3D Printed Surgical Cutting Guides in Stainless Steel
Innehållsförteckning
Introduction: Revolutionizing Surgical Precision with Metal 3D Printed Cutting Guides
Surgery, by its very nature, demands the highest levels of precision and accuracy. Every millimeter matters, and the tools used must enable surgeons to execute complex procedures with confidence and predictability. In recent years, a technological revolution has been unfolding in the operating room, driven by the power of metalltillsatstillverkning (AM), commonly known as metal 3D-utskrifter. One of the most impactful applications of this technology is the creation of patient-specific surgical cutting guides.
These guides, meticulously designed based on individual patient anatomy derived from CT or MRI scans, act as bespoke templates during surgery. They precisely direct surgical instruments like saws or drills, ensuring cuts and holes are made exactly according to the pre-operative plan. Traditionally, manufacturing such complex, custom devices involved lengthy and costly subtractive machining processes, often limiting their use to the most complex cases. Men.., 3D-utskrift av metall changes the paradigm entirely.
By building cutting guides layer by layer directly from medical-grade metal powders like 316L stainless steel or Ti-6Al-4V ELI, manufacturers can produce highly intricate, patient-matched instruments efficiently and cost-effectively. This capability is transforming procedures across various surgical specialties, offering tangible benefits:
- Enhanced Surgical Accuracy: Guides conform perfectly to the patient’s unique bone structure, minimizing guesswork and improving the accuracy of bone resections, osteotomies, and implant placements.
- Minskad operationstid: Pre-planned cuts guided by the template can significantly shorten procedure times, leading to less time under anesthesia for the patient and improved operating room efficiency.
- Improved Patient Outcomes: Greater accuracy and potentially shorter procedures can contribute to better functional outcomes, faster recovery times, and reduced complication rates.
- Facilitation of Complex Procedures: Patient-specific guides can make complex reconstructive or corrective surgeries more predictable and manageable.
For procurement managers and engineers in the medical device industry, understanding the potential of metal AM for producing surgical cutting guides is crucial. Sourcing these components requires partnering with knowledgeable tillverkning av medicintekniska produkter suppliers who possess expertise not only in printing technology but also in material science, regulatory requirements, and quality control. Companies like Met3dp, with their advanced powder production capabilities and industry-leading printing systems, are at the forefront of enabling this surgical innovation. This article delves into the applications, advantages, materials, design considerations, and supplier selection criteria for leveraging metal 3D printing to produce high-performance surgical cutting guides.
Applications: Where are Metal 3D Printed Cutting Guides Utilized?
The ability to create patient-specific, complex geometries makes metal 3D printed cutting guides invaluable across a wide spectrum of surgical disciplines. Their use streamlines procedures, enhances precision, and allows surgeons to tackle challenging anatomical variations with greater confidence. Key application areas include:
1. Orthopedic Surgery: This is arguably the largest field utilizing 3D printed cutting guides.
- Knee Arthroplasty (Replacement): Patient-specific guides ensure accurate femoral and tibial bone resections, crucial for optimal implant alignment, joint kinematics, and long-term implant survival. They help achieve the desired mechanical axis and rotational alignment tailored to the individual.
- Hip Arthroplasty: Guides can assist in precise acetabular reaming and femoral preparation, particularly in complex primary cases or revision surgeries where landmarks are distorted.
- Shoulder Arthroplasty: Accurate glenoid component placement is critical for stability and range of motion. 3D printed guides help navigate complex glenoid morphology and version correction.
- Corrective Osteotomies: For procedures like high tibial osteotomy (HTO) or distal femoral osteotomy (DFO) to correct limb alignment, guides ensure the precise location, orientation, and depth of the bone cuts.
- Trauma Surgery: In complex fracture fixation, guides can help plan screw trajectories and ensure accurate reduction, especially around joints like the ankle, wrist, or pelvis.
- Spine Surgery: Guides assist in accurate pedicle screw placement, reducing the risk of nerve root or spinal cord injury, particularly in patients with abnormal anatomy or in minimally invasive procedures.
2. Craniomaxillofacial (CMF) Surgery:
- Orthognathic Surgery (Jaw Correction): Cutting guides ensure precise osteotomies of the maxilla and mandible, allowing for accurate repositioning of the jaw segments according to the virtual surgical plan.
- Mandibular/Maxillary Reconstruction: Following tumor resection or trauma, patient-specific cutting guides facilitate accurate bone harvesting (e.g., from the fibula) and precise shaping of the graft and recipient site for optimal fit and facial symmetry.
- Distraction Osteogenesis: Guides can direct the placement of distractors and the initial osteotomy cuts.
3. Neurosurgery:
- Cranial Procedures: While less common than in orthopedics, guides can assist in planning craniotomies or defining trajectories for biopsies or electrode placements, especially near critical structures.
4. Podiatric Surgery:
- Foot and Ankle Deformity Correction: Guides aid in complex osteotomies for correcting conditions like bunions (hallux valgus) or flatfoot deformities, ensuring accurate angular corrections.
Key Functions and Advantages in These Applications:
- Patient-Specific Fit: Guides perfectly match the contours of the patient’s bone surface, providing stable and reliable referencing.
- Pre-Planned Accuracy: Translates the virtual surgical plan directly to the operating room, minimizing intraoperative decision-making regarding cut location and orientation.
- Reduced Reliance on Fluoroscopy: In some cases, the accuracy of the guides can reduce the need for intraoperative X-rays, lowering radiation exposure for both patient and staff.
- Effektivitet: Streamlines the surgical workflow by eliminating steps involved in traditional freehand techniques or the use of standard instrumentation.
The demand for reliable custom surgical instruments och surgical planning tools is growing, and medical device suppliers capable of providing high-quality, additively manufactured solutions are becoming essential partners for hospitals and surgical centers globally. Sourcing orthopedic surgery guides eller maxillofacial cutting jigs increasingly involves evaluating a supplier’s expertise in metal 3D printing technologies and materials.
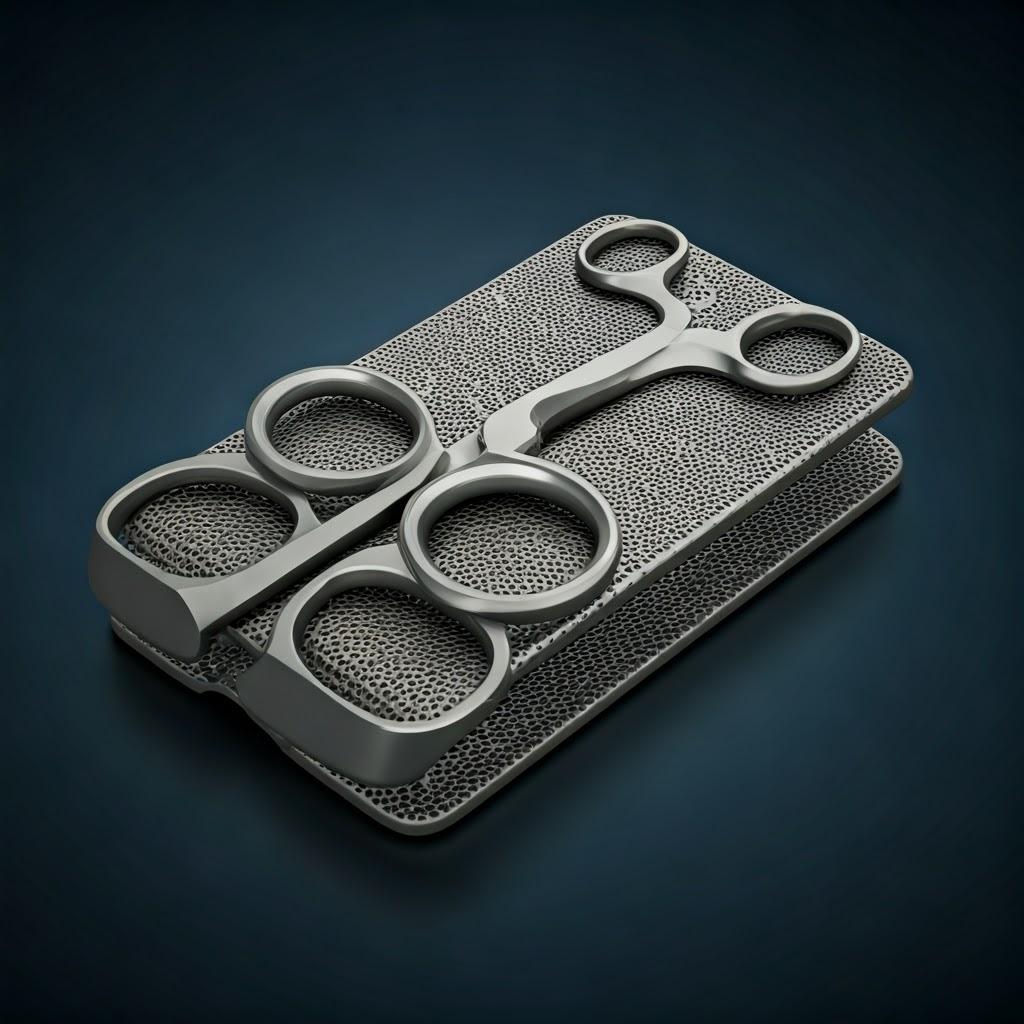
Advantages: Why Choose Metal Additive Manufacturing for Surgical Guides?
While traditional manufacturing methods like CNC machining can produce cutting guides, additiv tillverkning av metall offers distinct advantages that make it particularly well-suited for this application, especially when patient-specificity and complex designs are required. Procurement specialists and design engineers should consider these key benefits when evaluating production methods:
1. Unparalleled Design Freedom & Complexity:
- Patient-Specific Adaptation: AM excels at creating organic, freeform shapes that perfectly conform to the unique contours of a patient’s bone anatomy. This level of customization is extremely difficult, time-consuming, and often cost-prohibitive with traditional machining.
- Integrated Features: Complex features like internal channels (e.g., for irrigation or cooling), specific drill hole angulations, multiple cutting slots in varying orientations, and integrated referencing points can be built directly into the guide design without the constraints imposed by tool access in machining.
- Topology Optimization & Lightweighting: Algorithms can be used to optimize the guide’s structure, removing material where it’s not needed while maintaining stiffness and strength. This can result in lighter, less bulky guides without compromising performance, often incorporating intricate lattice structures that are impossible to machine.
2. Rapid Prototyping and Iteration:
- Faster Design Cycles: New guide designs or iterations based on surgeon feedback can be printed and evaluated much faster than waiting for machined prototypes. This accelerates the development and refinement process.
- On-Demand-produktion: AM allows for decentralized or localized manufacturing, potentially enabling quicker turnaround times for patient-specific guides compared to relying on centralized machining facilities.
3. Suitability for Low-to-Medium Volume Production:
- Cost-Effective Customization: For patient-specific instruments (volume = 1), AM avoids the high setup costs and programming time associated with CNC machining each unique part. The cost per part is less sensitive to volume changes at the low end.
- Tool-Less Manufacturing: AM does not require specific molds, jigs, or fixtures for each unique guide design, reducing tooling costs and lead times significantly.
4. Material Properties:
- Medical-Grade Metals: AM processes like Selective Electron Beam Melting (SEBM) or Selective Laser Melting (SLM) can process well-characterized, biocompatible metals like 316L stainless steel and Ti-6Al-4V ELI, achieving material properties (density, strength) comparable or even superior to wrought materials. Met3dp utilizes advanced gas atomization and PREP technologies to produce high-sphericity powders essential for achieving these dense, high-quality parts.
- Near-Net Shape: Parts are built layer by layer close to their final dimensions, minimizing material waste compared to subtractive machining where significant material is removed from a solid block.
5. Potential for Consolidated Assemblies:
- Del Konsolidering: In some cases, multiple components of a guiding system could potentially be consolidated into a single, more complex 3D printed part, simplifying assembly and reducing potential points of failure.
Comparison: Additive Manufacturing vs. CNC Machining for Surgical Guides
Funktion | Additiv tillverkning av metall (AM) | Traditionell CNC-bearbetning |
---|---|---|
Designens komplexitet | High (Organic shapes, internal channels, lattices) | Moderate (Limited by tool access & paths) |
Patient-Specificity | Excellent (Ideal for unique geometries) | Possible, but often costly & time-consuming |
Installationskostnad | Low (Primarily digital setup) | High (Programming, fixturing) |
Verktygskostnad | Ingen | Required (Cutting tools, potentially fixtures) |
Lead Time (Custom) | Potentially Shorter (Especially for complex parts) | Can be longer due to programming & setup |
Material Avfall | Low (Near-net shape) | High (Subtractive process) |
Volume Sweet Spot | Low to Medium (Prototypes, custom, small batches) | Medium to High (Mass production) |
Surface Finish (As-Built) | Typically Rougher | Typically Smoother |
Efterbearbetning | Often Required (Support removal, finishing) | Minimal (Deburring, cleaning) |
Export to Sheets
While AM offers compelling advantages, it’s important to note that post-processing steps (like support removal, surface finishing, and potentially heat treatment) are often necessary to achieve the required tolerances and surface quality for surgical instruments. However, for complex, patient-specific surgical tools, den benefits of 3D printed guides—particularly the geometric freedom and customization potential—often outweigh the additional post-processing requirements, leading to improved surgical outcomes and enabling procedures previously considered too challenging. Choosing a knowledgeable metal 3D printing service provider like Met3dp ensures access to both advanced printing capabilities and the necessary expertise in materials and post-processing for medical applications.
Material Focus: 316L Stainless Steel and Ti-6Al-4V ELI for Optimal Performance
The selection of the right material is paramount for any medical device, especially for surgical instruments like cutting guides that come into contact with tissue and bone, and must withstand the rigors of the operating room environment, including sterilization. For metal 3D printed surgical guides, two materials stand out due to their proven track record, excellent biocompatibility, mechanical properties, and processability via additive manufacturing: 316L rostfritt stål och Titanium alloy Ti-6Al-4V ELI.
1. 316L Stainless Steel (UNS S31603): The Workhorse Surgical Alloy
- Sammansättning: An austenitic stainless steel alloy containing chromium (Cr), nickel (Ni), and molybdenum (Mo). The ‘L’ denotes low carbon content (<0.03%), which improves weldability and reduces the risk of sensitization (chromium carbide precipitation) during thermal processes, enhancing corrosion resistance.
- Viktiga egenskaper:
- Utmärkt biokompatibilitet: Long history of safe use in temporary and permanent implants and surgical instruments. Meets ISO 10993 and ASTM F138/F139 standards for surgical implant applications (when processed correctly).
- Hög korrosionsbeständighet: The passive chromium oxide layer provides excellent resistance to corrosion in bodily fluids and during sterilization (e.g., autoclaving). Molybdenum further enhances resistance to pitting and crevice corrosion.
- Good Mechanical Strength and Ductility: Offers a good balance of strength, toughness, and workability. Sufficiently strong and stiff for the demands placed on cutting guides.
- Kostnadseffektivitet: Generally less expensive than titanium alloys, making it a viable option for single-use or limited-reuse guides.
- Processbarhet: Well-established parameters exist for processing 316L via SLM and SEBM, achieving high density (>99.5%) and good mechanical properties.
- Överväganden:
- Nickel Sensitivity: Although rare, some patients may have nickel allergies.
- Densitet: Significantly denser (approx. 8 g/cm³) than titanium alloy.
- MRI Compatibility: It is non-magnetic but can cause artifacts in MRI scans, although this is less critical for temporary-contact guides than for permanent implants.
2. Ti-6Al-4V ELI (Grade 23) (UNS R56401): The Premium Implant Standard
- Sammansättning: An alpha-beta titanium alloy containing Aluminum (Al) and Vanadium (V). ‘ELI’ stands for Extra Low Interstitials (Oxygen, Nitrogen, Carbon), which significantly improves the alloy’s ductility and fracture toughness, particularly at cryogenic temperatures, but also beneficial for medical applications.
- Viktiga egenskaper:
- Exceptional Biocompatibility: Considered one of the most biocompatible metals, widely used for permanent implants (hips, knees, dental) and surgical tools. Excellent tissue and bone integration (osseointegration), though less critical for temporary guides. Meets ISO 10993 and ASTM F136 standards.
- Överlägset korrosionsmotstånd: Forms a highly stable and passive titanium dioxide (TiO₂) layer, making it virtually immune to corrosion in the body environment.
- Högt förhållande mellan styrka och vikt: Offers strength comparable to or exceeding 316L but at nearly half the density (approx. 4.4 g/cm³). This results in lighter, less cumbersome instruments.
- Excellent Fatigue Strength: Important for instruments subjected to cyclic loading, although less critical for single-use guides.
- MRI and CT Compatibility: Non-magnetic and generally produces fewer artifacts in medical imaging compared to stainless steel.
- Överväganden:
- Högre kostnad: Titanium alloys and their powders are significantly more expensive than stainless steel.
- Reaktivitet: Titanium can be reactive at elevated temperatures, requiring controlled atmosphere (argon or vacuum) during AM processing and certain post-processing steps like heat treatment.
- Lower Ductility (vs. 316L): While ELI grade improves ductility over standard Grade 5 Ti-6Al-4V, it is generally less ductile than 316L.
Material Selection Criteria for Surgical Cutting Guides:
Funktion | 316L rostfritt stål | Ti-6Al-4V ELI (Grade 23) |
---|---|---|
Biokompatibilitet | Excellent (ASTM F138/F139) | Exceptional (ASTM F136) |
Motståndskraft mot korrosion | Hög | Överlägsen |
Täthet | ~8,0 g/cm³ | ~4.4 g/cm³ |
Styrka | Bra | High (Similar/Higher than 316L) |
Stiffness (Modulus) | High (~193 GPa) | Moderate (~114 GPa) |
Duktilitet | Hög | Måttlig |
Kostnad | Lägre | Högre |
MRI Compatibility | Fair (Artifacts possible) | Good (Fewer artifacts) |
Primary Use Case | Cost-sensitive guides, single-use devices | Weight-sensitive guides, high-performance tools |
Export to Sheets
The Role of Powder Quality:
Regardless of the alloy chosen, the quality of the medical grade metal powders is critical for successful additive manufacturing. Powder characteristics directly impact the final part’s density, mechanical properties, and surface finish. Key powder attributes include:
- Sfäriskhet: Highly spherical powders ensure good flowability (essential for uniform powder bed layering) and high packing density, leading to denser final parts with fewer voids.
- Fördelning av partikelstorlek (PSD): A controlled PSD optimized for the specific AM process (e.g., SLM, SEBM) is crucial for process stability and part resolution.
- Renhet: Low levels of impurities and contaminants (especially oxygen in titanium) are vital for achieving desired mechanical properties and biocompatibility.
- Flytbarhet: Ensures consistent powder delivery and spreading across the build platform.
Ledande metal powder suppliers like Met3dp leverage advanced manufacturing techniques such as Vacuum Induction Melting Gas Atomization (VIGA) and Plasma Rotating Electrode Process (PREP). These methods, coupled with rigorous quality control, produce metallpulver av hög kvalitet with high sphericity, controlled PSD, low oxygen content, and excellent flowability, specifically optimized for AM processes like the Selective Electron Beam Melting (SEBM) systems offered by Met3dp. This ensures that the 316L and Ti-6Al-4V ELI powders used meet the stringent requirements for manufacturing reliable and safe medicintekniska produkter. Choosing a partner like Met3dp provides confidence in both the printing process and the fundamental quality of the Met3dp metal powders used to create these critical surgical instruments.
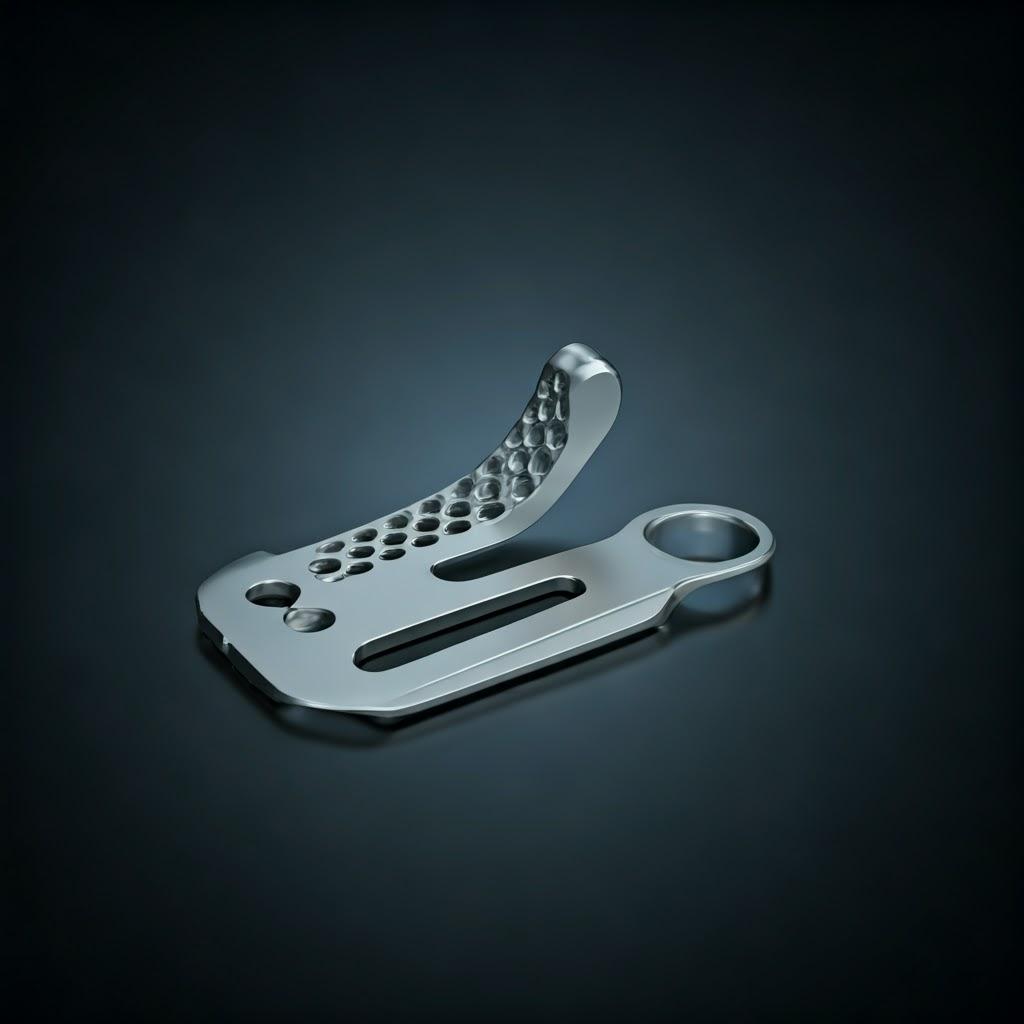
Design for Additive Manufacturing (DfAM): Optimizing Cutting Guides for Production
Simply replicating a design intended for machining using additive manufacturing often fails to leverage the full potential of AM and can even introduce production challenges. Design för additiv tillverkning (DfAM) is a crucial methodology that involves tailoring the part geometry specifically for the chosen AM process (like SLM or SEBM) and material (316L or Ti-6Al-4V ELI). Applying DfAM principles to surgical cutting guides ensures manufacturability, optimizes performance, reduces post-processing efforts, and can lower costs. Engineers and designers should consider the following key DfAM medical devices aspects:
1. Patient-Specific Conforming Surfaces:
- Leverage Scan Data: Utilize the high resolution of patient CT/MRI data to create guide surfaces that intimately conform to the bone anatomy. This ensures stable and accurate placement during surgery.
- Surface Offsetting: Account for cartilage thickness or soft tissue layers if the guide sits partially on such surfaces, ensuring the cutting/drilling features are accurately positioned relative to the underlying bone.
- Contact Area Optimization: Design sufficient, well-distributed contact points or surface areas to guarantee stability without making the guide unnecessarily bulky or difficult to place.
2. Support Structure Strategy & Minimization:
- Self-Supporting Angles: Design features with overhang angles greater than typically 45 degrees (relative to the build plate) to minimize the need for sacrificial support structures. This reduces material waste, printing time, and post-processing effort (support removal).
- Bygg orientering: Consider the optimal build orientation early in the design phase. Orienting critical features (like cutting slots or drill holes) vertically or self-supporting can improve accuracy and surface finish in those areas and simplify support removal.
- Support Design for Removal: When supports are unavoidable (e.g., for low angles, bridges, or large horizontal surfaces), design them strategically for easier removal. This includes using minimal contact points, perforation points, or designing specific access channels for removal tools, especially crucial for internal guide features.
3. Wall Thickness and Feature Size:
- Minimum Wall Thickness: Adhere to minimum printable wall thicknesses recommended for the chosen process (SLM/SEBM) and material to ensure structural integrity and prevent print failures. This typically ranges from 0.4mm to 1.0mm, depending on the specific printer and parameters.
- Feature Resolution: Understand the minimum feature size (e.g., pins, holes, slot widths) that can be accurately resolved by the printing process. Fine, delicate features may require careful design and orientation or might be better created via post-machining if ultra-high precision is needed.
- Uniform Thickness: Avoid abrupt changes in cross-sectional area where possible, as this can lead to thermal stresses and potential distortion during printing.
4. Designing for Sterilization and Cleaning:
- Eliminate Inaccessible Voids: Avoid creating internal cavities or channels that cannot be easily accessed for cleaning, powder removal, and sterilant penetration. If internal channels are necessary (e.g., for irrigation), ensure clear entry and exit points and smooth internal surfaces.
- Smooth Surfaces & Radii: Incorporate fillets and radii instead of sharp internal corners where possible. This aids in cleaning, reduces stress concentrations, and can improve fatigue life if the guide is intended for multiple uses (though many are single-use).
- Material Choice: Ensure the chosen material (316L or Ti-6Al-4V ELI) and the final surface finish are compatible with standard hospital sterilization methods (e.g., autoclaving).
5. Incorporating Functionality & Identification:
- Integrated Features: Combine multiple functions if possible. For instance, integrate drill sleeves directly into the cutting guide structure rather than having separate components.
- Text/Labeling: Embed identification markings (patient ID, surgical site indicators like ‘L’/’R’, part numbers, surgeon name) directly onto the guide surface. Use recessed (engraved) text rather than raised (embossed) text for easier cleaning and durability. Ensure font size and depth are suitable for AM resolution.
- Reference Features: Include clear anatomical landmarks or reference points on the guide to aid the surgeon in confirming correct placement.
6. Lightweighting with Lattice Structures:
- Selective Stiffness: For larger guides, internal lattice structures or topology optimization can significantly reduce weight and material usage while maintaining the necessary stiffness and rigidity in critical areas (like around cutting slots).
- Powder Removal: Ensure lattice designs allow for easy removal of unfused powder during post-processing. Open-cell structures are generally preferred over closed-cell designs unless specific mechanical properties are required.
Collaborating with AM Experts:
Successfully optimizing surgical guide design often requires close collaboration between the surgical planning team and AM specialists. Experienced leverantörer av 3D-utskriftstjänster av metall like Met3dp can offer invaluable DfAM consultation, leveraging their knowledge of process capabilities, material behavior, and post-processing requirements to help refine designs for optimal production and clinical performance. Their expertise ensures that the final CAD for additive manufacturing model is fully optimized for their specific printing systems and workflows.
Achieving Precision: Tolerance, Surface Finish, and Dimensional Accuracy
In surgery, precision is non-negotiable. Surgical cutting guides must accurately transfer the pre-operative plan to the patient’s anatomy, requiring tight control over dimensionell noggrannhet, appropriate ytfinish, and achievable toleranser. Understanding the capabilities and limitations of metal additive manufacturing in these areas is critical for engineers specifying these devices and for surgeons relying on them.
1. Dimensional Accuracy and Tolerance:
- General Tolerances: Metal powder bed fusion processes (SLM and SEBM) typically achieve dimensional accuracy in the range of ±0.1mm to ±0.2mm or ±0.1% to ±0.2% of the nominal dimension, whichever is larger. However, this can vary significantly based on several factors:
- Machine Calibration: Regular calibration and maintenance of the AM system (e.g., laser/electron beam positioning, energy density) are crucial. Met3dp’s commitment to industry-leading print volume, accuracy and reliability ensures their SEBM systems are maintained for optimal performance.
- Part Size and Geometry: Larger parts or those with complex geometries and varying thermal masses may exhibit greater deviation due to thermal stresses and potential warping.
- Bygg orientering: The orientation of a feature relative to the build layers affects its accuracy. Vertical walls are typically more accurate than sloped or curved surfaces.
- Material: Different materials (e.g., 316L vs. Ti-6Al-4V ELI) have different shrinkage rates and thermal properties, influencing final dimensions.
- Efterbearbetning: Stress relief heat treatments can cause minor dimensional changes, while machining can achieve much tighter tolerances on specific features.
- Critical Feature Tolerancing: For critical features like cutting slots or drill guide holes, tighter tolerances (e.g., ±0.05mm or better) may be required. These can often be achieved through:
- Processoptimering: Fine-tuning print parameters for specific features.
- Post-Machining: Incorporating a secondary CNC machining step to finish critical dimensions after printing. This adds cost but guarantees high precision where needed.
- Kvalitetskontroll: Rigorös quality control for medical devices is essential. This includes dimensional inspection using calibrated tools like CMMs (Coordinate Measuring Machines), 3D scanners, or calipers to verify conformance to specifications.
2. Surface Finish (Roughness):
- As-Built Surface Roughness: Parts produced by powder bed fusion typically have a rougher surface finish compared to machined parts. The as-built surface roughness (Ra) often ranges from 5 µm to 20 µm, influenced by:
- Powder Particle Size: Finer powders generally lead to smoother surfaces but can pose challenges with flowability. Met3dp’s advanced powder making system using gas atomization produces spherical powders with controlled PSD, optimizing for both print quality and surface finish.
- Skiktets tjocklek: Thinner layers result in smoother up-facing and angled surfaces.
- Energy Input: Laser/electron beam parameters affect melt pool stability and surface texture.
- Surface Orientation: Down-facing surfaces (supported areas) tend to be rougher than vertical or up-facing surfaces due to support contact points.
- Importance for Surgical Guides:
- Cleaning & Sterilization: Rough surfaces can be harder to clean thoroughly and may potentially harbor bioburden. Smoother surfaces facilitate effective sterilization.
- Tissue Contact: While guides have temporary contact, excessively rough surfaces could potentially cause minor tissue irritation or debris generation.
- Haptic Feel: Surgeons may prefer a smoother finish for handling.
- Förbättra ytfinishen: Post-processing is typically required to achieve the desired surface finish for surgical tools. Common methods include bead blasting, tumbling, micro-machining, grinding, polishing, or electropolishing (especially effective for 316L). Target Ra values for surgical guides are often in the range of 0.8 µm to 3.2 µm, depending on the specific requirements and contact area.
Achievable Precision Summary:
Parameter | As-Built (Typical Range) | After Post-Processing (Typical) | Anteckningar |
---|---|---|---|
Dimensionell tolerans | ±0.1 to ±0.2 mm | ±0.05 mm (Machined Features) | Highly dependent on geometry, size, material, process control |
Ytjämnhet (Ra) | 5 µm to 20 µm | 0.8 µm to 3.2 µm (or lower) | Achieved via blasting, tumbling, polishing, electropolishing, machining |
Export to Sheets
Achieving the necessary precision requires a combination of optimized design (DfAM), tightly controlled AM processing using high-quality equipment and materials (like those provided by Met3dp), and appropriate post-processing steps tailored to the specific requirements of the surgical cutting guide. Clear specification and verification through robust quality management systems are essential for regulatory compliance and clinical success.
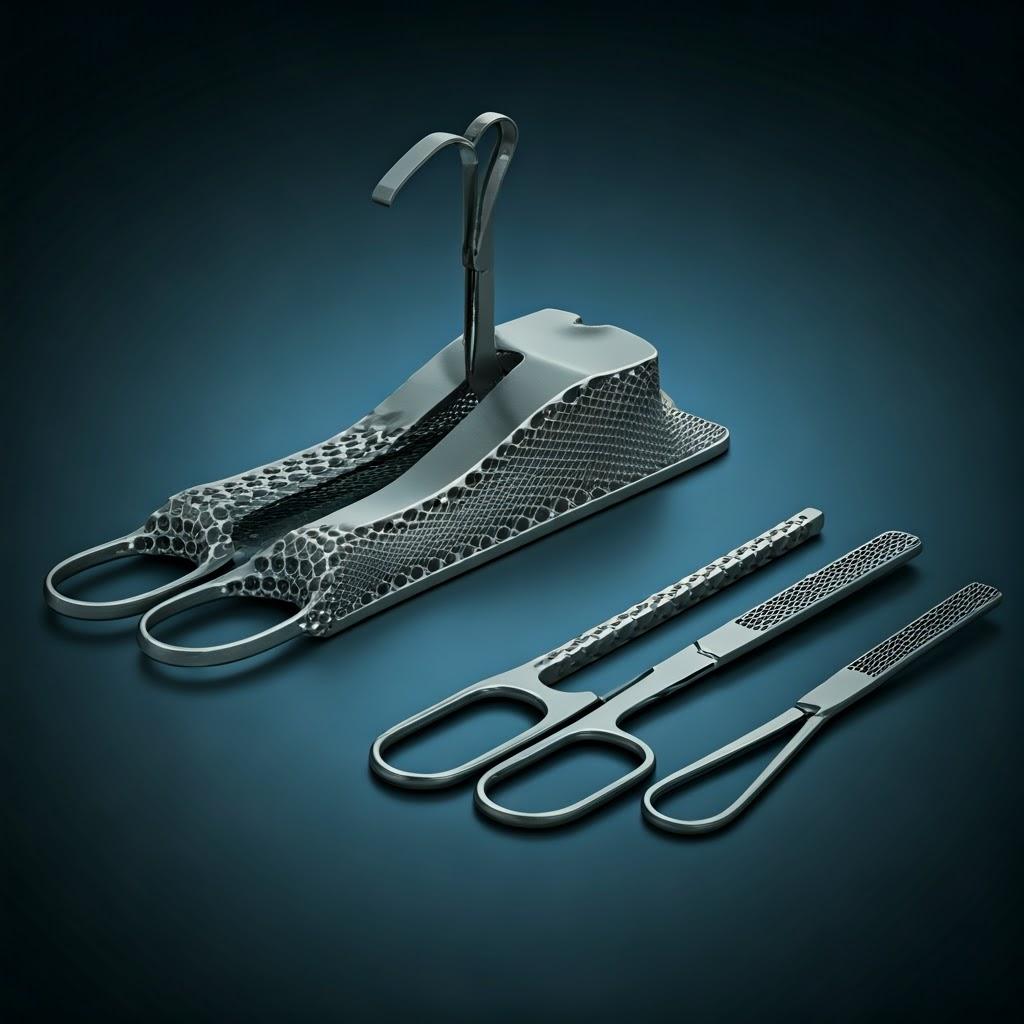
Essential Post-Processing Steps for Surgical Cutting Guides
Metal 3D printed parts, including surgical cutting guides made from 316L or Ti-6Al-4V ELI, rarely come off the printer ready for clinical use. A series of post-processing metal 3D prints steps are essential to transform the near-net shape part into a functional, safe, and sterile medical device. The specific sequence and requirements can vary based on the material, design complexity, and application, but typically involve the following stages:
1. Stress Relief / Heat Treatment:
- Syfte: The rapid heating and cooling cycles inherent in powder bed fusion can build up significant internal residual stresses within the printed part. These stresses can cause distortion (warping) after the part is removed from the build plate or during subsequent machining, and can negatively impact mechanical properties. Heat treatment relaxes these stresses.
- Förfarande:
- 316L rostfritt stål: While sometimes used as-printed, a stress relief anneal (e.g., at temperatures around 650°C – 900°C followed by controlled cooling) can be performed, especially if significant machining is planned or maximum dimensional stability is required. Solution annealing at higher temperatures (e.g., 1040°C – 1150°C) followed by rapid cooling can optimize corrosion resistance and ductility but may affect dimensional accuracy.
- Ti-6Al-4V ELI: Stress relief is almost always required. This is typically done in a vacuum or inert atmosphere furnace at temperatures ranging from 590°C to 840°C, followed by slow cooling. Specific cycles depend on the desired balance of properties. Hot Isostatic Pressing (HIP) may also be used – applying high pressure and temperature simultaneously to eliminate internal porosity and further improve mechanical properties, though it adds cost.
- Överväganden: Heat treatment must be performed before removing parts from the build plate if distortion is a major concern. It requires calibrated furnaces and controlled atmospheres (especially for titanium).
2. Part Removal from Build Plate:
- Metoder: Parts are typically removed from the build plate using wire EDM (Electrical Discharge Machining) or a band saw. Care must be taken not to damage the parts during this process.
3. Support Structure Removal:
- Syfte: Sacrificial support structures, needed to anchor the part and support overhanging features during printing, must be removed.
- Metoder: This is often a manual process using pliers, cutters, grinders, or specialized tools. For intricate or internal supports, methods like electrochemical machining or abrasive flow machining might be employed, although manual dexterity is most common.
- Utmaningar: Support removal can be labor-intensive and time-consuming, especially for complex geometries. Poorly designed supports can be difficult to access or may damage the part surface upon removal. DfAM plays a crucial role in minimizing and optimizing supports.
4. Machining (If Required):
- Syfte: To achieve tighter tolerances, specific surface finishes, or features that cannot be accurately produced by AM alone (e.g., very fine threads, sharp edges, critical interface dimensions).
- Förfarande: Standard CNC finishing additive parts techniques (milling, drilling, turning, grinding) can be used on 3D printed metal parts. Fixturing the complex geometry of a patient-specific guide might require custom solutions.
- Överväganden: Adds cost and lead time. Requires careful planning to integrate with the AM workflow.
5. Surface Finishing:
- Syfte: To remove support witness marks, improve surface smoothness for cleaning and handling, and achieve the desired aesthetic or functional surface texture.
- Metoder:
- Abrasive Blasting: (Bead blasting, sand blasting) Used to create a uniform matte finish and remove loose particles. Different media (glass beads, aluminum oxide) produce different textures.
- Tumbling/Vibratory Finishing: Parts are placed in a machine with media that rubs against the surfaces, smoothing edges and surfaces. Effective for batch processing but less controlled for specific areas.
- Grinding/Polishing: Manual or automated processes using abrasive belts, wheels, or compounds to achieve smoother, reflective surfaces. Required for achieving very low Ra values.
- Electropolishing: An electrochemical process that removes a microscopic layer of material, resulting in a very smooth, clean, and passive surface. Particularly effective for 316L stainless steel, enhancing its corrosion resistance.
- Passivation (for 316L): A chemical treatment (typically using nitric or citric acid) specifically for stainless steels to remove free iron from the surface and enhance the passive chromium oxide layer, maximizing passivation stainless steel corrosion resistance. Often performed after machining or polishing.
6. Cleaning and Inspection:
- Cleaning: Thorough cleaning is critical to remove residual powder, machining fluids, polishing compounds, and any other contaminants before sterilization. Multi-stage processes involving ultrasonic baths, detergents, and rinsing with purified water are common.
- Inspection: Includes dimensional verification (CMM, scanning), visual inspection for defects (cracks, porosity), surface finish measurement, and potentially NDT (Non-Destructive Testing) like CT scanning for internal integrity if required by risk assessment.
7. Marking/Labeling (If not done in AM):
- Laser marking is often used to add permanent identification marks if not included during the build process.
8. Preparation for Sterilization:
- The final cleaned and inspected guide must be packaged appropriately (e.g., in sterilization pouches) before undergoing validated sterilization, typically via steam autoclaving.
The complexity and rigor of these post-processing steps highlight the importance of choosing a metal AM partner like Met3dp, who not only possesses advanced printing technology but also has established, validated workflows and expertise in handling the necessary finishing, cleaning, and inspection processes required for critical medical device components. Their comprehensive solutions ensure that the cutting guides meet all specifications and are ready for clinical use.
Navigating Challenges: Common Issues and Mitigation Strategies
While metal additive manufacturing offers significant advantages for producing surgical cutting guides, it’s not without its challenges. Understanding potential metal 3D printing defects and implementing effective mitigation strategies is crucial for ensuring consistent quality, reliability, and safety. Procurement managers and engineers should be aware of these common issues when working with AM suppliers:
1. Warping and Distortion:
- Cause: Uneven heating and cooling during the layer-by-layer process generate internal stresses. When the part is released from the build plate, these stresses can cause it to warp or deviate from the intended geometry. Larger parts or those with significant variations in cross-section are more susceptible.
- Mitigation Strategies:
- Optimized Build Orientation: Orienting the part to minimize large flat surfaces parallel to the build plate and reduce thermal gradients.
- Effective Support Structures: Strategically placed supports act as heat sinks and anchor the part securely during the build.
- Process Parameter Control: Fine-tuning laser/electron beam power, scan speed, and hatching strategies to manage heat input. Met3dp’s focus on accuracy and reliability includes optimizing parameters for thermal management.
- Stress Relief Heat Treatment: Performing a heat treatment cycle before removing the part from the build plate is highly effective in relaxing residual stresses.
- Simulation: Using process simulation software to predict stress accumulation and optimize orientation and supports preemptively.
2. Support Removal Difficulties:
- Cause: Supports in hard-to-reach areas (internal channels, complex geometries) or overly dense support structures can be challenging and time-consuming to remove without damaging the part.
- Mitigation Strategies:
- DfAM: Designing for minimal support need (self-supporting angles, topology optimization).
- Smart Support Design: Using easily removable support types (e.g., conical points, perforated structures) and planning access routes.
- Process Selection: Some AM processes might offer easier support removal (e.g., binder jetting, though material properties differ). Powder bed fusion requires careful support planning.
- Skilled Technicians: Experienced technicians are essential for effective manual support removal.
3. Surface Finish Imperfections:
- Cause: The layer-wise nature of AM, partially melted powder particles adhering to surfaces (“powder spatter”), and support contact points inherently result in a rougher finish than machining. Down-facing surfaces are often particularly rough.
- Mitigation Strategies:
- Optimized Parameters: Fine-tuning energy input and gas flow (in SLM) can reduce spatter.
- Pulverkvalitet: Using highly spherical powders with controlled PSD, like those produced by Met3dp’s advanced powder making system, promotes smoother layers.
- Bygg orientering: Orienting critical surfaces upwards or vertically.
- Effective Post-Processing: Implementing appropriate surface finishing techniques (blasting, tumbling, polishing, electropolishing) as described previously.
4. Porosity:
- Cause: Small voids or pores within the printed material can result from incomplete fusion between layers (Lack of Fusion – LoF), gas becoming trapped in the melt pool (gas porosity), or keyholing (vapor depression instability at high energy densities). Porosity can compromise mechanical strength and fatigue life.
- Mitigation Strategies:
- Processparameteroptimering: Developing robust parameter sets (power, speed, layer thickness, hatch spacing) specific to the material and machine to ensure complete melting and stable melt pools.
- Powder Quality & Handling: Using high-quality, dry powder with good flowability minimizes gas pickup and ensures uniform layers.
- Controlled Atmosphere: Maintaining a high-purity inert gas environment (Argon in SLM) or vacuum (in SEBM) minimizes gas porosity. Met3dp’s SEBM printers operate under vacuum, contributing to high-density parts.
- Het isostatisk pressning (HIP): An effective post-processing step to close internal pores, especially critical for fatigue-sensitive applications (though potentially overkill for many single-use guides).
- Icke-förstörande testning (NDT): Using CT scanning or other methods to detect internal porosity as part of quality assurance AM medical.
5. Residual Stress Management:
- Cause: As mentioned under warping, thermal gradients create stress. Even if macroscopic warping is avoided, high residual stress can impact fatigue life or cause unexpected distortion during machining.
- Mitigation Strategies:
- Värmebehandling: The primary method for reducing residual stress.
- Build Strategy: Using optimized scan patterns (e.g., island scanning) can help distribute heat more evenly.
- Process Simulation: Predicting and managing stress build-up.
6. Powder Removal:
- Cause: Unfused powder can become trapped in intricate features, internal channels, or lattice structures. Complete removal is essential for biocompatibility and proper function.
- Mitigation Strategies:
- DfAM: Designing features with clear escape paths for powder (e.g., minimum hole sizes, accessible lattices).
- Tekniker för efterbearbetning: Utilizing compressed air, vibration, ultrasonic cleaning, and thorough flushing protocols.
- Inspection: Visually inspecting and potentially using methods like CT scanning to confirm powder removal from critical areas.
Successfully navigating these challenges requires deep expertise in materials science, process physics, DfAM, and rigorous process control. Partnering with an experienced metal 3D printing service provider medical like Met3dp, which has a proven track record, robust quality systems (potentially including ISO 13485 certified additive manufacturing), and a thorough understanding of these potential issues, is key to mitigating risks and ensuring the reliable supply of high-quality, safe surgical cutting guides. Their integrated approach, from högpresterande metallpulver to advanced printing and post-processing, provides a strong foundation for tackling these challenges.
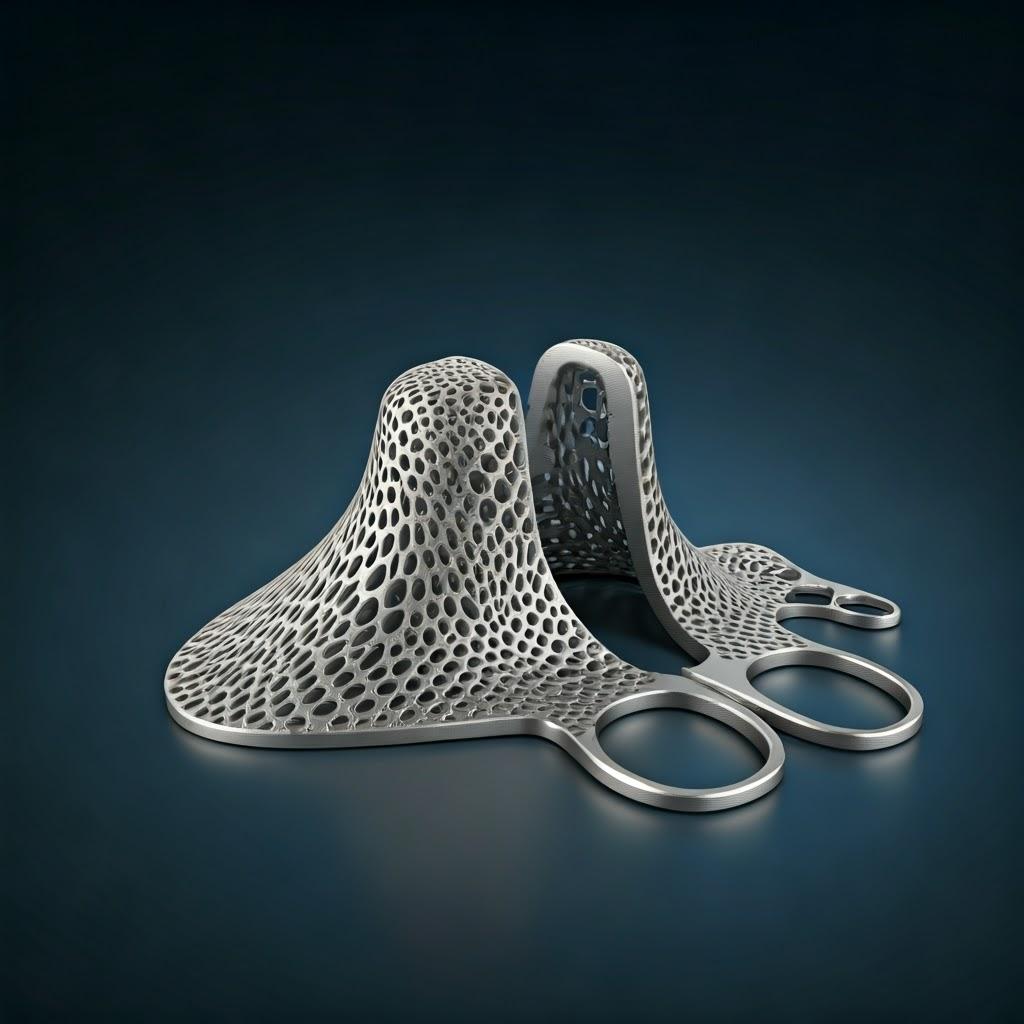
Supplier Selection: Choosing the Right Metal AM Partner for Medical Devices
The clinical success and regulatory compliance of 3D printed surgical cutting guides depend heavily on the capabilities and quality standards of the manufacturing partner. Selecting the right metal 3D printing service provider medical is a critical decision for medical device companies, hospitals, and surgical teams. Procurement managers and engineers should evaluate potential suppliers based on a comprehensive set of criteria:
1. Quality Management System (QMS) and Certifications:
- ISO 13485 Certification: This is the international standard for quality management systems for medical device manufacturers. ISO 13485 certified additive manufacturing demonstrates a supplier’s commitment to maintaining traceability, process control, risk management, and documentation required for medical device production. This should be considered a minimum requirement.
- FDA Registration (If applicable): If supplying to the US market, ensure the supplier understands and complies with relevant FDA regulations (e.g., Quality System Regulation – 21 CFR Part 820).
- Robust Internal QMS: Beyond certifications, assess the supplier’s internal quality procedures, including material acceptance, process monitoring, equipment calibration, non-conformance handling, and final inspection protocols.
2. Medical Device Expertise and Experience:
- Proven Track Record: Look for suppliers with demonstrable experience in manufacturing similar medical devices, particularly orthopedic or CMF instruments. Case studies, testimonials, and references can provide valuable insights.
- Understanding of Clinical Needs: The supplier should understand the critical nature of surgical guides and the importance of accuracy, biocompatibility, and timely delivery in a clinical setting.
- Regulatory Awareness: Familiarity with the regulatory pathways for patient-specific instruments in target markets is crucial.
3. Material Expertise and Traceability:
- Specialization in Medical Alloys: Ensure the supplier has extensive experience printing with the required materials (316L, Ti-6Al-4V ELI) and can provide evidence of optimal process parameters for achieving high density and desired mechanical properties.
- Powder Sourcing and Control: Verify the supplier sources high-quality, medical-grade metal powders from reputable sources or, ideally, produces them in-house with rigorous quality control. Full traceability of powder batches from raw material to final part is essential. Met3dp, for instance, leverages its own avancerat system för pulvertillverkning (Gas Atomization and PREP) to ensure the quality and consistency of its metallpulver av hög kvalitet.
- Material Certifications: The supplier must provide material certifications confirming compliance with relevant standards (e.g., ASTM F138/F139 for 316L, ASTM F136 for Ti-6Al-4V ELI).
4. Technological Capabilities:
- Appropriate AM Technology: Ensure the supplier utilizes suitable, industrial-grade metal AM systems (like SLM or SEBM) known for producing high-accuracy, high-density parts. Met3dp’s specialization in SEBM printers provides industry-leading print volume, accuracy and reliability.
- Process Validation: The supplier should have validated processes (IQ/OQ/PQ – Installation, Operational, and Performance Qualification) for their AM equipment and critical post-processing steps, ensuring consistency and repeatability.
- Comprehensive Post-Processing: Evaluate their in-house or tightly controlled external capabilities for all necessary post-processing steps: stress relief, support removal, CNC machining, surface finishing (polishing, blasting, electropolishing, passivation), cleaning, and inspection.
5. Design Support and Collaboration:
- DfAM Expertise: The ideal partner can offer Design for Additive Manufacturing support, collaborating with your design team to optimize guide designs for printability, functionality, and cost-effectiveness.
- Communication and Responsiveness: Clear communication channels, project management, and responsiveness are vital, especially when dealing with patient-specific cases requiring quick turnaround.
6. Capacity and Scalability:
- Produktionskapacitet: Ensure the supplier has sufficient machine capacity and resources to meet your volume requirements and lead time expectations, from single patient-specific guides to batch production.
- Skalbarhet: Can the supplier scale production up or down as your needs evolve?
Met3dp: A Trusted Partner for Medical AM
Met3dp exemplifies the qualities of a reliable medical device contract manufacturing partner in the additive space. With decades of collective expertise in metal additive manufacturing, Met3dp offers comprehensive solutions encompassing:
- Advanced SEBM Printers: Delivering precision and reliability for mission-critical medical parts.
- In-House High-Quality Powder Production: Ensuring material quality and traceability for alloys like Ti-6Al-4V ELI, 316L, CoCrMo, and even custom or developmental alloys like TiNi or TiTa.
- End-to-End Capabilities: From DfAM consultation through printing, post-processing, and quality assurance.
- Fokus på branschen: Experience serving demanding sectors including medical, aerospace, and automotive.
Av evaluating AM suppliers thoroughly against these criteria, you can establish a partnership that ensures the consistent delivery of safe, effective, and high-quality 3D printed surgical cutting guides.
Understanding Cost Drivers and Production Lead Times
Implementing 3D printed surgical cutting guides requires understanding the factors that influence their cost and the time required for production. While AM offers advantages for customization, careful planning is needed to manage budgets and surgical schedules effectively.
Key Cost Factors for Metal 3D Printed Guides:
- Part Volume and Complexity:
- Print Time: Larger or more complex parts require longer printing times, directly impacting machine utilization costs. Part volume is often the most significant cost driver.
- Bounding Box: The overall dimensions influence how many parts can fit on a single build plate, affecting per-part machine time costs in batch production.
- Material Type and Usage:
- Kostnad för pulver: Ti-6Al-4V ELI powder is significantly more expensive than 316L rostfritt stål pulver.
- Material Volume: The actual volume of material used in the part contributes to the cost. Design optimization (e.g., using lattice structures) can help reduce material consumption.
- Support Structure Volume: Material used for support structures adds to the cost and requires time/labor for removal. DfAM aims to minimize this.
- Krav på efterbearbetning:
- Support Removal Labor: Complex supports require more manual effort.
- Ytbehandling: The level of finish required (e.g., basic bead blast vs. extensive manual polishing or electropolishing) significantly impacts labor and processing costs.
- Maskinbearbetning: Any secondary CNC machining adds considerable cost due to programming, setup, and machine time.
- Heat Treatment/HIP: Adds furnace time and energy costs (HIP is particularly expensive).
- Quality Assurance and Inspection:
- The level of dimensional inspection (e.g., basic caliper checks vs. full CMM report), NDT (if required), and documentation complexity adds to the overhead.
- Design and Engineering Time:
- For patient-specific guides, the time spent by engineers or designers converting scan data into a printable guide design (segmentation, virtual planning, guide design) is a key cost component, often managed by specialized surgical planning services or integrated hospital teams.
- Order Volume (Economies of Scale):
- While AM is cost-effective for single custom parts, some economies of scale can be achieved in batch production (e.g., printing multiple identical or similar guides simultaneously) by optimizing build plate usage and streamlining post-processing workflows. Quoting metal AM services for bulk orders may reflect lower per-unit costs compared to single pieces.
Typical Production Lead Times:
Lead time refers to the duration from design freeze (final CAD model approval) to the shipment of the finished part. For patient-specific surgical guides, rapid turnaround is often critical.
- Design Phase: (Performed by surgical planning team/service) Can range from hours to days depending on case complexity and workflow efficiency.
- Manufacturing Phase:
- Build Preparation: Few hours (queuing, slicing, machine setup).
- Tryckning: Typically 8 to 36+ hours, depending on part size, complexity, and number of parts on the build plate.
- Cool Down & Depowdering: Few hours.
- Heat Treatment (if needed): 8 to 24 hours (including furnace cycles).
- Part Removal & Support Removal: Few hours to a full day, depending on complexity.
- Machining (if needed): Can add 1-3 days depending on complexity and scheduling.
- Surface Finishing & Cleaning: Few hours to multiple days depending on requirements.
- Kvalitetsinspektion: Few hours to a day.
- Overall Manufacturing Lead Time: For a typical patient-specific guide, the manufacturing lead time (post-design freeze) often ranges from 3 to 10 business days, heavily dependent on the specific processes required (especially machining and complex finishing) and the supplier’s capacity and scheduling.
Expedited services are often available at a premium cost. Clear communication with the chosen metal AM supplier regarding lead time expectations is crucial for effective surgical planning. Efficient suppliers like Met3dp strive to optimize their integrated workflows to minimize additive manufacturing lead times while maintaining rigorous quality standards.
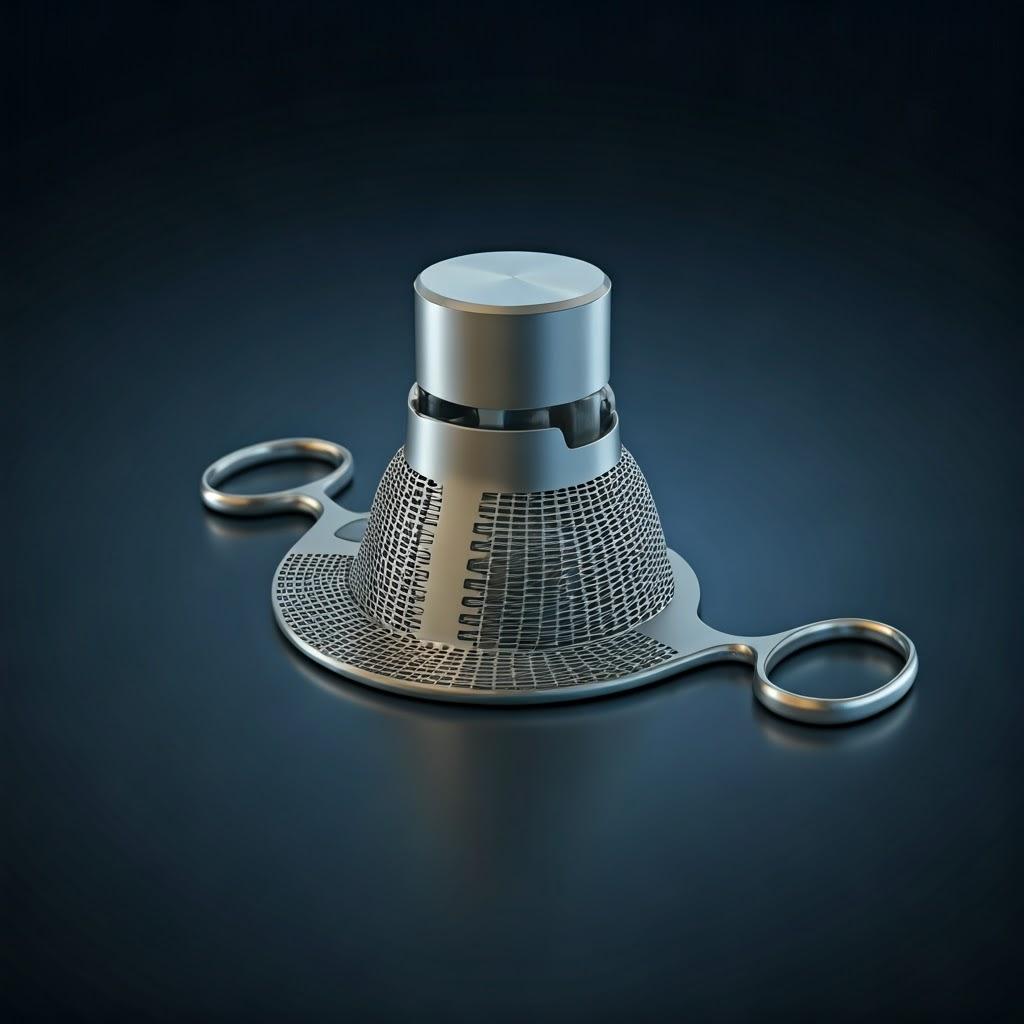
Frequently Asked Questions (FAQ) about 3D Printed Surgical Cutting Guides
Here are answers to some common questions regarding metal 3D printed surgical cutting guides:
1. Are 3D printed metal surgical guides sterilizable?
- Yes. Guides printed from medical-grade materials like 316L stainless steel and Ti-6Al-4V ELI are compatible with standard hospital sterilization methods, primarily steam autoclaving. The specific cycle parameters should be validated. Proper cleaning before sterilization is absolutely critical, and the design (DfAM) should ensure no features hinder sterilant penetration. Post-processing steps like electropolishing (for 316L) can further enhance cleanability and corrosion resistance during sterilization cycles.
2. How is the biocompatibility of these guides ensured?
- Biocompatibility is ensured through several key factors:
- Materialval: Using certified medical-grade metal powders (e.g., meeting ASTM F138/F139 for 316L or ASTM F136 for Ti-6Al-4V ELI) with known biocompatibility profiles.
- Processtyrning: Utilizing validated printing processes that produce dense, chemically stable parts without harmful residues.
- Efterbearbetning: Implementing validated cleaning procedures to thoroughly remove any residual powder, support material, or processing fluids. Passivation (for 316L) further enhances the biocompatible passive surface layer.
- Supplier QMS: Partnering with an ISO 13485 certified supplier ensures adherence to procedures designed to maintain biocompatibility throughout the manufacturing process.
3. What level of accuracy can be expected from a 3D printed cutting guide?
- As discussed in the precision section, typical metal 3D printing tolerances are in the range of ±0.1 mm to ±0.2 mm for general dimensions. Critical features like cutting slots or drill holes can often achieve higher accuracy directly through printing process optimization. If tighter tolerances (e.g., ±0.05 mm or less) are required for specific features, post-process CNC machining can be incorporated to achieve that precision. Verification through 3D scanning or CMM inspection confirms the final accuracy.
4. Can these surgical cutting guides be reused?
- Most patient-specific surgical cutting guides are designed and intended for single surgical episode use. While the materials themselves (316L, Ti-6Al-4V ELI) are durable, the primary reasons for single-use designation include:
- Cleaning Validation: Validating cleaning protocols to ensure complete removal of biological debris from complex geometries after use can be challenging and costly.
- Sterilization: Repeated sterilization cycles can potentially affect the material or surface over time, although these materials are generally robust.
- Patient Specificity: The guide is matched to one specific patient’s anatomy.
- Kostnadseffektivitet: The cost of rigorous cleaning, inspection, and re-sterilization often outweighs the cost of printing a new single-use guide.
5. What information is needed to order a patient-specific surgical cutting guide?
- Typically, the process starts with anonymized patient medical imaging data, usually from a CT or MRI scan, in DICOM format. This data is used by a surgical planning team or specialized service provider to create a virtual surgical plan. Based on this plan, the guide is designed in CAD software. The necessary inputs for the metal AM service provider are usually:
- The final 3D CAD model of the guide (e.g., in .STL or .STEP format).
- Material specification (316L or Ti-6Al-4V ELI).
- Required tolerances for critical features.
- Desired surface finish.
- Any specific marking/labeling requirements.
- Quantity and required delivery date.
Conclusion: The Future of Surgery Enhanced by Metal 3D Printed Guides
Metal additive manufacturing has irrevocably advanced the field of surgical instrumentation, and 3D printed cutting guides stand out as a prime example of its transformative power. By enabling the creation of patient-specific surgical guides from robust, biocompatible materials like 316L rostfritt stål och Ti-6Al-4V ELI, this technology directly contributes to enhanced surgical accuracy, improved efficiency in the operating room, and ultimately, better patient outcomes.
The ability to translate a virtual surgical plan into a physical template that perfectly matches an individual’s anatomy minimizes guesswork and allows surgeons to perform complex procedures with greater confidence and predictability. From intricate osteotomies in orthopedic and maxillofacial surgery to precise implant placement, these guides streamline workflows and support minimally invasive approaches.
Key takeaways include:
- Anpassning: AM excels at producing complex, patient-matched geometries impossible or impractical with traditional methods.
- Precision: When combined with appropriate post-processing, metal AM delivers the accuracy required for demanding surgical applications.
- Materialets prestanda: Medical-grade alloys like 316L and Ti-6Al-4V ELI offer proven biocompatibility and mechanical strength.
- Partnership is Key: Realizing the full potential requires collaborating with an expert metal AM partner possessing ISO 13485 certification, deep material and process knowledge, comprehensive post-processing capabilities, and a robust quality management system.
As AM technologies continue to mature, offering faster printing speeds, higher resolution, and an expanding portfolio of qualified materials, the scope for 3D printed surgical instruments will only broaden. Companies like Met3dp are at the vanguard of this evolution, providing not just state-of-the-art SEBM skrivare och metallpulver av hög kvalitet, but also the crucial application development expertise needed to turn innovative concepts into clinical reality.
Investing in metal 3D printed surgical cutting guides is an investment in the future of precision surgery. By leveraging the unique capabilities of additive manufacturing and partnering with knowledgeable suppliers, medical device companies and healthcare providers can continue to advance patient care and redefine surgical possibilities.
Explore how Met3dp’s cutting-edge systems and materials can power your organization’s additive manufacturing goals for medical devices. Visit met3dp.com to learn more about our comprehensive solutions.
Dela på
Facebook
Twitter
LinkedIn
WhatsApp
E-post
MET3DP Technology Co, LTD är en ledande leverantör av lösningar för additiv tillverkning med huvudkontor i Qingdao, Kina. Vårt företag är specialiserat på 3D-utskriftsutrustning och högpresterande metallpulver för industriella tillämpningar.
Förfrågan för att få bästa pris och anpassad lösning för ditt företag!
Relaterade artiklar
Om Met3DP
Senaste uppdateringen
Vår produkt
KONTAKTA OSS
Har du några frågor? Skicka oss meddelande nu! Vi kommer att betjäna din begäran med ett helt team efter att ha fått ditt meddelande.
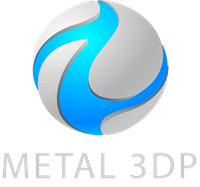
Metallpulver för 3D-printing och additiv tillverkning
FÖRETAG
PRODUKT
cONTACT INFO
- Qingdao City, Shandong, Kina
- [email protected]
- [email protected]
- +86 19116340731