen verstärkt werden. Das Ergebnis sind leichte, hocheffiziente Strukturen, bei denen das Material nur dort eingesetzt wird, wo es strukturell notwendig ist.
Topologieoptimierung für Drohnenrahmen
ist eine wichtige Voraussetzung für eine drastische Gewichtsreduzierung. - Gitterförmige Strukturen: AM ermöglicht die Integration interner Gitterstrukturen - komplexe, sich wiederholende Netze aus Streben oder Zellen. Diese Strukturen können das Gewicht erheblich reduzieren und gleichzeitig eine hohe Steifigkeit und Festigkeit beibehalten. Außerdem können sie so konstruiert werden, dass sie bestimmte Schwingungsdämpfungs- oder Energieabsorptionseigenschaften aufweisen, die für den Schutz empfindlicher Nutzlasten entscheidend sind.
- Teil Konsolidierung: Mehrere Komponenten, die traditionell separat hergestellt und dann zusammengebaut werden (z. B. Halterungen, Befestigungen, Abschnitte des Gehäuses), können oft neu entworfen und als ein einziges, monolithisches Teil gedruckt werden. Dies reduziert die Anzahl der Teile, die Montagezeit, das Gewicht und potenzielle Fehlerquellen (Befestigungselemente, Verbindungen).
- Integrierte Funktionalitäten: Merkmale wie Befestigungspunkte für Sensoren, Antennen, Kabelkanäle, Batterieanschlüsse und sogar Flüssigkeitskanäle können direkt in das Gehäusedesign integriert werden, was die Montage vereinfacht und die Systemintegration insgesamt verbessert.
2. Erhebliches Leichtbaupotenzial: Das Gewicht ist ein Hauptfeind der Drohnenleistung. Jedes Gramm, das eingespart wird, führt direkt zu greifbaren Vorteilen: längere Flugzeiten, höhere Nutzlastkapazität, verbesserte Agilität und Manövrierbarkeit sowie geringerer Energieverbrauch. Metall-AM erleichtert die Gewichtsreduzierung durch mehrere Mechanismen, die durch Designfreiheit ermöglicht werden:
- Topologie-Optimierung: Wie bereits erwähnt, führt das Entfernen von strukturell unnötigem Material zu leichteren Teilen. Im Vergleich zu traditionell konstruierten Metallteilen sind oft Einsparungen von 20-50% möglich.
- Gitterförmige Strukturen: Das Ersetzen von Volumenkörpern durch optimierte Gitter ermöglicht eine drastische Gewichtsreduzierung bei gleichzeitiger Wahrung der strukturellen Integrität.
- Materialien mit hoher Festigkeit im Verhältnis zum Gewicht: AM-Verfahren eignen sich hervorragend für Materialien wie AlSi10Mg und insbesondere Scalmalloy®, die im Vergleich zu vielen konventionell verwendeten Materialien (einschließlich einiger Kunststoffe und Standardaluminiumsorten) eine höhere Festigkeit im Verhältnis zu ihrer Dichte aufweisen. Dies ermöglicht dünnere Wände und schlankere Strukturelemente, ohne die Festigkeit zu beeinträchtigen.
- Teil Konsolidierung: Durch den Wegfall von Verbindungselementen (Schrauben, Nieten, Bolzen) und Montageschnittstellen wird das Gesamtgewicht weiter reduziert.
3. Verbesserte Materialleistung und -auswahl: Bei der Metall-AM geht es nicht nur um die Form, sondern auch um den Einsatz fortschrittlicher Materialien, die speziell für das Verfahren geeignet sind oder sogar dafür entwickelt wurden.
- Optimierte Mikrostrukturen: Die schnelle Erwärmung und Abkühlung, die LPBF-Prozessen eigen ist, kann zu feinkörnigen Mikrostrukturen in Legierungen wie AlSi10Mg und Scalmalloy® führen, was oft zu mechanischen Eigenschaften (wie Festigkeit und Härte) führt, die denen von gegossenen oder gekneteten Gegenstücken entsprechen oder diese übertreffen, insbesondere nach entsprechenden Wärmebehandlungen nach dem Prozess.
- Zugang zu Hochleistungslegierungen: AM ermöglicht den praktischen Einsatz von Legierungen wie Scalmalloy®, die speziell für die additive Fertigung entwickelt wurden und Leistungsmerkmale bieten, die bei komplexen Aluminiumkomponenten bisher nur schwer zu erreichen waren.
- Konsistente Materialeigenschaften: Eine fortschrittliche Prozesssteuerung und hochwertige Pulver, wie sie mit Met3dp's
fortschrittliche Systeme zur Pulverherstellung
gewährleisten gleichbleibende Materialeigenschaften über das gesamte gedruckte Teil, was für eine zuverlässige Leistung entscheidend ist.
4. Rapid Prototyping, Iteration und Anpassung: Das Innovationstempo in der Drohnenindustrie ist rasant. Metall-AM beschleunigt den Entwicklungszyklus erheblich.
- Werkzeugfreie Produktion: AM erfordert keine Gussformen, Matrizen oder spezielle Werkzeuge. Die Teile können direkt aus einer CAD-Datei gedruckt werden. Dadurch werden die Vorlaufzeit und die Kosten für die Herstellung erster Prototypen oder für Designänderungen drastisch reduziert.
- Schnelle Design-Iteration: Ingenieure können eine Drohnenhülle entwerfen, einen funktionalen Prototyp aus Aluminium drucken, ihn testen, verbesserungswürdige Bereiche identifizieren, den digitalen Entwurf ändern und eine überarbeitete Version innerhalb von Tagen oder Wochen statt Monaten drucken. Dieser iterative Kreislauf ermöglicht eine schnelle Optimierung und Verfeinerung.
- Kosteneffiziente Anpassung: Die Herstellung einzigartiger oder in Kleinserien hergestellter Drohnenschalen ist mit AM wirtschaftlich machbar. Ganz gleich, ob es sich um eine spezifische Nutzlast für eine Mission, ein einzigartiges Sensorsystem oder eine maßgeschneiderte Forschungsplattform handelt, AM ermöglicht
Partner für die Herstellung kundenspezifischer Drohnen
um maßgeschneiderte Lösungen zu liefern, ohne die unerschwinglichen Kosten der traditionellen Werkzeugherstellung für kleine Serien.
5. Vorteile der Lieferkette und bedarfsorientierte Fertigung: AM bietet ein flexibleres und potenziell widerstandsfähigeres Lieferkettenmodell.
- Reduzierte Vorlaufzeiten: Bei komplexen Teilen kann die Gesamtdurchlaufzeit von der Fertigstellung des Designs bis zum fertigen Teil mit AM oft kürzer sein als mit der Koordination mehrerer traditioneller Fertigungsschritte und der Montage.
- Produktion auf Abruf: Die Teile können je nach Bedarf gedruckt werden, wodurch sich der Bedarf an großen Lagerbeständen von Fertigwaren oder Komponenten verringert. Dies ist besonders für spezialisierte, hochwertige Drohnenschalen von Vorteil.
- Verteilte Fertigung: Die Produktion kann potenziell dezentralisiert werden, wodurch die Herstellung näher an den Ort des Bedarfs rückt und die Transportkosten und die logistische Komplexität reduziert werden.
Vergleich: Metall-AM vs. traditionelle Methoden für Drohnenhüllen
Merkmal | Metall AM (LPBF - Al-Legierungen) | CNC-Bearbeitung (Aluminium) | Spritzgießen (Kunststoffe) | Verbundwerkstoff-Layup (z. B. Kohlefaser) |
---|---|---|---|---|
Geometrische Komplexität | Sehr hoch (interne Merkmale, Gitter) | Mäßig (begrenzt durch den Zugang zu Werkzeugen) | Hoch (erfordert jedoch komplexe Formen) | Mäßig-Hoch (Einschränkungen durch die Form) |
Potenzial zur Gewichtsreduzierung | Sehr hoch (Topologie opt., Gitternetze) | Mäßig (subtraktive Grenzen) | Mäßig (Materialdichte begrenzt) | Sehr hoch (ausgezeichnetes Verhältnis von Festigkeit zu Gewicht) |
Materialstärke | Hoch (AlSi10Mg) bis sehr hoch (Scalmalloy®) | Hoch (abhängig von der Legierung) | Gering bis mäßig | Sehr hoch |
Vorlaufzeit für Prototyping | Fasten (Tage/Wochen) | Mäßig (Wochen) | Langsam (Monate - wegen der Werkzeugherstellung) | Mäßig langsam (manueller Prozess/Tooling) |
Produktionsvorlaufzeit | Mäßig (abhängig von der Skalierbarkeit) | Schnell (für hohes Volumen) | Sehr schnell (für hohes Volumen) | Langsam (arbeitsintensiv) |
Kosten der Anpassung | Niedrig (keine Werkzeuge) | Hoch (Programmierung/Einrichtung) | Sehr hoch (Werkzeugänderung) | Hoch (Änderung der Form/Werkzeuge) |
Werkzeugkosten | Keiner | Niedrig-Mäßig (Vorrichtungen) | Sehr hoch | Mäßig-hoch |
Teil Konsolidierung | Ausgezeichnet | Begrenzt | Begrenzt | Begrenzt |
In Blätter exportieren
Während Verbundwerkstoffe wie Karbonfasern ein hervorragendes Leichtgewicht darstellen, bietet Metall-AM Vorteile bei der Herstellung extrem komplexer monolithischer Strukturen, isotrope Materialeigenschaften (vorhersagbare Festigkeit in allen Richtungen) und oft eine einfachere Integration von Gewindeelementen oder komplexen Montageschnittstellen im Vergleich zu Verbundwerkstoffen. Darüber hinaus bieten das Know-how und die fortschrittlichen Systeme von Unternehmen, die sich auf 3D-Druck von Metall stellen sicher, dass das Potenzial dieser Aluminiumlegierungen voll ausgeschöpft wird und Teile entstehen, die den strengen Anforderungen der Luft- und Raumfahrt und der Industrie entsprechen. Die Kombination aus Designfreiheit, Leichtbau, Materialleistung und schneller Iteration macht Metall-AM zu einem unverzichtbaren Werkzeug für die Entwicklung der nächsten Generation von Hochleistungsdrohnenschalen.
Werkstoff-Fokus: AlSi10Mg und Scalmalloy® für leistungsstarke Drohnenstrukturen
Die Wahl des richtigen Materials ist von grundlegender Bedeutung für die Konstruktion von Hochleistungskomponenten, und Drohnenschalen bilden da keine Ausnahme. Während in der additiven Fertigung verschiedene Materialien verwendet werden können, haben sich Aluminiumlegierungen aufgrund ihrer überzeugenden Kombination aus geringer Dichte, guten mechanischen Eigenschaften, Korrosionsbeständigkeit und Verarbeitbarkeit mit Laser Powder Bed Fusion (LPBF)-Verfahren als beliebte Wahl für Drohnenstrukturen herausgestellt. Innerhalb der Familie des druckbaren Aluminiums stechen zwei Legierungen für Drohnenanwendungen hervor: das Arbeitspferd AlSi10Mg und die leistungsstarke Scalmalloy®. Für Ingenieure und Beschaffungsmanager, die das Design von UAVs optimieren wollen, ist es entscheidend, ihre Eigenschaften, Vorteile und idealen Anwendungsfälle zu kennen. Darüber hinaus ist die Beschaffung dieser Materialien von seriösen Lieferanten von Metallpulver
die Qualität und Konsistenz garantieren, wie Met3dp mit seinem hochmodernen fortschrittliche Systeme zur Pulverherstellung
ist für die Erzielung zuverlässiger und wiederholbarer Ergebnisse unerlässlich.
Einführung in Aluminiumlegierungen in der Metall-AM:
Aluminiumlegierungen sind wesentlich leichter als Stähle oder Titanlegierungen, was sie für Anwendungen in der Luft- und Raumfahrt, bei denen Gewichtseinsparungen im Vordergrund stehen, von Natur aus attraktiv macht. Im Zusammenhang mit LPBF stellen Aluminiumlegierungen aufgrund ihres hohen Reflexionsvermögens und ihrer Wärmeleitfähigkeit einige Herausforderungen dar, aber dank der Fortschritte in der Maschinentechnologie und der Entwicklung von Prozessparametern ist das Drucken hochwertiger Aluminiumteile inzwischen Routine. Die verwendeten Legierungen enthalten in der Regel Elemente wie Silizium (zur Verbesserung der Fließfähigkeit und zur Verringerung der Rissneigung), Magnesium (zur Verfestigung durch Wärmebehandlung) und im Falle von Scalmalloy® Scandium und Zirkonium (für außergewöhnliche Festigkeit).
AlSi10Mg: Das vielseitige Arbeitspferd
- Zusammensetzung und Merkmale: AlSi10Mg ist im Wesentlichen eine Aluminiumgusslegierung für die additive Fertigung. Sie enthält etwa 9-11% Silizium und 0,2-0,45% Magnesium. Der Siliziumgehalt sorgt für eine gute "Schweißbarkeit" während des schichtweisen Schmelzprozesses und minimiert die Rissbildung, während das Magnesium eine Ausscheidungshärtung der Legierung durch Wärmebehandlung ermöglicht.
- Vorteile für Drone Shells:
- Breite Verfügbarkeit und Reifegrad: Es ist eine der gängigsten und am besten charakterisierten Aluminiumlegierungen, die in der Metall-AM verwendet werden, was bedeutet, dass die Prozessparameter gut bekannt sind und eine Fülle von Daten verfügbar ist.
- Gute Verarbeitbarkeit: Er druckt im Allgemeinen zuverlässig mit guter Dichte und Oberflächengüte, die mit verschiedenen LPBF-Plattformen erreicht werden können.
- Ausgezeichnete thermische Eigenschaften: Es weist eine gute Wärmeleitfähigkeit auf, was für die Wärmeableitung von Motoren oder der in die Drohnenhülle integrierten Elektronik von Vorteil sein kann.
- Gutes Verhältnis von Stärke zu Gewicht: Es ist zwar nicht so stark wie Scalmalloy®, bietet aber eine deutliche Verbesserung gegenüber den meisten Kunststoffen und ein ausgewogenes Verhältnis von Festigkeit, Steifigkeit und geringem Gewicht, das sich für viele Strukturkomponenten eignet.
- Kosten-Nutzen-Verhältnis: Im Allgemeinen ist AlSi10Mg-Pulver preiswerter als Hochleistungslegierungen wie Scalmalloy® und eignet sich daher für Prototypen, weniger anspruchsvolle Strukturteile und Anwendungen, bei denen das Budget eine wichtige Rolle spielt.
- Typische mechanische Eigenschaften: Die Eigenschaften können je nach den Druckparametern und der Wärmebehandlung variieren. Eine typische T6-Wärmebehandlung (Lösungsglühen und künstliche Alterung) verbessert die Festigkeit und Härte erheblich. | Eigenschaft | Zustand | Typischer Wertebereich | Einheit | Anmerkungen | | :------- | :----- | :--------- | :---- | :-------------- | | Dichte | Druckzustand | ~ 2,67 | g/cm³ | Deutlich leichter als Stahl oder Titan | | Höchstzugkraft | Druckzustand | 330 - 430 | MPa | | | Höchstzugkraft | Wärmebehandelt (T6) | 440 - 480 | MPa | Vergleichbar mit mittelfesten Al-Knetlegierungen | | | Streckgrenze (0.2%)| Druckfrisch | 180 - 250 | MPa | | Streckgrenze (0.2%)| Wärmebehandelt (T6) | 280 - 330 | MPa | Deutliche Erhöhung nach der Wärmebehandlung | | Bruchdehnung | Druckfrisch | 6 - 11 | % | Angemessene Duktilität | | Bruchdehnung | Wärmebehandelt (T6) | 8 - 15 | % | Verbessert sich oft leicht mit T6 | | Härte | Wärmebehandelt (T6) | 110 - 130 | HV | Gute Härte für Verschleißfestigkeit |
- Ideale Anwendungen: Allzweck-Drohnenschalen, Prototypen, die Metalleigenschaften erfordern, strukturelle Halterungen, in Rahmen integrierte Kühlkörper, Komponenten, bei denen die Kosten im Vordergrund stehen, aber eine über Kunststoff hinausgehende Festigkeit erforderlich ist.
Scalmalloy®: Der Hochleistungschampion
- Zusammensetzung und Merkmale: Die von APWORKS (einer Airbus-Tochtergesellschaft) entwickelte Scalmalloy® ist eine Aluminium-Magnesium-Scandium-Zirkonium-Legierung (Al-Mg-Sc-Zr), die speziell für die harten Anforderungen der additiven Fertigung entwickelt wurde. Durch die geringen Zusätze von Scandium und Zirkonium entstehen während des Drucks und der Wärmebehandlung extrem feine Ausscheidungen, die zu außergewöhnlichen mechanischen Eigenschaften führen.
- Vorteile für Drone Shells:
- Außergewöhnliches Verhältnis von Stärke zu Gewicht: Dies ist die entscheidende Eigenschaft von Scalmalloy®. Seine spezifische Festigkeit (Festigkeit geteilt durch Dichte) erreicht oder übertrifft die einiger hochfester Stähle und Titanlegierungen, was wirklich minimale Konstruktionen ermöglicht.
- Hohe Duktilität: Im Gegensatz zu vielen anderen hochfesten Aluminiumlegierungen weist Scalmalloy® eine gute Duktilität auf, was es widerstandsfähiger gegen Brüche unter Belastung macht.
- Ausgezeichnete Ermüdungsfestigkeit: Entscheidend für Komponenten, die zyklischen Belastungen und Vibrationen ausgesetzt sind, wie z. B. Flugwerke von Drohnen und Motorhalterungen.
- Gute Schweißbarkeit und Korrosionsbeständigkeit: Es zeichnet sich durch gute Schweißbarkeit (nützlich für eventuelle Nachbearbeitungen oder Reparaturen) und Korrosionsbeständigkeit aus, die für Luft- und Raumfahrt und Außenanwendungen geeignet ist.
- Ermöglicht extremes Lightweighting: Dank seiner hohen Festigkeit können die Konstrukteure die Topologieoptimierung und dünnwandige Designs weiter vorantreiben, als dies mit AlSi10Mg möglich ist, was zu einer maximalen Gewichtsreduzierung führt.
- Typische mechanische Eigenschaften: Scalmalloy® erfordert in der Regel eine spezielle Wärmebehandlung, um seine optimalen Eigenschaften zu erreichen. | Eigenschaft | Zustand | Typischer Wertebereich | Einheit | Anmerkungen | | :------- | :------ | :------ | :-- | :--------------------- | | Dichte | Druckfrisch | ~ 2,66 | g/cm³ | Ähnliche Dichte wie AlSi10Mg | | Höchstzugkraft | Wärmebehandelt | 520 - 540 | MPa | Deutlich höher als AlSi10Mg T6, annähernd auf dem Niveau von Ti-6Al-4V | | Streckgrenze (0.2%)| Wärmebehandelt | 480 - 500 | MPa | Außergewöhnlich hohe Streckgrenze für eine Aluminiumlegierung | | Bruchdehnung | Wärmebehandelt | 11 - 15 | % | Ausgezeichnete Duktilität für sein Festigkeitsniveau | | Ermüdungsfestigkeit | Wärmebehandelt | Hoch | MPa | Überragende Ermüdungseigenschaften, die für dynamische Belastungen entscheidend sind |
- Ideale Anwendungen: Primäre Strukturbauteile für Hochleistungsdrohnen, einsatzkritische Teile der Flugzeugzelle, Anwendungen, die eine größtmögliche Gewichtsreduzierung erfordern, Bauteile, die hohen zyklischen Belastungen oder Vibrationen ausgesetzt sind, wobei schwerere Materialien wie Titan oder Stahl nach Möglichkeit ersetzt werden.
Die Wahl zwischen AlSi10Mg und Scalmalloy®:
Die Auswahl hängt stark von den spezifischen Anforderungen an die Drohnenhülle und den Projektbedingungen ab:
- Leistungsbedürfnisse: If maximizing strength-to-weight, fatigue resistance, and overall performance is the top priority, Scalmalloy® is the superior choice, despite its higher cost.
- Kostenzwänge: If the budget is tighter or the structural demands are less extreme, AlSi10Mg provides a very capable and more economical solution.
- Prototyping vs. Production: AlSi10Mg is often preferred for early-stage functional prototypes due to its lower cost and wider availability, while Scalmalloy® might be chosen for final production parts of high-performance drones.
- Operating Environment: Both offer good corrosion resistance, but specific environmental factors might slightly favor one over the other or necessitate specific surface treatments (discussed later).
Ultimately, both AlSi10Mg and Scalmalloy® represent excellent choices for 3D printed aluminum drone shells, enabled by advanced LPBF technology and high-quality metal powders. Partnering with a knowledgeable AM service provider like Met3dp, which combines expertise in both printing processes and materials science – backed by their investment in producing premium powders through methods like gas atomization – ensures that the chosen material delivers its full potential, resulting in lighter, stronger, and more capable UAVs.
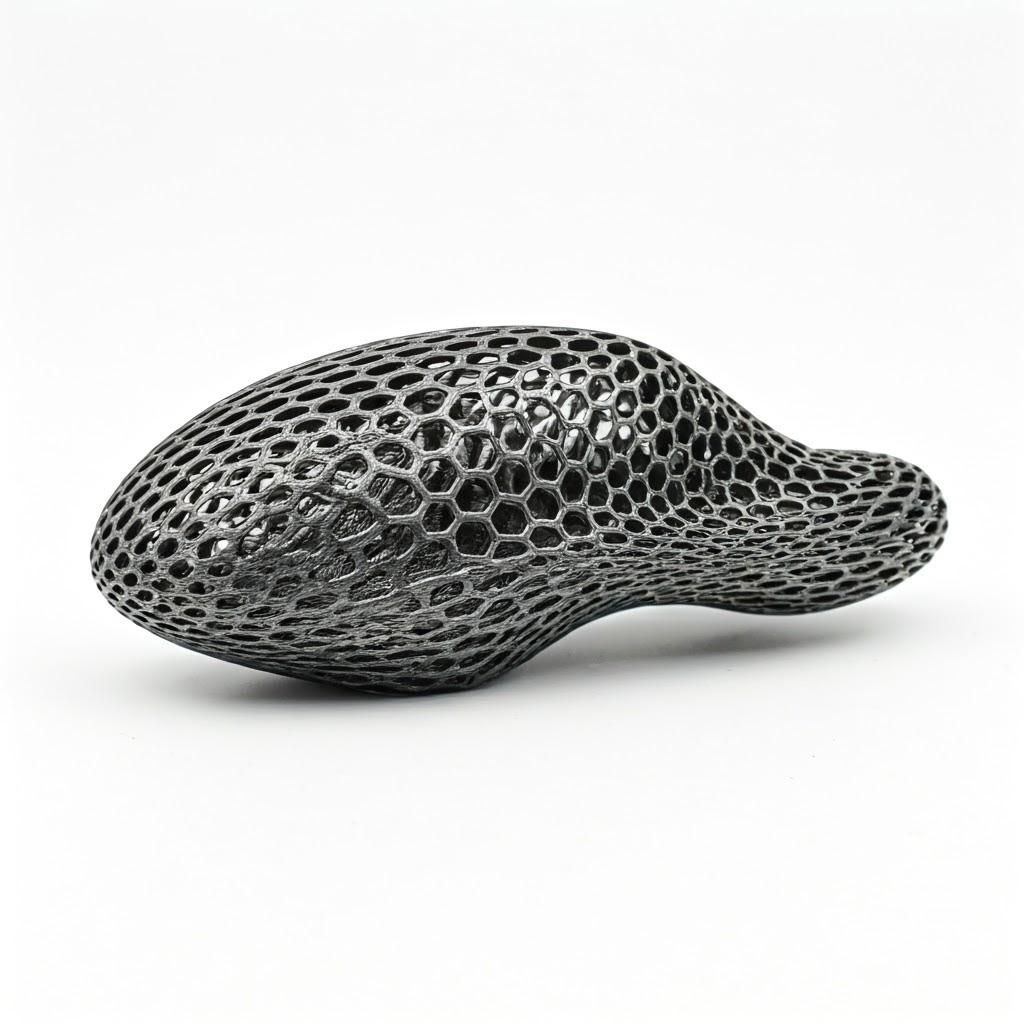
Design for Additive Manufacturing (DfAM): Optimizing Drone Shells for 3D Printing
Successfully leveraging metal 3D printing for drone shells isn’t merely about taking an existing design and hitting “print.” To truly unlock the transformative potential of additive manufacturing – achieving maximum lightweighting, integrating complex functionalities, and ensuring cost-effective, high-quality production – requires embracing a methodology known as Design für additive Fertigung (DfAM). DfAM is a fundamental shift in thinking, moving away from the constraints of traditional manufacturing and designing parts specifically to take advantage of the layer-by-layer build process offered by technologies like Laser Powder Bed Fusion (LPBF). For engineers designing the next generation of UAVs, mastering DfAM principles is paramount; for procurement managers, understanding these principles helps in evaluating the capabilities of potential DfAM services
and AM partners.
Why is DfAM so critical for aluminum drone shells? Because simply replicating a design intended for CNC machining or injection molding using AM often results in suboptimal outcomes: parts that are unnecessarily heavy, require excessive support structures (increasing cost and post-processing time), suffer from higher residual stress, or fail to capitalize on the unique geometric possibilities of AM. Effective DfAM, conversely, leads to superior components optimized for both performance and manufacturability within the AM process.
Here are key DfAM principles essential for optimizing aluminum drone shells for LPBF:
1. Strategic Part Orientation and Support Structure Minimization: How a part is oriented on the build plate profoundly impacts several factors:
- Support Needs: LPBF requires support structures for overhanging features typically below a certain angle (often around 45 degrees for aluminum, though material and parameter dependent). Strategic orientation aims to minimize the volume and complexity of these supports.
- Oberfläche: Different surfaces (upward-facing, downward-facing, vertical walls) exhibit varying roughness. Orientation can prioritize finish quality on critical surfaces. Downward-facing surfaces tend to be rougher due to contact with supports.
- Eigenspannung: Orientation influences heat distribution and cooling rates, affecting internal stress buildup.
- Print Time & Cost: Taller prints generally take longer. Minimizing height (Z-axis) can reduce build time.
- Feature Accuracy: Fine features might resolve better in certain orientations.
- DfAM Goal: Orient the drone shell to minimize steep overhangs. Utilize self-supporting angles where possible. Design features like chamfers or fillets instead of sharp horizontal overhangs. When supports are unavoidable, design them for easy removal without damaging the part surface, potentially incorporating specific break-away points or using lattice supports that consume less material and are easier to detach.
2. Harnessing Topology Optimization and Lattice Structures: These powerful computational tools are intrinsically linked to DfAM:
- Topologie-Optimierung: Software analyzes the load conditions (e.g., motor thrust, landing impact, payload weight) on the drone shell and intelligently removes material from areas experiencing low stress, leaving behind an optimized, often organic-looking load-bearing structure. This is a primary driver for achieving dramatic weight reductions (e.g., transforming a solid mounting bracket into a skeletal frame) while maintaining or even increasing stiffness in critical areas.
- Gitterförmige Strukturen: Instead of solid infill, designers can incorporate internal lattices (honeycomb, gyroid, stochastic foams, etc.). These offer remarkable weight savings with tailored structural properties (stiffness, energy absorption). For drone shells, lattices can fill non-critical volumes, provide vibration damping, or create specific crush zones for impact protection. Selecting the appropriate lattice type, cell size, and strut thickness is key.
3. Embracing Part Consolidation: AM’s ability to create complex single pieces allows designers to rethink assemblies:
- Konzept: Combine multiple, traditionally separate components (e.g., shell sections, motor mounts, battery trays, wiring clips, antenna housings) into a single, integrated printed part.
- Vorteile:
- Reduced Part Count: Simplifies inventory and supply chain management.
- Eliminated Assembly Steps: Saves significant time and labor costs.
- Weight Reduction: Removes the need for fasteners (screws, rivets, bolts, adhesives) and overlapping joint flanges.
- Increased Reliability: Fewer joints mean fewer potential failure points or points of ingress for dust/moisture.
- Improved Performance: Monolithic structures can offer greater stiffness and better load distribution.
- Beispiel: A drone arm could be redesigned from a tube, separate motor mount, and wiring clips into a single printed component featuring an optimized internal structure, integrated motor mounting points, and internal channels for wiring.
4. Respecting Wall Thickness and Feature Size Limitations: LPBF processes have physical limitations:
- Mindestwanddicke: There’s a limit to how thin a structurally sound wall can be printed reliably (often around 0.4-0.8 mm for aluminum, depending on the machine and parameters). Designers must ensure structural walls meet or exceed this minimum.
- Minimale Featuregröße: Very small pins, holes, or intricate details also have resolution limits. Consult the AM provider’s specific capabilities.
- Unsupported Spans: Large horizontal spans or flat roofs cannot be printed without supports. Designing with self-supporting angles or incorporating sacrificial ribs might be necessary if supports are undesirable in certain areas.
- Bildseitenverhältnis: Very tall, thin features can be prone to distortion or vibration during printing.
5. Intelligent Hole Design: Holes are common features, but require thought in AM:
- Vertical vs. Horizontal: Vertical holes (parallel to the build direction) generally print with better accuracy and finish.
- Self-Supporting Horizontal Holes: Small horizontal holes can often be printed without supports, but larger ones require them. Designing horizontal holes with a “teardrop” or diamond shape at the top allows them to be self-supporting up to a certain diameter.
- Die Genauigkeit: For high-precision holes (e.g., for bearings or press-fits), it’s often best practice to design them slightly undersized and then ream or machine them to the final tolerance during post-processing. Threading is also typically done post-print via tapping or using thread inserts.
6. Designing for Thermal Management (During Print & Operation):
- During Printing: Avoid large, solid sections connected by very thin features, as this can create uneven heat distribution and increase residual stress. Gradual transitions in thickness are preferred.
- During Operation: DfAM allows for the seamless integration of thermal management features directly into the drone shell, such as heat sink fins near motors or electronics, or internal channels designed for passive or active air cooling.
7. Planning for Post-Processing: Effective DfAM anticipates downstream steps:
- Unterstützung bei der Entfernung Zugang: Ensure supports are placed in areas accessible for removal tools without damaging critical features.
- Zulagen für die Bearbeitung: If surfaces require high precision or specific finishes achievable only through machining, design these features with extra “stock” material (e.g., 0.5-1.0 mm) to be removed during CNC machining.
- Surface Treatment Considerations: Design parts to avoid features that might trap blasting media or prevent uniform coating application.
Collaboration is Key: Successfully implementing DfAM often involves close collaboration between the product designer and the additive manufacturing service provider. Experienced AM specialists, like the team at Met3dp, can provide invaluable feedback on design feasibility, suggest optimizations for printability and cost-effectiveness, and help navigate the nuances of material selection and process parameters. Leveraging their expertise early in the design cycle ensures that the final drone shell fully benefits from the capabilities of additive manufacturing. Exploring the comprehensive Met3dp range of products and services can provide insights into how such partnerships can streamline the journey from concept to high-performance printed part. Engaging with AM experts transforms DfAM from a theoretical exercise into a practical pathway for innovation.
Achievable Precision: Tolerance, Surface Finish, and Dimensional Accuracy in Aluminum Drone Shells
When specifying components for aerospace and high-performance applications like drone shells, precision is paramount. Engineers and procurement managers need a clear understanding of the dimensional capabilities and limitations of metal additive manufacturing using aluminum alloys like AlSi10Mg and Scalmalloy®. While metal AM offers unprecedented design freedom, it’s crucial to differentiate between as-printed capabilities and the precision achievable after secondary operations. Let’s clarify the concepts of tolerance, surface finish, and dimensional accuracy in the context of Laser Powder Bed Fusion (LPBF) for aluminum drone parts.
Defining the Terms:
- Maßgenauigkeit: This refers to the overall conformity of the printed part to the nominal dimensions specified in the CAD model. It describes how closely the final part matches the intended size and shape across its entire geometry. It’s often expressed as a general deviation, like ±0.1% of the dimension, or a fixed value like ±0.1 mm over a certain length.
- Verträglichkeit: This defines the permissible range of variation for a specific dimension. Tolerances are applied to individual features (e.g., hole diameter, wall thickness, distance between features) and are usually much tighter than general dimensional accuracy. They dictate the acceptable upper and lower limits for a dimension to ensure proper fit and function (e.g., 10.0 mm ±0.05 mm).
- Oberflächengüte (Oberflächenrauhigkeit): This quantifies the texture of a part’s surface. It’s typically measured as Ra (average roughness) or Rz (average maximum height of the profile) in micrometers (µm). Lower Ra/Rz values indicate a smoother surface. Surface finish impacts friction, wear, fatigue life, sealing, aesthetics, and potentially aerodynamics.
Typical Expectations for LPBF Aluminum (AlSi10Mg & Scalmalloy®):
It’s important to note that achievable precision depends heavily on the specific LPBF machine, its calibration, the chosen process parameters, the material batch, the part geometry and size, its orientation on the build plate, and the effectiveness of post-processing (especially heat treatment). However, we can provide general guidelines:
- Maßgenauigkeit:
- Wie gedruckt: For well-calibrated industrial LPBF systems printing aluminum alloys, general dimensional accuracy typically falls within the range of ±0.1 mm to ±0.2 mm for smaller dimensions (e.g., up to 100 mm), potentially increasing to ±0.1% to ±0.2% for larger dimensions. Factors like thermal warping during the build, especially on large, flat sections, can influence this. Met3dp emphasizes the
branchenführendes Druckvolumen, Genauigkeit und Zuverlässigkeit
of their printer systems, showcasing a commitment to achieving high levels of dimensional conformity. - Nachbearbeitet: Machining specific features after printing allows for much higher accuracy, essentially matching standard CNC machining capabilities where applied.
- Wie gedruckt: For well-calibrated industrial LPBF systems printing aluminum alloys, general dimensional accuracy typically falls within the range of ±0.1 mm to ±0.2 mm for smaller dimensions (e.g., up to 100 mm), potentially increasing to ±0.1% to ±0.2% for larger dimensions. Factors like thermal warping during the build, especially on large, flat sections, can influence this. Met3dp emphasizes the
- Toleranzen:
- Wie gedruckt: Holding tight tolerances directly off the printer is challenging. While general accuracy might be ±0.1 mm, achieving a specific tolerance like ±0.02 mm on a feature is usually not feasible without secondary operations. Tolerances of ±0.1 mm might be achievable on smaller, well-supported features, but ±0.2 mm or larger is more realistic for general as-printed features.
- Post-Processed (Machined): Critical features requiring tight tolerances (e.g., bearing housings, mating flanges, alignment pins) should be designed with machining stock and finished using CNC machining. Tolerances of ±0.01 mm to ±0.05 mm are readily achievable via post-machining, depending on the feature and machining process.
- Oberflächengüte (Rauhigkeit - Ra):
- Wie gedruckt: LPBF parts inherently have a somewhat rough, grainy texture due to the partially melted and sintered powder particles adhering to the surface. Typical Ra values range from 6 µm to 20 µm.
- Vertikale Mauern: Generally offer the best as-printed finish within this range.
- Upward-Facing Surfaces (Flat or Gently Sloped): Also tend to have a relatively good finish.
- Downward-Facing Surfaces (Overhangs/Supported): Typically exhibit the roughest surfaces due to contact with support structures, potentially exceeding 20 µm Ra.
- Nachbearbeitet: Significant improvements are possible:
- Perlstrahlen: Creates a uniform matte finish, typically Ra 3 µm to 10 µm.
- Taumeln/Gleitschleifen: Can achieve Ra 1 µm to 5 µm.
- Bearbeitungen: Produces smooth surfaces comparable to conventional machining, potentially Ra 0.8 µm to 3.2 µm or better.
- Polieren: Can achieve very low Ra values, < 0.8 µm, for mirror-like finishes.
- Wie gedruckt: LPBF parts inherently have a somewhat rough, grainy texture due to the partially melted and sintered powder particles adhering to the surface. Typical Ra values range from 6 µm to 20 µm.
Summary Table: Precision Capabilities for LPBF Aluminum
Parameter | Zustand | Typical Achievable Range | Anmerkungen |
---|---|---|---|
Maßgenauigkeit | Wie gedruckt | ±0.1 to ±0.2 mm (or ±0.1-0.2%) | Machine/process/geometry dependent |
Post-Machined | Dictated by machining capability | High accuracy on specific features | |
Toleranz | As-Printed (General) | ±0.2 mm or larger | Looser tolerances typical |
As-Printed (Optimized Feat.) | ±0.1 mm possible | Smaller features, ideal orientation | |
Post-Machined | ±0.01 to ±0.05 mm typical | Standard machining tolerances achievable | |
Oberflächengüte (Ra) | As-Printed (Vertical) | 6 – 15 µm | Best as-printed surfaces |
As-Printed (Down-facing) | 15 – 25+ µm | Rougher due to supports | |
Bead Blasted | 3 – 10 µm | Uniform matte finish | |
Tumbled/Vibratory | 1 – 5 µm | Smoother, edge refinement | |
Machined | 0.8 – 3.2 µm (or better) | Standard machined finish | |
Polished | < 0.8 µm | Mirror finish possible |
In Blätter exportieren
Faktoren, die die Präzision beeinflussen:
Achieving the best possible precision requires careful control over numerous factors:
- Maschinenqualität und Kalibrierung: Regular maintenance and precise calibration of the LPBF system are fundamental.
- Prozessparameter: Laser power, scan speed, layer thickness, hatching strategy, etc., all influence melt pool dynamics and final part quality.
- Qualität des Pulvers: Consistent particle size distribution, sphericity, and low moisture content, as emphasized by suppliers like Met3dp, are crucial for stable processing and dense parts.
- Wärmemanagement: Controlling heat buildup and dissipation during the print minimizes warping and residual stress.
- Strategie unterstützen: Robust supports prevent deformation during printing but can impact the surface finish of supported areas.
- Geometrie und Größe des Teils: Large, complex parts are generally more prone to deviation than smaller, simpler ones.
Managing Expectations and Communication:
For engineers and procurement managers, clear communication with the AM service provider is vital.
- Clearly indicate critical dimensions and required tolerances on drawings using Geometric Dimensioning and Tolerancing (GD&T).
- Distinguish between features that can accept as-printed tolerances and those requiring post-machining.
- Discuss surface finish requirements for different areas of the drone shell (e.g., aerodynamic surfaces vs. internal structures).
- Understand the provider’s specific capabilities and quality control procedures.
By understanding the achievable levels of tolerance, surface finish, and dimensional accuracy, and by designing parts with these capabilities and necessary post-processing steps in mind (DfAM), stakeholders can effectively utilize LPBF aluminum printing to produce high-quality, high-performance drone shells that meet demanding application requirements.
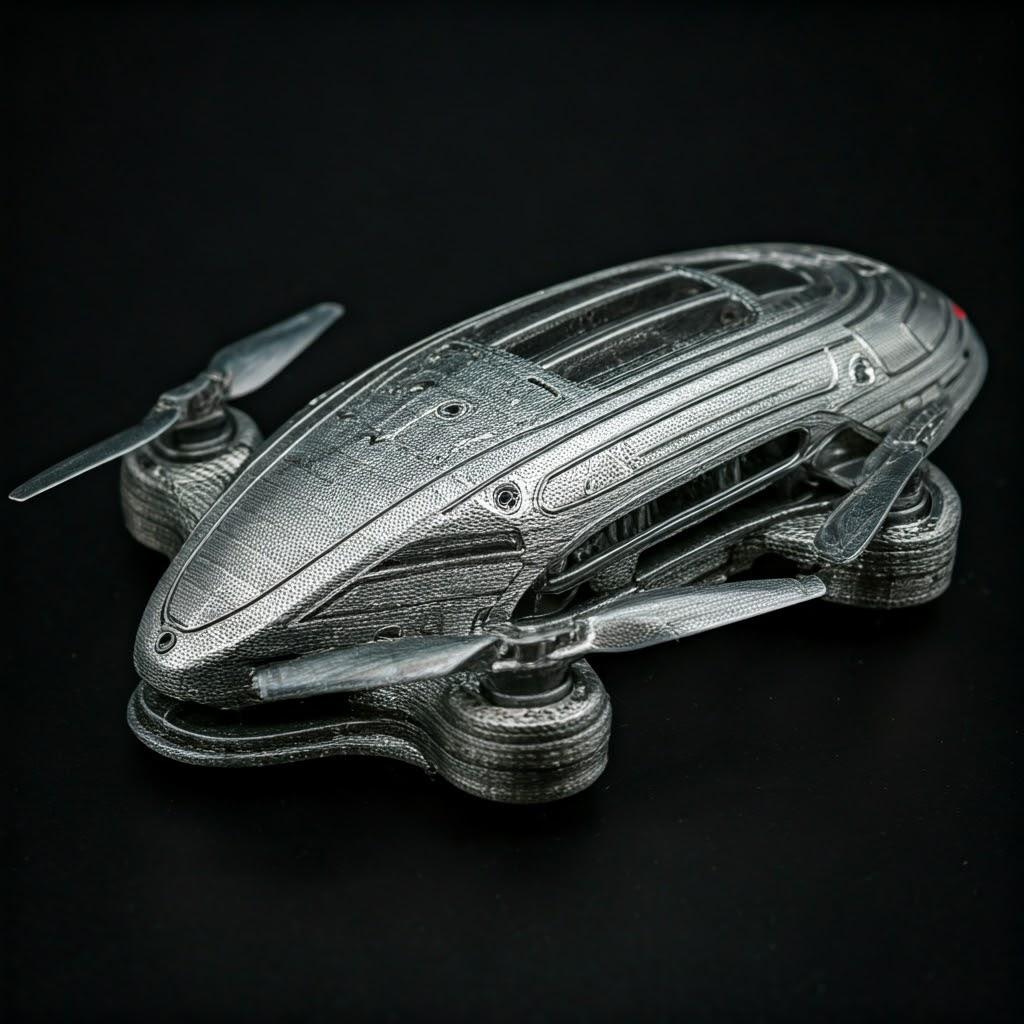
Essential Post-Processing Steps for 3D Printed Aluminum Drone Shells
A common misconception about metal 3D printing is that parts emerge from the printer ready for immediate use. In reality, for demanding applications like drone shells produced using LPBF with AlSi10Mg or Scalmalloy®, the printing process is just the first major step. A series of essential post-processing steps are required to transform the as-printed part into a functional, reliable component that meets dimensional specifications, achieves desired mechanical properties, and possesses the appropriate surface characteristics. Understanding these steps is crucial for engineers planning project timelines and for procurement managers evaluating the full scope of work and cost when engaging with AM service providers or dedicated post-processing suppliers
.
Here’s a breakdown of the typical post-processing workflow for 3D printed aluminum drone shells:
1. Stressabbau / Wärmebehandlung:
- Why it’s Critical: The rapid, localized heating and cooling cycles inherent in LPBF create significant residual stresses within the printed aluminum part. These internal stresses can cause warping or cracking (especially after removal from the build plate), dimensional instability over time, and can negatively impact fatigue life.
- Stressabbau: This is typically the very first step after the build finishes, often performed while the part is still attached to the build plate within the machine or immediately after removal in a separate furnace. It involves heating the part to a moderate temperature (e.g., around 300°C for AlSi10Mg for approximately 2 hours) followed by slow cooling. This allows the microstructure to relax, significantly reducing internal stresses without altering the fundamental hardness or strength dramatically.
- Solutionizing & Aging (e.g., T6 Treatment): To achieve the optimal mechanical properties (particularly yield strength and ultimate tensile strength) promised by alloys like AlSi10Mg and Scalmalloy®, a more comprehensive heat treatment is necessary. This typically involves:
- Lösungsfindung: Heating to a higher temperature (e.g., ~500-540°C) to dissolve alloying elements into the aluminum matrix.
- Abschrecken: Rapid cooling (e.g., in water or polymer) to lock the elements in solution.
- Künstliche Alterung: Reheating to a lower temperature (e.g., ~160-180°C) for a specific duration to allow controlled precipitation of strengthening phases (like Mg2Si in AlSi10Mg or Al3(Sc,Zr) in Scalmalloy®).
- Wichtigkeit: Skipping or improperly performing heat treatment can lead to parts that fail prematurely or do not meet design specifications. It is arguably the most critical post-processing step for structural integrity.
2. Entfernen des Teils von der Bauplatte:
- Prozess: The printed drone shell is fused to a thick metal build plate during the LPBF process. Separation is typically achieved using:
- Draht-Elektroerosion (Wire EDM): Offers high precision and minimal mechanical stress on the part, ideal for delicate structures.
- Bandsägen: A faster and often more cost-effective method for robust parts, though less precise. Requires careful handling.
- Erwägung: Allowance for the cutting process (kerf width) might need to be considered in the initial build setup.
3. Entfernung der Stützstruktur:
- Die Notwendigkeit: Overhanging features and parts requiring elevation from the build plate need support structures during printing. These metallic supports must be removed.
- Methoden: This can range from simple manual breaking or cutting for easily accessible supports to more complex and time-consuming methods for intricate or internal supports:
- Manual Tools: Pliers, cutters, grinders.
- Bearbeitungen: CNC milling can be used to precisely remove support interface layers.
- Hand Finishing: Files, rotary tools for detailed cleanup.
- Herausforderungen: Support removal can be labor-intensive and risks damaging the part surface if not done carefully. This highlights the importance of DfAM principles aimed at minimizing support needs and designing supports for easier removal.
4. Surface Finishing: As-printed surfaces are typically rough and may retain semi-sintered particles. Various techniques are used to achieve the desired finish:
- Strahlen (Perlstrahlen, Sandstrahlen, Sandkornstrahlen): The most common method. Propelling abrasive media (glass beads, aluminum oxide, etc.) at the surface removes loose powder, smooths roughness slightly, and creates a uniform, typically matte, cosmetic finish. Different media and pressures yield different textures.
- Taumeln / Vibrationsgleitschleifen: Parts are placed in a tub with abrasive media, which vibrates or tumbles. This process smooths surfaces and deburrs edges, effective for batches of smaller parts or achieving a more polished look than blasting alone.
- CNC-Bearbeitung: Used selectively on critical surfaces requiring tight tolerances, specific flatness, or very smooth finishes (Ra < 1.6 µm) that blasting or tumbling cannot achieve. This creates a hybrid manufacturing approach.
- Manual Polishing / Lapping: For applications demanding mirror finishes (e.g., specific aerodynamic requirements or aesthetic components), manual or automated polishing using progressively finer abrasives is employed. This is highly labor-intensive and costly.
- Elektropolieren: An electrochemical process that can smooth surfaces, though less common for structural drone shells than for medical or food-grade parts.
5. Cleaning: Thorough cleaning is necessary to remove any residual powder (especially from internal channels), cutting fluids from machining, blasting media, or polishing compounds. Ultrasonic cleaning baths are often used.
6. Inspection and Quality Control (QC):
- Prüfung der Abmessungen: Using calipers, micrometers, Coordinate Measuring Machines (CMMs), or 3D scanning to verify critical dimensions and tolerances against the design specifications.
- Überprüfung der Materialeigenschaften: Depending on criticality, sample coupons printed alongside the part may undergo tensile testing or hardness testing to verify heat treatment effectiveness and material properties.
- Zerstörungsfreie Prüfung (NDT): For flight-critical drone components, NDT methods may be required to detect internal defects:
- Computertomographie (CT) Scannen: Provides a 3D view of the internal structure, revealing porosity, inclusions, or cracks.
- Dye Penetrant Testing / Magnetic Particle Testing: Surface inspection methods (though less common for internal checks).
- Visuelle Inspektion: Checking for surface defects, incomplete support removal, etc.
The specific sequence and combination of these post-processing steps depend heavily on the drone shell’s design complexity, material (AlSi10Mg vs. Scalmalloy® might have slightly different heat treatment protocols), and the final application requirements regarding tolerances, finish, and structural integrity. Engaging with a full-service AM provider who possesses in-house capabilities or strong partnerships with trusted post-processing suppliers
ensures a streamlined workflow and accountability for the final part quality.
Overcoming Challenges: Ensuring Quality in Aluminum Drone Shell Printing
While metal additive manufacturing offers significant advantages for producing lightweight, complex aluminum drone shells, the technology is not without its challenges. Achieving consistent, high-quality results with alloys like AlSi10Mg and Scalmalloy® using Laser Powder Bed Fusion (LPBF) requires careful process control, material management, adherence to DfAM principles, and rigorous quality assurance. Recognizing potential issues and understanding how experienced AM providers mitigate them is crucial for both design engineers and procurement managers seeking reliable manufacturing partners.
Here are some common challenges encountered in aluminum LPBF and the strategies used to overcome them:
1. Verformung und Verzerrung:
- Herausforderung: The intense, localized heat from the laser followed by rapid cooling creates significant thermal gradients within the part during the build. This leads to the buildup of residual stress, which can cause the part to warp, curl, or distort, especially thin sections or large flat areas, potentially even detaching from supports mid-print.
- Lösungen:
- Optimierte Teileausrichtung: Orienting the part to minimize large, flat surfaces parallel to the build plate and reduce thermal mass concentrations.
- Robuste Unterstützungsstrategie: Designing supports not only to anchor overhangs but also to act as heat sinks, drawing heat away from critical areas and mechanically constraining the part during the build. Finite Element Analysis (FEA) simulation can help predict stress and optimize support placement.
- Optimierung der Prozessparameter: Fine-tuning laser power, scan speed, and scan strategy (e.g., island scanning) to manage heat input and reduce thermal gradients.
- Wirksamer Stressabbau: Performing a thermal stress relief cycle immediately after printing and before support removal is absolutely critical to relax internal stresses and stabilize the part’s geometry.
2. Reststress-Management:
- Herausforderung: Even if significant warping doesn’t occur during the print, high levels of residual stress can remain locked within the as-printed part. This can negatively impact fatigue life, lead to unexpected distortion during post-machining, and potentially cause delayed cracking.
- Lösungen:
- Obligatorische Wärmebehandlung: Appropriate stress relief and/or full solutionizing and aging heat treatments are essential not just for mechanical properties but fundamentally for reducing detrimental residual stresses.
- Build Plate Heating: Some LPBF machines utilize heated build platforms to reduce the thermal gradient between the melted material and the surrounding powder/part, thereby lowering stress buildup.
- Simulation & Design Adaptation: Predictive software can estimate residual stress distribution, allowing designers to make minor modifications (e.g., adding sacrificial ribs, adjusting thicknesses) to mitigate high-stress zones.
3. Support Removal Difficulty and Surface Quality:
- Herausforderung: Supports are necessary but removing them can be time-consuming, labor-intensive, and potentially damage the part surface, especially complex lattice or conformal supports in hard-to-reach internal areas. The interface between the support and the part often leaves a rougher surface finish.
- Lösungen:
- DfAM für die Minimierung der Unterstützung: Designing with self-supporting angles (>45°), using fillets instead of sharp overhangs, and orienting the part strategically are the first lines of defense.
- Optimiertes Support-Design: Using support types (e.g., thin-walled lattice, conical) that are easier to remove and have minimal contact points. Designing break-away features or specific access points for tools.
- Skilled Labor & Tools: Relying on experienced technicians with appropriate tools (hand tools, potentially specialized cutters or even localized machining) for careful support removal.
- Nachbearbeiten: Utilizing bead blasting or tumbling to improve the surface finish of supported areas after removal. Planning for machining if a very smooth finish is required on those surfaces.
4. Porosity Control:
- Herausforderung: Small voids or pores can sometimes form within the printed material due to incomplete melting, gas entrapment (e.g., dissolved hydrogen in aluminum), or powder inconsistencies (keyholing). Excessive porosity can degrade mechanical properties like fatigue strength and ductility.
- Lösungen:
- Optimierte Druckparameter: Developing robust parameter sets (laser power, speed, hatch spacing, layer thickness) validated to achieve high density (>99.5%, often >99.8%) for the specific alloy and machine.
- Hochwertiges Pulver: Using powder with consistent spherical morphology, controlled particle size distribution (PSD), good flowability, and low moisture/gas content. Rigorous powder handling and recycling protocols are essential. Met3dp's focus on producing high-quality spherical powders using advanced atomization techniques directly addresses this need.
- Kontrolle der inerten Atmosphäre: Maintaining a high-purity inert gas environment (Argon or Nitrogen) within the build chamber to minimize oxidation and gas pickup during melting.
- Heiß-Isostatisches Pressen (HIP): For critical applications demanding near-100% density, HIP (applying high temperature and isostatic gas pressure) can be used post-print to close internal pores. However, this adds significant cost and is less commonly required for aluminum AM compared to titanium or superalloys unless fatigue is absolutely critical.
- NDT-Inspektion: Using CT scanning to detect and quantify internal porosity in final parts for quality assurance.
5. Achieving Tight Tolerances and Smooth Finishes:
- Herausforderung: As discussed previously, the as-printed state typically has limitations regarding achievable tolerances and surface smoothness.
- Lösungen:
- Hybrid Manufacturing Approach: Accepting the as-printed limitations for non-critical features and incorporating planned post-machining steps for surfaces and features requiring high precision or specific smooth finishes.
- Appropriate Surface Treatments: Selecting the right post-processing method (blasting, tumbling, polishing) based on the required surface characteristics. Understanding the capabilities of various Druckverfahren and their inherent surface quality helps set realistic expectations.
- Clear Specification: Precisely defining tolerance and finish requirements on drawings using GD&T ensures the AM provider understands the critical aspects of the design.
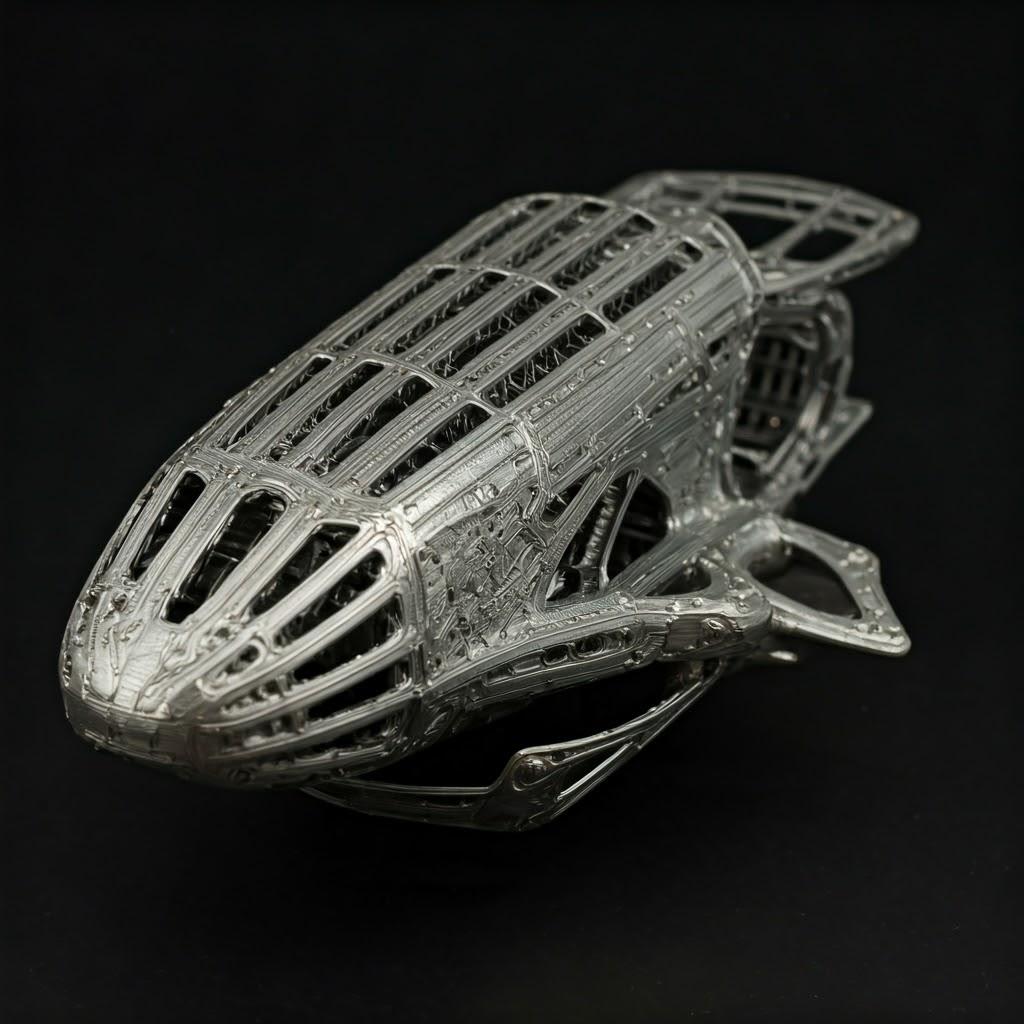
Quality Assurance as the Overarching Solution:
Overcoming these challenges consistently relies on a robust quality management system implemented by the AM service provider. This includes:
- Incoming powder inspection and traceability.
- Regular machine maintenance and calibration.
- In-process monitoring (melt pool monitoring, sensor data).
- Strict adherence to validated process parameters.
- Controlled post-processing procedures (especially heat treatment).
- Thorough final inspection using appropriate metrology and NDT methods.
By partnering with an experienced and quality-focused metal AM provider like Met3dp, who understands these challenges and implements rigorous processes to mitigate them – leveraging their expertise from powder production through to final part verification – engineers and procurement managers can confidently adopt 3D printed aluminum for demanding drone shell applications, ensuring both performance and Zuverlässigkeit
.
Choosing Your Partner: Selecting the Right Metal 3D Printing Service Provider for Drone Components
The decision to leverage the power of metal additive manufacturing for critical components like drone shells is significant. However, realizing the full potential of this technology—achieving optimal performance, ensuring consistent quality, and managing costs effectively—hinges critically on selecting the right manufacturing partner. Choosing a metal 3D printing service provider is not merely a transactional procurement decision; it’s about establishing a collaborative partnership, especially when dealing with complex, high-performance parts manufactured from advanced materials like AlSi10Mg and Scalmalloy®. For engineers demanding technical precision and procurement managers seeking reliable, long-term Lieferanten von Drohnenteilen
, a thorough evaluation process is essential.
Selecting an inadequate provider can lead to subpar part quality, missed deadlines, unexpected costs, and ultimately, compromised drone performance or project failure. Conversely, a capable and collaborative partner becomes an extension of your development team, offering expertise, ensuring quality, and helping you innovate.
Here are key criteria to consider when evaluating and selecting a metal AM service provider for your aluminum drone shell needs:
1. Technical Expertise and Proven Experience:
- Spezifität des Materials: Do they have demonstrable experience printing successfully with the specific aluminum alloy you require (AlSi10Mg or the more demanding Scalmalloy®)? Ask for evidence, such as datasheets derived from their machines or non-proprietary case studies.
- Technology Proficiency: Are they experts in Laser Powder Bed Fusion (LPBF)? What is their understanding of process parameter optimization for aluminum alloys?
- Industry Relevance: Have they worked on projects in demanding sectors like aerospace, defense, or automotive? Experience with UAV components specifically is a significant advantage. Look for providers like Met3dp, who explicitly state their focus on mission-critical parts and possess
jahrzehntelange Erfahrung in der additiven Fertigung von Metallen
. - Technische Unterstützung: Do they offer DfAM consultation? Can their engineers review your design for printability, suggest optimizations, and help troubleshoot potential issues?
2. Machine Capabilities, Capacity, and Quality:
- Ausrüstung: What specific LPBF machines do they operate? Are these industrial-grade systems known for reliability and accuracy with aluminum? (e.g., EOS, SLM Solutions, Trumpf, Renishaw, Velo3D, potentially Met3dp’s own SEBM printers if applicable or comparable LPBF systems).
- Bauvolumen: Is the machine’s build envelope large enough to accommodate your drone shell dimensions, potentially allowing for multiple parts per build (nesting)?
- Capacity & Redundancy: Do they have sufficient machine capacity to meet your required lead times for both prototypes and potential low-volume production? Do they have multiple compatible machines to mitigate risks associated with machine downtime?
- Prozessüberwachung: Do their machines incorporate in-process monitoring capabilities (e.g., melt pool monitoring, thermal imaging) that can provide insights into build quality and consistency?
3. Material Quality Control and Handling:
- Powder Sourcing & Certification: Where do they source their aluminum powders? Can they provide material certifications confirming compliance with relevant standards (e.g., ASTM)? Is the powder sourced from reputable suppliers, or do they, like Met3dp, have advanced in-house powder production capabilities ensuring tight control over quality? The Über uns page often details a company’s vertical integration and commitment to quality inputs.
- Pulvermanagement: What are their procedures for powder handling, storage (preventing moisture absorption, crucial for aluminum), sieving, blending, and recycling? Rigorous powder management is critical for consistent part quality.
4. Umfassende Post-Processing-Fähigkeiten:
- In-House vs. Outsourced: Which essential post-processing steps (stress relief/heat treatment, support removal, machining, surface finishing, cleaning) do they perform in-house? While outsourcing is common, in-house capabilities often lead to better integration, faster turnaround, and clearer accountability.
- Heat Treatment Expertise: Do they have properly calibrated furnaces and proven expertise in executing the specific heat treatment cycles required for AlSi10Mg (T6) and Scalmalloy® to achieve optimal properties? This is non-negotiable.
- Machining & Finishing: Do they have CNC machining capabilities for finishing critical features? What range of surface finishes can they provide (blasting, tumbling, polishing)?
5. Robust Quality Management System (QMS) & Certifications:
- ISO 9001: This certification is a baseline indicator of a documented QMS.
- AS9100: This is the critical standard for aerospace manufacturers and suppliers. If your drone shell is for aerospace or defense applications, AS9100 certification (or equivalent) is often mandatory, demonstrating rigorous quality control, traceability, and risk management processes.
- Rückverfolgbarkeit: Can they provide full material and process traceability for each part?
- Inspektionskapazitäten: What metrology equipment do they use (CMMs, 3D scanners, gauges)? Do they offer NDT services (e.g., CT scanning) if required for internal defect detection?
6. Engineering Collaboration and Design Support:
- DfAM Services: Do they proactively offer DfAM reviews and actively collaborate with your engineers to optimize designs for additive manufacturing?
- Simulation: Can they perform simulation (e.g., build process simulation for stress/distortion, topology optimization) to aid design and manufacturing planning?
- Kommunikation: Are they responsive, transparent, and easy to communicate with? Effective project management is key.
7. Lead Time, Responsiveness, and Scalability:
- Zitierte Vorlaufzeiten: Are their quoted lead times realistic and competitive for both prototypes and potential production runs?
- Flexibilität: Can they accommodate urgent requests or changes in schedule?
- Skalierbarkeit: If your project moves from prototype to low-volume production, can they scale their capacity accordingly?
8. Cost Structure and Overall Value:
- Transparente Preisgestaltung: Is their quoting clear and detailed, outlining costs for material, printing, support, post-processing, and any additional services? Beware of quotes that seem too low – they might omit necessary post-processing or compromise on quality checks.
- Wert-Angebot: Evaluate the overall value, considering not just the price per part but also the provider’s expertise, quality assurance, reliability, and support services. The cheapest option is rarely the best value for critical components.
Checkliste zur Lieferantenbewertung (Beispiel):
Bewertungskriterium | Bedeutung | Key Questions to Ask Provider |
---|---|---|
Technical Expertise (Alloy/LPBF) | Hoch | Provide examples/case studies? Detail experience with AlSi10Mg/Scalmalloy®? Describe DfAM support process? |
Machine Capability & Capacity | Hoch | What machines? Build volume? How many units? What is typical utilization/queue time? Process monitoring used? |
Material Quality & Handling | Hoch | Powder source & certs? Describe handling/storage/recycling protocols? In-house powder production (like Met3dp)? |
Post-Processing Capabilities | Hoch | Which steps in-house? Detail heat treatment process/equipment? Machining tolerance capability? Finishing options? |
QMS & Certifications (AS9100?) | High (esp. Aero) | Provide ISO 9001 cert? AS9100 cert? Describe traceability process? Detail inspection equipment & NDT capabilities? |
Engineering & Design Support | Mittel bis Hoch | Offer DfAM review? Simulation services available? How do you manage collaboration/communication? |
Lead Time & Responsiveness | Hoch | Standard lead times (proto/prod)? Expedite options? How are project updates communicated? |
Kosten und Wert | Hoch | Provide detailed quote breakdown? Explain pricing model? What QA steps are included standard? |
Location & Logistics | Niedrig bis mittel | Where are facilities located? What are typical shipping arrangements/costs? |
In Blätter exportieren
Selecting the right metal AM service provider is a strategic investment. By carefully evaluating potential partners against these criteria, focusing on demonstrated expertise, robust quality systems, and a collaborative approach, you can build a relationship that ensures the successful production of high-performance, reliable 3D printed aluminum drone shells, turning advanced manufacturing potential into tangible operational advantages. Look for partners who position themselves not just as printers, but as comprehensive solution providers in the additive manufacturing space.
Understanding Costs and Lead Times for 3D Printed Drone Shells
Additive manufacturing offers remarkable advantages for producing complex, lightweight drone shells, but understanding the associated costs and typical timelines is essential for effective project planning, budgeting, and managing expectations. Unlike traditional mass production methods with high initial tooling costs but low per-part costs at volume, metal AM generally has minimal setup cost but a higher per-part cost that decreases more gradually with quantity. Procurement managers seeking Großhandel Drohne Teile
pricing and engineers needing prototypes quickly must grasp the factors influencing these variables.
Key Cost Factors for 3D Printed Aluminum Drone Shells:
The final price of a 3D printed aluminum drone shell is an amalgamation of several contributing factors:
- Materialkosten:
- Pulver Preis: The raw material cost per kilogram. High-performance alloys like Scalmalloy® are significantly more expensive (often 5-10x or more) than standard AlSi10Mg.
- Materialverbrauch: This includes the material making up the final part plus the material used for support structures. Efficient DfAM aims to minimize both part volume and support volume.
- Powder Refresh/Recycling: Not all powder in the build chamber is used. Recycled powder must often be refreshed with virgin powder, and handling/sieving processes add overhead, which is factored into the material cost component. Providers with efficient powder management systems can offer better material utilization rates.
- Machine Time (Amortized Hourly Rate):
- Primary Driver: This is often the single largest cost component. Industrial metal LPBF machines represent a significant capital investment, and their operational costs (power, gas, maintenance) are substantial.
- Build Time Calculation: Determined by:
- Teilband: The total volume of material (part + supports) to be melted.
- Teilhöhe (Z-Höhe): Each layer adds time (recoating, laser scanning). Taller parts take longer, making orientation a key cost factor.
- Teil Komplexität: Intricate details or extensive lattice structures require more complex laser scan paths per layer.
- Nesting-Effizienz: How many parts can be printed simultaneously on a single build plate. Higher density reduces the machine time allocated per part.
- Maschinentarif: Varies depending on the provider, machine type, and location.
- Arbeitskosten:
- Vorverarbeitung: CAD file preparation, build layout planning (nesting, orientation, support generation). This requires skilled technicians or engineers.
- Machine Setup & Monitoring: Loading powder, setting up the build, monitoring the print process (though largely automated during the build itself).
- Nachbearbeiten: This is often the most labor-intensive phase and significantly impacts the final cost. It includes:
- Heat Treatment (furnace operation).
- Part Removal (sawing/EDM).
- Support Removal (can be very time-consuming for complex parts).
- Surface Finishing (blasting, tumbling, manual polishing).
- Machining (setup and operation of CNC machines).
- Inspection & QA (technician time for measurements, NDT, documentation).
- Design and Engineering Services (If Applicable):
- If the AM provider offers DfAM consultation, topology optimization services, or build simulation, these engineering hours will be added to the cost. Investing here can often lead to downstream savings through optimized designs.
- Qualitätssicherung und Prüfung:
- Standard dimensional inspection costs are usually factored in.
- More rigorous QA, such as NDT (CT scanning), material testing (tensile tests on witness coupons), or extensive CMM reporting, will add cost and should be specified if required.
- Overheads and Profit:
- Like any business, the service provider includes operational overheads (facility, utilities, administration) and a profit margin in their pricing.
- Quantity / Batch Size:
- Amortisation einrichten: Fixed costs (file prep, machine setup) are spread over more parts in larger batches, reducing the per-part cost.
- Nesting-Effizienz: Larger batches often allow for more efficient packing of the build volume, reducing machine time per part.
- Post-Processing Efficiency: Handling parts in batches can sometimes streamline post-processing steps.
- Wholesale Pricing: For recurring orders or significantly large batches,
Großhandel Drohne Teile
pricing structures may be negotiable, but AM’s cost scaling differs from injection molding.
Table: Breakdown of Cost Factors for 3D Printed Aluminum Drone Shell
Kostenfaktor | Beschreibung | Typical Impact on Total Cost | Anmerkungen |
---|---|---|---|
Materialkosten | Price of AlSi10Mg or Scalmalloy® powder consumed (part + supports) | Mittel bis Hoch | Scalmalloy® significantly higher. Minimize volume/supports. |
Maschinenzeit | Hourly rate applied to total build time (Z-height, volume, complexity) | Hoch | Optimize orientation, nesting. Often the largest single factor. |
Labor: Setup | Build file preparation, machine setup | Niedrig bis mittel | Can be amortized over batches. |
Labor: Post-Processing | Heat treat, support removal, finishing, machining, inspection | Mittel bis Hoch | Highly dependent on complexity & requirements. Often underestimated. |
Engineering Services | DfAM consultation, simulation (if requested) | Low to Medium (Optional) | Can provide long-term savings. |
Qualitätssicherung | Standard inspection; NDT or advanced metrology adds cost | Low to High (Requirement Dep.) | Specify needs clearly. |
Menge | Number of identical parts ordered | Mittel | Per-part cost decreases with volume, but less dramatically than mass prod. |
Overheads & Profit | Provider’s operational costs and margin | Mittel | Standard business practice. |
In Blätter exportieren
Verständnis der Vorlaufzeiten:
AM lead time
is another critical factor influenced by several stages:
- Angebotserstellung und Auftragsbestätigung: Typically 1-3 business days, assuming clear input data.
- Design Review & File Prep: 1-2 days, potentially longer if DfAM optimization is needed.
- Warteschlangenzeit der Maschine: Highly variable (days to weeks) depending on the provider’s current workload and machine availability. This is often the biggest uncertainty.
- Druckzeit: Ranges from ~12 hours for small parts to several days (e.g., 48-120+ hours) for large, complex drone shells or densely packed build plates.
- Cooling & Heat Treatment: Parts need to cool before removal. Heat treatment cycles (stress relief + aging) can add 1-2 days, including furnace time and controlled cooling.
- Nachbearbeiten: This can add significant time:
- Part/Support Removal: Hours to days, depending on complexity.
- Machining: Depends on complexity and machine shop scheduling (can add days or weeks if outsourced).
- Finishing/Inspection: 1-3 days typically.
- Versand: Standard courier/freight times (1-5 days domestically, longer internationally).
Typische Vorlaufzeitspannen (Schätzungen):
- Prototypes (1-5 units): 1 to 3 weeks is common, assuming available machine capacity and standard post-processing. Can be expedited for a premium.
- Kleinserienproduktion (10-100 Einheiten): 3 bis 6 Wochen might be typical, allowing for optimized nesting and batch post-processing. Highly dependent on part size and complexity.
Das Wichtigste zum Mitnehmen: Both cost and lead time in metal AM are heavily influenced by design complexity, part size (especially height), material choice, required precision/finish, and the service provider’s capacity and efficiency. Early engagement with potential providers and clear communication of requirements are essential for obtaining accurate quotes and realistic timelines for your 3D printed aluminum drone shell project.
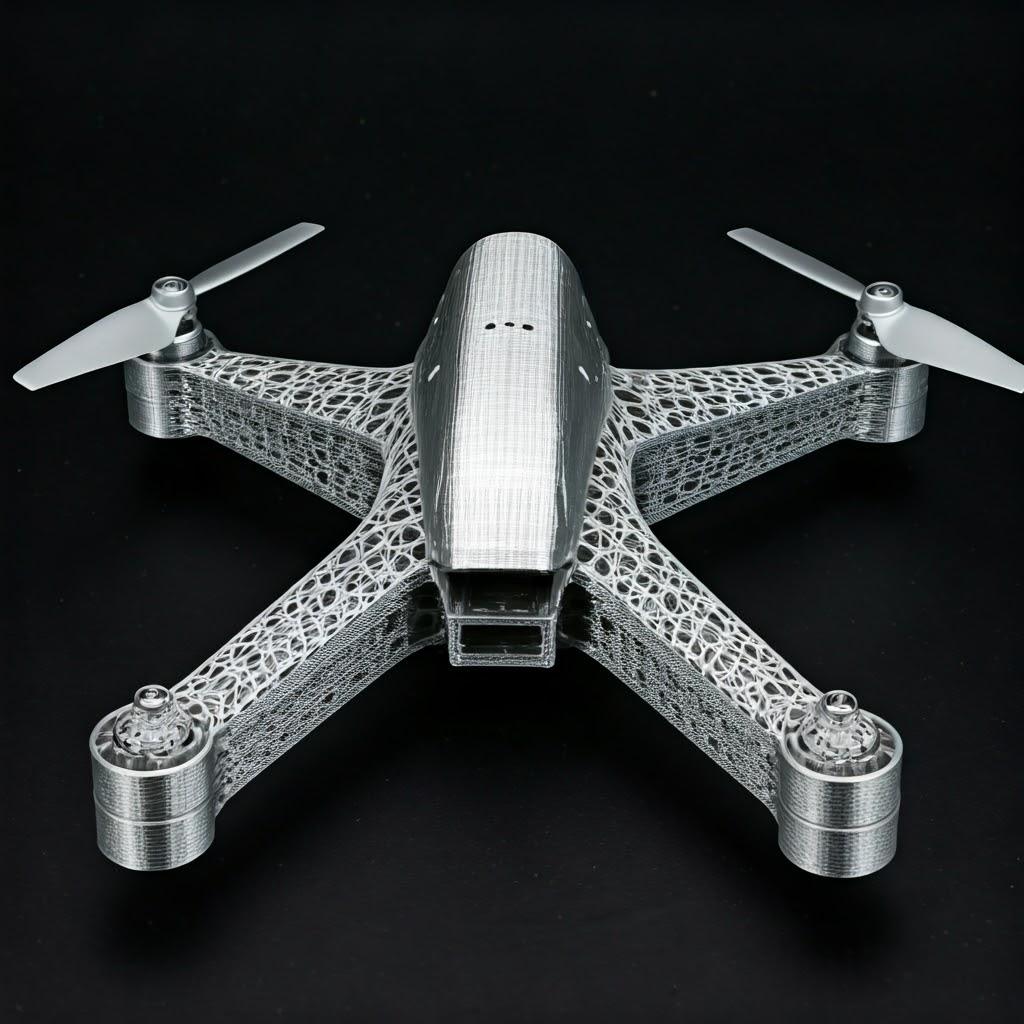
Frequently Asked Questions (FAQ) about 3D Printed Aluminum Drone Shells
As engineers and procurement managers explore the use of metal additive manufacturing for drone components, several common questions arise. Here are answers to some frequently asked questions regarding 3D printed aluminum (AlSi10Mg and Scalmalloy®) drone shells:
Q1: How does the strength of 3D printed AlSi10Mg / Scalmalloy® compare to traditionally machined aluminum alloys like 6061-T6 or 7075-T6?
A: The comparison depends heavily on the specific printed alloy and the post-print heat treatment applied.
- AlSi10Mg (Heat Treated – T6 equivalent): Generally exhibits Yield Strength (YS) and Ultimate Tensile Strength (UTS) slightly lower than or comparable to wrought 6061-T6 aluminum. Its elongation (ductility) is often lower than 6061-T6. It’s significantly less strong than 7075-T6. However, its strength-to-weight ratio is still very favorable for many drone applications, and AM allows complex geometries often unachievable with machining 6061.
- Scalmalloy® (wärmebehandelt): This high-performance AM alloy truly shines. Its Yield Strength and UTS significantly exceed those of 6061-T6 and are comparable to, or even slightly exceed, those of high-strength 7075-T6 aluminum, particularly when considering specific strength (strength divided by density). Furthermore, Scalmalloy® generally offers better ductility and fatigue properties than 7075, making it an exceptional choice for demanding, lightweight structural components where performance rivals or surpasses traditional high-strength aluminum options.
Q2: What are the main cost drivers I should focus on minimizing when designing a drone shell for metal AM?
A: To manage costs effectively when designing for aluminum LPBF, focus on these key areas:
- Minimize Part Volume: Employ topology optimization and lattice structures judiciously to remove unnecessary material. Smaller volume means less powder consumed and faster printing.
- Minimize Part Height (Z-axis): Orient the part on the build plate to minimize its height, as this directly impacts print time (layer count). Consider splitting very tall parts if feasible, though this negates part consolidation benefits.
- Design for Minimal Supports: Utilize self-supporting angles (typically >45°) wherever possible. Avoid large horizontal overhangs or design them with self-supporting shapes (e.g., chamfers, teardrops). Supports consume material, add print time, and require significant post-processing labor to remove.
- Specify Tolerances and Finishes Appropriately: Only call out tight tolerances (requiring post-machining) and smooth surface finishes (requiring extra finishing steps) on features where they are functionally necessary. Over-specifying precision dramatically increases post-processing costs.
- Teil Konsolidierung: While potentially increasing the complexity of a single part, consolidating multiple components into one print can eliminate assembly labor and fastener costs, often leading to overall savings, particularly for smaller production runs.
Q3: Can complex internal features or channels within a drone shell be effectively cleaned of residual powder?
A: Yes, but it requires careful design and diligent post-processing. Residual powder trapped in internal cavities can be detrimental.
- Überlegungen zum Design: Ensure internal channels have sufficient diameter (typically > 1-2 mm) and include strategically placed outlet holes to allow powder to escape during the build and cleaning process. Avoid complex dead-end pockets where powder can become permanently trapped.
- Cleaning Methods: Post-print cleaning involves using compressed air, vibration, and sometimes specialized vacuums or flushing techniques to remove powder from internal geometries. Ultrasonic cleaning can also help dislodge particles.
- Verifizierung: For critical channels (e.g., cooling passages), inspection methods like endoscopy or even CT scanning might be employed to verify complete powder removal, although this adds cost. Discuss cleaning strategies and limitations with your AM provider.
Q4: Is 3D printed aluminum suitable for drone parts exposed to harsh environments, such as saltwater spray or industrial chemicals?
A: Aluminum alloys, including AlSi10Mg and Scalmalloy®, offer good inherent corrosion resistance due to the formation of a passive oxide layer. They generally perform well in typical atmospheric conditions. However, for prolonged exposure to aggressive environments like saltwater (marine drones) or specific chemicals:
- Base Resistance: Both alloys hold up reasonably well, but are susceptible to pitting corrosion in chloride-rich environments over time.
- Oberflächenbehandlungen: Applying protective surface treatments during post-processing is highly recommended for enhanced durability in harsh conditions. Common options include:
- Eloxieren: Creates a thicker, harder, more corrosion-resistant oxide layer. Can also be colored.
- Conversion Coatings (e.g., Chromate, TCP): Provide good corrosion protection and act as an excellent primer for paint.
- Anstrich/Pulverbeschichtung: Offers a robust barrier against the environment.
- Empfehlung: Discuss the specific operating environment with your AM provider to select the most appropriate alloy and determine if additional protective coatings are necessary.
Q5: What information do I absolutely need to provide to a metal AM service provider to get an accurate quote for my drone shell part?
A: To receive a timely and accurate quote, provide the following:
- 3D-CAD-Datei: A high-quality 3D model, preferably in STEP (.stp or .step) format. Avoid mesh files (like .stl) if possible, as they lack the precise geometric data needed for manufacturing planning.
- Spezifikation des Materials: Clearly state the desired aluminum alloy (e.g., AlSi10Mg or Scalmalloy®).
- Menge: Specify the number of parts required (for prototype or production run).
- Critical Tolerances & Finishes: Ideally, provide a 2D drawing (e.g., PDF) with Geometric Dimensioning and Tolerancing (GD&T) indicating critical dimensions, required tolerances, and specific surface finish requirements (Ra values) for key features. If no drawing is provided, the provider will likely quote based on their standard “as-printed” capabilities, which may not meet your needs.
- Wärmebehandlung: Specify if a particular heat treatment condition is required (e.g., T6 or equivalent for AlSi10Mg, specific aging for Scalmalloy®), or if standard stress relief is sufficient.
- Post-Processing-Bedarf: Indicate any mandatory post-processing like specific machining operations, required surface treatments (anodizing, painting), or NDT inspection.
- Gewünschtes Lieferdatum: Mention your target timeline.
Providing comprehensive information upfront minimizes delays and ensures the quote accurately reflects the work required to produce drone shells meeting your specifications.
Conclusion: Elevating UAV Capabilities with 3D Printed Lightweight Aluminum Shells
The landscape of Unmanned Aerial Vehicles is one of relentless innovation, driven by the continuous pursuit of greater performance, expanded capabilities, and enhanced operational efficiency. As we’ve explored throughout this discussion, the drone shell – the airframe itself – plays a pivotal role in achieving these goals. The advent of metal additive manufacturing, specifically Laser Powder Bed Fusion applied to advanced lightweight aluminum alloys like AlSi10Mg and the exceptional Scalmalloy®, represents a paradigm shift in how these critical structures are designed and produced.
The advantages are compelling and directly address the core challenges faced by UAV designers:
- Unprecedented Lightweighting: Through topology optimization and intricate lattice structures impossible with traditional methods, metal AM enables significant weight reductions, directly translating to longer flight endurance, increased payload capacity, and improved maneuverability.
- Geometrische Freiheit: Designers are liberated from the constraints of molds and machining access, allowing for highly complex, aerodynamically efficient shapes, integrated functional features (mounts, channels), and optimized internal structures.
- Teil Konsolidierung: The ability to combine multiple components into a single printed part reduces assembly time, minimizes weight associated with fasteners, and enhances overall structural integrity by eliminating potential failure points.
- Leistung des Materials: Access to high-strength-to-weight aluminum alloys, often with mechanical properties meeting or exceeding traditional counterparts after heat treatment, ensures structural robustness without a weight penalty.
- Rapid Iteration and Customization: The tooling-free nature of AM accelerates prototyping cycles and makes low-volume production of customized or mission-specific drone shells economically viable.
These technological benefits are not merely theoretical; they are enabling tangible advancements across diverse UAV applications, from demanding aerospace and defense missions requiring maximum performance and durability, to industrial inspection and delivery drones prioritizing efficiency and payload capacity, and specialized platforms for emergency services and scientific research.
However, harnessing these benefits requires a holistic approach. It demands embracing Design für additive Fertigung (DfAM) principles, understanding the achievable levels of precision and surface finish, meticulously planning for essential post-processing steps like heat treatment and finishing, and acknowledging and mitigating potential manufacturing challenges durch robuste Prozesskontrolle und Qualitätssicherung.
Critically, success hinges on Auswahl des richtigen Produktionspartners. Selecting a metal AM service provider with proven expertise in aluminum alloys, state-of-the-art equipment, comprehensive post-processing capabilities, rigorous quality systems (including relevant certifications like AS9100 where needed), and a collaborative engineering approach is paramount. Understanding the cost drivers and lead time factors allows for realistic budgeting and project planning.
The future of drone manufacturing is increasingly intertwined with additive manufacturing. As AM technologies continue to mature, offering faster print speeds, larger build volumes, enhanced material properties, and more sophisticated design and simulation tools, their role in producing high-value, performance-critical UAV components like drone shells will only grow. Adopting metal AM is no longer just an alternative manufacturing method; it is becoming a strategic capability for organizations aiming to lead in the rapidly evolving world of unmanned aerial systems.
For companies looking to explore the transformative potential of metal 3D printing for their drone projects, partnering with a knowledgeable and vertically integrated provider is key. Met3dp, with its deep expertise spanning advanced metal powder production, industry-leading printing equipment delivering accuracy and reliability, and comprehensive application development services, stands ready to assist. As a leader committed to enabling next-generation manufacturing, Met3dp offers the capabilities and partnership needed to turn complex designs into high-performance realities.
We invite engineers, designers, and procurement managers to delve deeper into the possibilities. Explore how Met3dp can empower your organization’s additive manufacturing goals and help you create lighter, stronger, and more capable drone solutions for the demanding applications of today and tomorrow.
Teilen auf
Facebook
Twitter
LinkedIn
WhatsApp
E-Mail
MET3DP Technology Co., LTD ist ein führender Anbieter von additiven Fertigungslösungen mit Hauptsitz in Qingdao, China. Unser Unternehmen ist spezialisiert auf 3D-Druckgeräte und Hochleistungsmetallpulver für industrielle Anwendungen.
Fragen Sie an, um den besten Preis und eine maßgeschneiderte Lösung für Ihr Unternehmen zu erhalten!
Verwandte Artikel
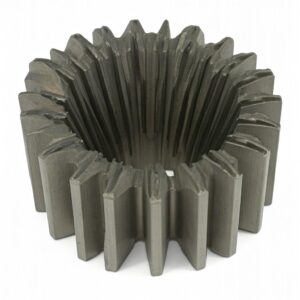
Hochleistungs-Düsenschaufelsegmente: Revolutionierung der Turbineneffizienz mit 3D-Metalldruck
Mehr lesen "
Mai 13, 2025
Keine Kommentare
Über Met3DP
Aktuelles Update
Unser Produkt
KONTAKT US
Haben Sie Fragen? Senden Sie uns jetzt eine Nachricht! Wir werden Ihre Anfrage mit einem ganzen Team nach Erhalt Ihrer Nachricht bearbeiten.
Holen Sie sich Metal3DP's
Produkt-Broschüre
Erhalten Sie die neuesten Produkte und Preislisten
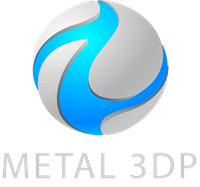
Metallpulver für 3D-Druck und additive Fertigung
UNTERNEHMEN
PRODUKT
cONTACT INFO
- Qingdao Stadt, Shandong, China
- [email protected]
- [email protected]
- +86 19116340731