Custom Cooling Channels 3D Printed in Copper Alloys
Innehållsförteckning
Introduction: Revolutionizing Thermal Management with 3D Printed Copper Cooling Channels
In the relentless pursuit of performance, efficiency, and miniaturization across industries like aerospace, automotive, medical, and high-power electronics, effective thermal management has transitioned from a design consideration to a critical enabling technology. Components are operating under increasingly demanding conditions – higher power densities, faster speeds, and more compact environments – generating significant heat that must be dissipated efficiently to ensure reliability, longevity, and optimal performance. Failure to manage this thermal load can lead to catastrophic failures, reduced lifecycles, and compromised functionality. This is where high-flow cooling channels play a pivotal role, acting as the intricate vascular systems within components, carrying away damaging heat.
Traditionally, manufacturing these vital cooling channels involved subtractive methods like drilling and milling, or joining techniques such as brazing and casting. While effective for simpler designs, these methods inherently limit the geometric complexity achievable. Straight lines, simple curves, and bulky junctions are often the norm, restricting the channel’s ability to conform perfectly to the heat source or navigate complex internal spaces. This often results in suboptimal cooling efficiency, larger component sizes, and longer development cycles, creating bottlenecks for innovation. Procurement managers often grapple with sourcing these complex, multi-part assemblies, managing multiple suppliers, and dealing with the inherent risks of joint failures in brazed components under high pressure or thermal stress.
Enter the world of metalltillsatstillverkning (AM), more commonly known as metal 3D-utskrifter. This disruptive technology is fundamentally reshaping how engineers approach thermal management challenges. By building components layer by layer directly from digital designs using high-performance metal powders, AM unlocks unprecedented design freedom. Specifically, the application of copper 3D printing för att skapa custom cooling components has emerged as a game-changer. Copper and its alloys, renowned for their exceptional thermal conductivity, can now be formed into highly complex, internal cooling channel networks that were previously impossible to manufacture.
Imagine cooling channels that precisely follow the contours of a heat-generating surface (conformal cooling), intricate lattice structures that maximize surface area for heat exchange within a minimal volume, or single-piece components with integrated, leak-proof channels, eliminating failure-prone joints. This is the reality enabled by metal AM. The benefits extend beyond just performance; additive manufacturing facilitates rapid prototyping, reduces lead times for complex parts, enables part consolidation, and opens avenues for lightweighting – advantages that resonate strongly with both design engineers seeking performance breakthroughs and purchasing departments looking for efficient, reliable metal AM suppliers.
Met3dp stands at forefront of this technological shift. As a leading provider of additive manufacturing solutions, specializing in both advanced 3D-utskriftssystem av metall and high-performance metal powders, Met3dp empowers industries to harness the full potential of AM for thermal management. Our expertise in processing challenging materials like copper alloys, combined with our commitment to quality and precision, makes us a trusted partner for businesses seeking to develop and procure next-generation high-flow cooling channels and other demanding thermal solutions. This article delves into the world of 3D printed copper cooling channels, exploring their applications, the advantages over traditional methods, the critical materials involved, and key considerations for design, manufacturing, and procurement.
Applications: Where are High-Flow Copper Cooling Channels Making an Impact?
The ability to create highly efficient, geometrically complex cooling channels using copper alloys via 3D printing has unlocked performance gains and solved persistent thermal challenges across a diverse range of demanding industries. These custom thermal management solutions are not just theoretical concepts; they are actively being deployed where conventional cooling methods fall short.
Key Industries Benefiting from 3D Printed Copper Cooling Channels:
- Flyg- och rymdindustrin samt försvarsindustrin:
- Komponenter till raketmotorer: Combustion chambers and nozzle throats in liquid rocket engines experience extreme temperatures and heat fluxes. 3D printed copper alloy liners with integrated, conformally mapped cooling channels provide highly efficient regenerative cooling, allowing for higher performance, longer firing durations, and reduced engine weight. The ability to print complex internal features optimizes coolant flow and heat absorption precisely where needed. Suppliers focus on reliability and material properties under extreme conditions.
- Hypersonic Vehicles: Managing the intense aerodynamic heating on leading edges and engine components is critical. Custom 3D printed thermal protection systems with embedded copper cooling channels offer a potential solution for active cooling in these demanding environments.
- Avionics and Directed Energy Systems: High-power density electronics generate significant heat. Compact, lightweight heat sinks and cold plates with optimized internal channel geometries, 3D printed in copper, ensure reliable operation in space-constrained airborne platforms.
- Automotive (Performance and Electric Vehicles):
- Electric Vehicle (EV) Components: Battery packs, power electronics (inverters, converters), and electric motors are major heat sources in EVs. Efficient thermal management is crucial for battery life, charging speed, and overall vehicle performance. 3D printed copper cooling plates and busbars with integrated channels offer superior, localized cooling compared to traditional extruded or stamped solutions, enabling higher power densities and faster charging. Procurement managers in the EV supply chain seek scalable solutions for automotive EV cooling.
- High-Performance Engines: In motorsport and performance vehicles, components like cylinder heads, pistons, and turbochargers benefit from enhanced cooling. 3D printed copper alloy inserts with conformal cooling channels can extract heat more effectively from critical areas, allowing for higher combustion pressures, improved engine efficiency, and greater reliability under race conditions.
- Värmeväxlare: Compact, high-efficiency heat exchangers for various automotive fluid systems can be designed and printed with complex internal structures (like triply periodic minimal surfaces – TPMS) that maximize heat transfer surface area within a given volume.
- Industrial Manufacturing and Tooling:
- Plastic Injection Molds & Die Casting: Conformal cooling channels, precisely following the shape of the mold cavity or core, are a well-established application for metal AM. Using copper alloys (particularly CuCrZr for its higher strength) instead of traditional tool steels significantly reduces cooling times, leading to shorter injection cycles, improved part quality (less warpage), and increased productivity. Tooling suppliers offer custom cooling design services based on AM.
- High-Power Electronics Cooling: Industrial power systems, welding equipment, high-frequency induction heating systems, and large data centers rely on robust cooling. 3D printed copper heat sinks, liquid cold plates, and busbars with optimized high-flow channels provide efficient thermal management solutions capable of handling very high heat loads.
- Laser Systems: Components within industrial laser systems, such as laser diodes and optical mounts, require precise temperature control. Custom copper cooling elements manufactured via AM ensure stability and longevity.
- Medicintekniska produkter:
- Imaging Equipment: Components in high-power X-ray tubes or MRI gradient coils generate substantial heat. 3D printed copper cooling elements provide efficient, compact thermal management solutions necessary for reliable and high-resolution imaging. Material purity and biocompatibility (if applicable) are key considerations for medical device cooling suppliers.
- Surgical Tools: Certain powered surgical instruments or laser probes may require integrated cooling. AM allows for the creation of complex, miniaturized cooling channels within these tools.
- Forskning och utveckling:
- Experimental Physics: Particle accelerators, fusion reactors, and other large-scale scientific instruments often require custom components capable of handling extreme heat fluxes. 3D printed copper alloys provide solutions for targets, collimators, and other thermally loaded elements.
- Prototyping: AM enables rapid iteration of complex thermal management designs, allowing researchers and engineers to test and validate novel cooling concepts quickly.
Cross-Industry Advantages Driving Adoption:
Feature Enabled by AM Copper Channels | Benefit Across Industries | B2B Focus Area |
---|---|---|
Conformal Cooling Paths | More uniform temperature control, reduced hot spots | Improved part quality, higher yields |
Complex Internal Geometries | Increased surface area, turbulent flow, higher heat transfer | Enhanced performance, efficiency gains |
Konsolidering av delar | Reduced assembly steps, elimination of joints/welds/brazes | Improved reliability, lower assembly cost |
Thin Walls & Lightweighting | Reduced component mass, material savings | Aerospace efficiency, automotive range |
Rapid Design Iteration | Faster prototyping and validation | Reduced time-to-market, R&D agility |
Anpassning | Solutions tailored to specific thermal challenges | Bespoke engineering, niche markets |
Export to Sheets
The breadth of these applications underscores the transformative potential of copper 3D printing för thermal management solutions. It enables engineers to overcome previous manufacturing constraints and design for optimal thermal performance, driving innovation and efficiency across multiple high-value sectors. Businesses looking to source these components require partners with deep expertise in both materials science and additive manufacturing processes.
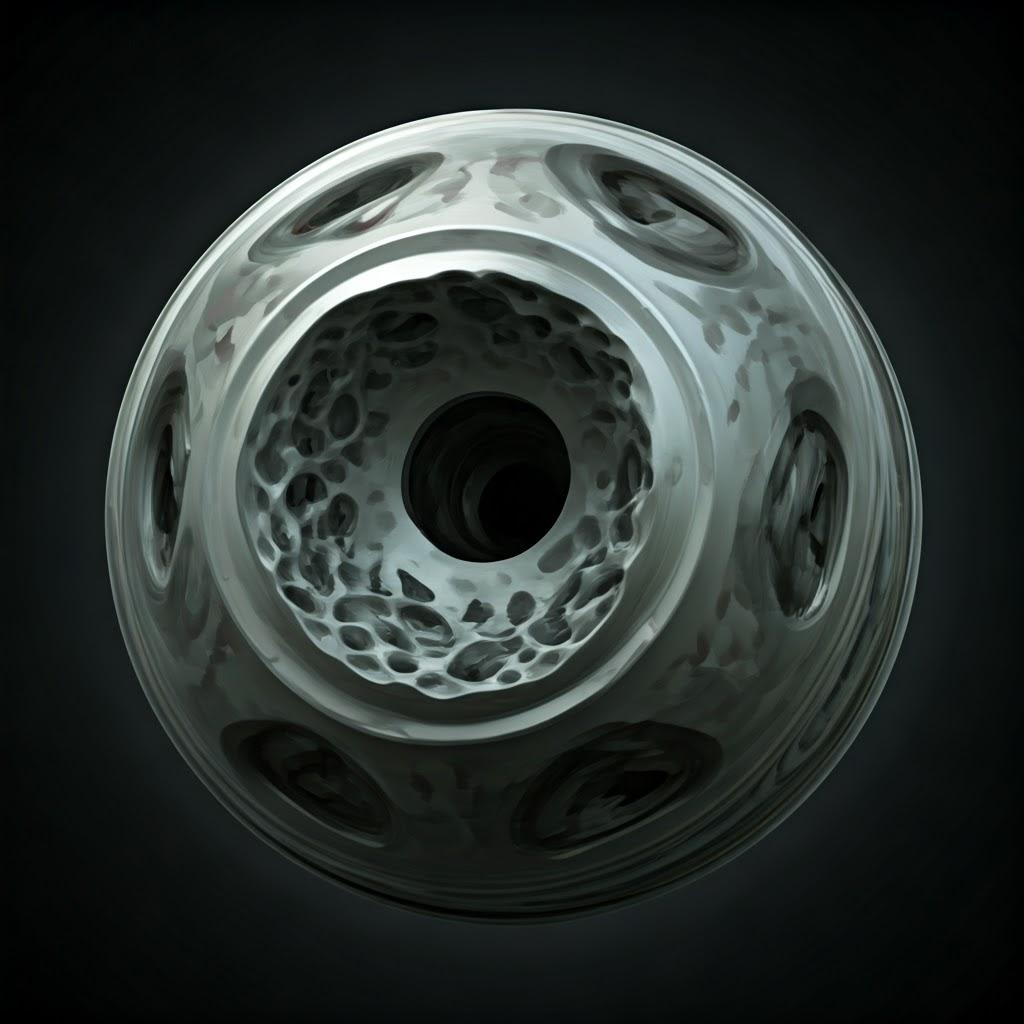
Why Choose Metal 3D Printing for Custom Cooling Channels?
While traditional manufacturing methods have served industries for decades, they present significant limitations when fabricating intricate, high-performance cooling channels, especially those requiring complex internal geometries or conformal paths. Metal additive manufacturing, particularly powder bed fusion techniques like Selective Laser Melting (SLM) or Direct Metal Laser Sintering (DMLS), offers compelling advantages that directly address these shortcomings, making it the preferred choice for cutting-edge thermal management applications.
Let’s compare AM with traditional methods for producing cooling channels:
Traditional Methods & Their Limitations:
- Machining (Drilling/Milling):
- Process: Material removal using drills and end mills.
- Cooling Channels: Primarily limited to straight lines or simple curves accessible from the exterior. Complex internal networks require multiple parts to be machined and then joined. Gun drilling can achieve deep straight holes.
- Begränsningar: Geometric constraints (line-of-sight access), difficulty creating conformal channels, complex internal features are impossible or require multi-part assemblies, potential for stress concentrations at sharp corners.
- Casting:
- Process: Pouring molten metal into a mold containing cores to form internal passages.
- Cooling Channels: Can create more complex shapes than machining, but limited by core removal, draft angles, minimum wall thickness, and potential for porosity.
- Begränsningar: Lower dimensional accuracy and surface finish compared to machining or AM, difficulty achieving very thin walls or intricate details, challenges with removing complex core structures, potential for casting defects (porosity, inclusions).
- Brazing/Welding:
- Process: Joining multiple machined or formed parts together to create an assembly with internal channels.
- Cooling Channels: Allows assembly of complex channel networks from simpler individual components.
- Begränsningar: Joints introduce potential weak points (leakage, thermal stress failure), limitations on joint proximity and complexity, additional manufacturing steps increase lead time and cost, potential for filler material to obstruct channels or affect thermal properties, managing distortion during joining.
Advantages of Metal Additive Manufacturing (AM) for Cooling Channels:
Metal AM overcomes many of these limitations by building the part layer-by-layer, offering unparalleled design freedom and functional integration:
- Unmatched Geometric Complexity:
- Conformal Channels: AM allows cooling channels to precisely follow the 3D contours of a component’s surface or internal heat source. This minimizes the distance between the coolant and the heat, drastically improving heat transfer efficiency and creating more uniform temperature distribution, reducing thermal stress and warpage (critical in injection molding and engine components).
- Complex Internal Networks: Intricate, branching, and optimized flow paths can be designed and built directly within a monolithic part. This includes features like variable channel diameters, smooth bends to minimize pressure drop, and integrated manifolds.
- Lattice Structures & TPMS: AM enables the incorporation of internal lattice structures or Triply Periodic Minimal Surfaces (TPMS) within channels or heat sinks. These significantly increase the surface area for heat exchange within a compact volume, boosting thermal performance while potentially reducing weight.
- Part Consolidation & Integration:
- Multiple components of a traditional cooling assembly (e.g., machined plates, tubes, manifolds, brazed joints) can often be redesigned and printed as a single, monolithic part.
- Fördelar: Eliminates potential leak points at joints, reduces assembly time and cost, improves structural integrity, simplifies the supply chain (dealing with one advanced component supplier instead of multiple). This is a significant advantage for procurement managers seeking streamlined sourcing and enhanced reliability.
- Design Freedom & Optimization:
- Engineers are freed from the constraints of traditional manufacturing (DfM – Design for Manufacturing) and can embrace DfAM (Design for Additive Manufacturing), focusing purely on optimizing for function (e.g., thermal performance, fluid dynamics, weight).
- Topology Optimization: Software can be used to computationally design the most efficient cooling channel layout based on defined thermal loads, flow rates, and design space constraints, resulting in organic, highly optimized structures that AM can uniquely produce.
- Thin Walls & Lightweighting: AM can produce very thin, leak-proof walls (down to fractions of a millimeter, depending on the process and material), enabling more compact designs and significant weight reduction, crucial for aerospace and automotive applications.
- Rapid Prototyping & Reduced Lead Times:
- For complex designs, creating prototypes via AM is often significantly faster than tooling up for casting or coordinating the machining and brazing of multi-part assemblies. This accelerates the design iteration cycle, allowing engineers to test and refine concepts quickly.
- While build times for large/complex AM parts can be substantial, the overall lead time from design finalization to functional part can be shorter compared to the multi-step processes of traditional methods, especially for low-to-medium volume production or highly customized parts.
- Material Capabilities:
- AM processes are increasingly capable of reliably processing high-performance materials like pure copper and CuCrZr, which possess superior thermal conductivity essential for high-flow cooling but can be challenging to machine or cast with high precision and complexity. Companies like Met3dp specialize in optimizing printing processes for these demanding materials.
Comparative Overview:
Funktion | Maskinbearbetning | Gjutning | Brazing/Welding | Metal AM (e.g., SLM/DMLS) |
---|---|---|---|---|
Geometrisk komplexitet | Low (Straight Lines) | Medium | Medium (Assemblies) | Very High (Freeform, Conformal) |
Conformal Channels | Very Difficult | Svårt | Difficult (Assembly) | Utmärkt |
Interna funktioner | Limited by Access | Limited by Core | Limited by Joining | Excellent (Complex Networks) |
Konsolidering av delar | Nej | Begränsad | Nej | Utmärkt |
Thin Walls | Possible (Limited) | Svårt | N/A (Assembly) | Utmärkt |
Lead Time (Complex) | Long (Multi-step) | Long (Tooling) | Long (Multi-step) | Moderate-Fast (Prototyping) |
Joint Failure Risk | N/A | N/A | Ja | No (Monolithic) |
Optimering av design | Limited by Process | Limited by Process | Limited by Process | Excellent (Topology Opt.) |
Export to Sheets
While metal AM involves its own set of design considerations (covered later), its fundamental ability to build complex shapes layer-by-layer provides transformative additive manufacturing advantages för att skapa custom cooling channels that deliver superior thermal performance, reliability, and design flexibility compared to traditional approaches. Choosing AM means choosing innovation in thermal management.
Material Focus: Pure Copper (Cu) and Copper Chromium Zirconium (CuCrZr) Powders
The effectiveness of a high-flow cooling channel hinges critically on the material’s ability to absorb and transfer heat rapidly. This is why copper and its alloys are the primary candidates for demanding thermal management applications realized through 3D printing. Their inherent properties make them ideal, although their processing via laser-based additive manufacturing requires specific expertise due to their high reflectivity and conductivity.
Met3dp, leveraging its advanced powder production capabilities including gas atomization and Plasma Rotating Electrode Process (PREP), produces high-quality, spherical metallpulver optimized for AM processes like SLM and SEBM. Our portfolio includes key materials for thermal management, ensuring consistent quality and performance for critical applications. Let’s explore the two primary copper-based materials used for 3D printed cooling channels:
1. Pure Copper (Cu): The Thermal Conductivity Champion
- Beskrivning: Commercially Pure Copper (typically >99.9% Cu) offers the highest thermal and electrical conductivity of any non-precious metal.
- Viktiga egenskaper:
- Exceptional Thermal Conductivity: ~390-400 W/(m·K). This allows for extremely rapid heat absorption and dissipation, making it ideal for applications where maximizing heat transfer is the absolute priority.
- Excellent Electrical Conductivity: Also boasts the highest electrical conductivity, making it suitable for applications requiring both thermal and electrical transfer (e.g., integrated busbars with cooling).
- God korrosionsbeständighet: Generally resistant to corrosion in many environments.
- Lower Mechanical Strength: Pure copper is relatively soft and has lower tensile and yield strength compared to its alloys, especially at elevated temperatures. Its strength decreases significantly above 200°C.
- Printability Challenges: High infrared reflectivity and thermal conductivity make pure copper notoriously difficult to process reliably with standard laser powder bed fusion (LPBF) parameters. It requires high laser power, specific parameter tuning (scan strategies, layer thickness), and often specialized green or blue lasers to overcome absorption issues and achieve dense, defect-free parts. Experienced metal AM suppliers like Met3dp have developed optimized process parameters for pure copper.
- When to Choose Pure Copper:
- Applications where maximizing thermal conductivity is paramount and mechanical loads are relatively low.
- Heat sinks requiring the absolute lowest thermal resistance.
- Certain high-frequency electronic components.
- Cryogenic applications where its conductivity remains high.
2. Copper Chromium Zirconium (CuCrZr): Balanced Performance
- Beskrivning: A precipitation-strengthened copper alloy (typically containing small amounts of Chromium and Zirconium, e.g., UNS C18150).
- Viktiga egenskaper:
- Hög värmeledningsförmåga: While lower than pure copper, CuCrZr still offers excellent thermal conductivity (~300-340 W/(m·K)), significantly higher than steels or nickel alloys.
- God elektrisk ledningsförmåga: Retains high electrical conductivity (~80% IACS), suitable for many electro-thermal applications.
- Significantly Higher Mechanical Strength: Exhibits much higher tensile strength, yield strength, and hardness compared to pure copper, especially after appropriate heat treatment (solution annealing followed by aging).
- Excellent Strength Retention at Elevated Temperatures: Crucially, CuCrZr maintains good mechanical properties at temperatures up to ~450-500°C, making it suitable for applications involving high thermal loads och mechanical stress (e.g., rocket engines, injection molds).
- Förbättrad tryckbarhet: Generally considered easier to process via LPBF than pure copper due to slightly lower reflectivity and conductivity, though it still requires careful parameter control for optimal densification and microstructure.
- Värmebehandlingsbar: Its properties can be tailored through post-print heat treatment cycles.
- When to Choose CuCrZr:
- Applications requiring a combination of high thermal conductivity AND good mechanical strength/hardness, especially at elevated temperatures.
- Injection mold inserts and tooling requiring conformal cooling.
- Rocket engine combustion chamber liners and nozzles.
- High-performance heat exchangers subject to pressure or mechanical loads.
- Welding electrodes.
- Components where wear resistance combined with thermal conductivity is needed.
Material Property Comparison (Typical Values):
Fastighet | Enheter | Pure Copper (Annealed) | CuCrZr (Heat Treated, e.g., C18150) | Anteckningar |
---|---|---|---|---|
Termisk konduktivitet | W/(m·K) | ~390-400 | ~300-340 | CuCrZr significantly higher than steels (~15-50) |
Elektrisk konduktivitet | % IACS | ~100-101 | ~75-85 | IACS = International Annealed Copper Standard |
Täthet | g/cm³ | 8.96 | 8.89 – 8.94 | Similar densities |
Tensile Strength (RT) | MPa | ~200-250 | ~450-550 | CuCrZr significantly stronger |
Yield Strength (RT) | MPa | ~70-100 | ~350-480 | CuCrZr significantly stronger |
Hårdhet | HV / HRB | ~40-60 HV | ~130-170 HV / ~70-85 HRB | CuCrZr significantly harder |
Max. Operating Temp (Strength) | °C | < 200 | ~450-500 | Major advantage for CuCrZr |
Printability (LPBF) | Qualitative | Utmanande | Moderate-Challenging | Requires expertise & optimized parameters |
Export to Sheets
Note: Properties of 3D printed materials can vary based on print parameters, density achieved, and post-processing.
Importance of Powder Quality:
Regardless of the chosen alloy, the quality of the copper 3D printing powder is paramount for successful additive manufacturing. Key powder characteristics influencing print quality include:
- Sfäriskhet: High sphericity ensures good powder flowability and uniform powder bed density, leading to more consistent melting and reduced porosity. Met3dp utilizes advanced gas atomization and PREP technologies to achieve excellent sphericity.
- Fördelning av partikelstorlek (PSD): A controlled PSD tailored to the specific AM machine and layer thickness is crucial for achieving high resolution and density.
- Purity/Low Oxygen Content: Minimizing impurities and oxygen content is vital, as these can negatively impact the material’s conductivity, mechanical properties, and printability.
- Flytbarhet: Good flowability ensures uniform spreading of powder layers during the printing process.
Choosing the right material involves balancing the absolute need for thermal conductivity against the requirements for mechanical strength, operating temperature, and manufacturability. Both Pure Copper and CuCrZr offer compelling advantages when processed correctly using high-quality powders from a reliable metal powder supplier like Met3dp, enabling the creation of high-flow cooling channels that push the boundaries of thermal performance. Consulting with material experts and experienced AM providers is crucial for making the optimal selection for your specific application.
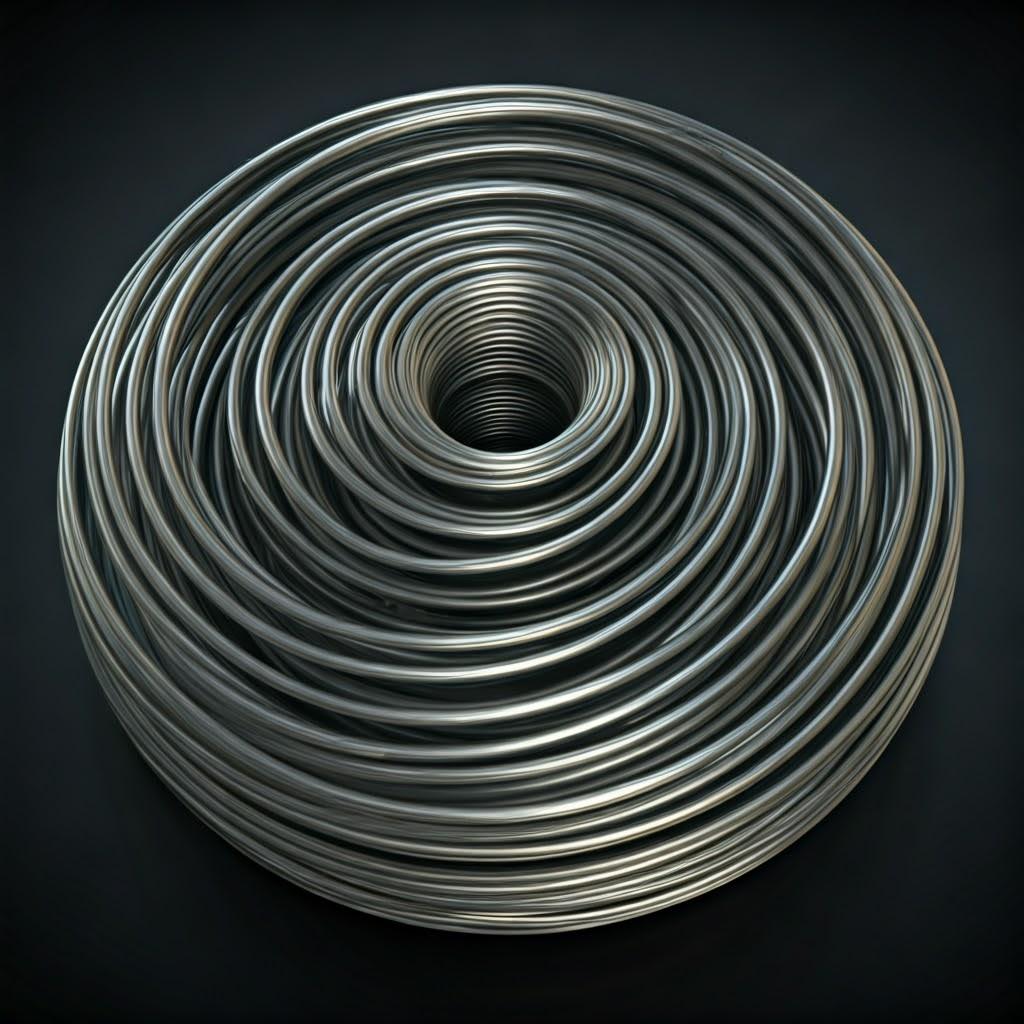
Design for Additive Manufacturing (DfAM): Optimizing Cooling Channel Performance
Simply replicating a traditionally designed cooling channel using 3D printing often fails to capture the full potential of additive manufacturing. To truly leverage the benefits described earlier – unparalleled complexity, conformal paths, integrated features – engineers must embrace Design för additiv tillverkning (DfAM) principles. DfAM for high-flow copper cooling channels involves rethinking the design from the ground up, considering both the functional requirements (thermal performance, fluid dynamics) and the nuances of the chosen tryckmetoder, such as Laser Powder Bed Fusion (LPBF). Partnering with an experienced AM service provider like Met3dp, who offers DfAM support, can significantly accelerate the learning curve and lead to superior outcomes.
Here are key DfAM considerations for optimizing cooling channel design optimization:
- Channel Path Optimization (Conformality & Flow):
- Conformal Design: Instead of straight drilled holes, design channels that follow the complex 3D contours of the heat source or the part’s external shape. This minimizes the thermal path and maximizes heat extraction efficiency. CAD software tools specifically designed for conformal channel generation can assist here.
- Flow Path Efficiency: Design smooth, sweeping bends instead of sharp angles to minimize pressure drop and avoid flow stagnation points. Use flow simulation (Computational Fluid Dynamics – CFD) early in the design process to predict fluid velocity, pressure drop, and heat transfer coefficients. Iterate the design based on simulation results to optimize flow distribution and eliminate “dead zones.”
- Variable Channel Cross-Sections: Taper or vary the channel diameter along its path to maintain optimal coolant velocity or adapt to changing heat loads. AM easily accommodates such variations.
- Leveraging Geometric Complexity:
- Internal Features: Incorporate internal features like ribs, fins, or turbulators directly into the channel design to induce turbulent flow. Turbulent flow significantly enhances the convective heat transfer coefficient compared to laminar flow, boosting cooling performance, albeit at the cost of a higher pressure drop. DfAM makes adding these features relatively simple.
- Lattice Structures & TPMS: For heat sinks or integrated cooling structures, consider using internal lattice structures or Triply Periodic Minimal Surfaces (TPMS) like Gyroids or Schwarz Diamonds. These mathematically defined structures offer extremely high surface area-to-volume ratios, maximizing heat transfer in compact spaces while maintaining structural integrity. They are uniquely manufacturable via AM. Selecting the appropriate unit cell size and density is critical and often requires thermal-fluid simulation.
- AM Process Constraints & Considerations:
- Self-Supporting Angles: Design channels and internal features with overhang angles typically greater than 45 degrees from the horizontal build plane whenever possible. Angles less than this often require internal support structures, which can be extremely difficult or impossible to remove from complex, enclosed channels. Careful part orientation during build setup is crucial.
- Minimum Wall Thickness: While AM can achieve thin walls, there are practical limits determined by the material (copper’s conductivity can make very thin features tricky), machine resolution, and powder particle size. Typical minimum printable wall thickness for robust copper channels might be around 0.4 – 0.8 mm, but this should be verified with the AM provider (like Met3dp). Design walls thick enough to withstand operating pressures and handling.
- Powder Removal: This is a critical DfAM consideration for internal channels. Design channels with sufficient diameter (typically >1-2 mm minimum, larger is better) and include strategically placed access ports or multiple outlets to allow for effective evacuation of unfused powder after printing. Avoid long, narrow, dead-end channels where powder can become trapped. Smooth internal surfaces also aid powder removal.
- Support Structure Strategy: For external features or necessary internal overhangs below the self-supporting angle, design supports that are effective during the build but also relatively easy to remove without damaging the part. Consider the accessibility for tools during post-processing. Sometimes, redesigning the part or changing its build orientation can minimize the need for critical supports.
- Integration and Part Consolidation:
- Actively look for opportunities to combine multiple traditionally separate parts (e.g., manifolds, pipes, heat sinks, mounting brackets) into a single, integrated 3D printed component. This reduces assembly complexity, eliminates potential leak paths, and often lowers overall system weight.
- Ensure integrated designs consider assembly interfaces, mounting points, and connections to the rest of the system. Tolerances for these critical features may need to be achieved through post-print machining.
- Simulation-Driven Design:
- Utilize simulation tools extensively throughout the DfAM process:
- CFD Analysis: Predicts fluid flow behavior, pressure drop, and identifies potential issues like cavitation or stagnation.
- Thermal Analysis (FEA): Simulates heat transfer, predicts temperature distributions, identifies hot spots, and assesses the effectiveness of the cooling channel design.
- Topology Optimization: Helps generate highly efficient, often organic-looking designs based on load cases (thermal and structural) and constraints, removing material where it’s not needed.
- Build Process Simulation: Can predict potential issues like distortion or residual stress during the printing process, allowing for design adjustments or optimized support strategies before printing.
- Utilize simulation tools extensively throughout the DfAM process:
DfAM Workflow Summary:
- Define Thermal Requirements & Constraints
- Initial Concept Design (Leveraging Conformality/Complexity)
- CFD & Thermal Simulation
- Iterative Design Refinement (Adjusting paths, features, diameters)
- Incorporate AM Constraints (Self-supporting angles, powder removal, wall thickness)
- Topology Optimization (Optional, for performance/weight)
- Support Structure Planning & Build Orientation
- Final Design Validation (Simulation & Review)
By adopting these DfAM principles, engineers can move beyond the limitations of traditional manufacturing and unlock the full performance potential of custom cooling channels 3D printed in copper alloys. This often requires a collaborative approach with an AM expert who understands the nuances of the printing process and materials involved.
Achievable Tolerances, Surface Finish, and Dimensional Accuracy
Engineers and procurement managers specifying custom cooling channels must understand the levels of precision achievable with 3D-utskrift av metall, particularly using copper alloys. While AM offers incredible geometric freedom, it typically does not match the ‘out-of-the-box’ tolerances and surface finish of precision machining. Understanding what to expect and planning for necessary post-processing steps is crucial for successful implementation.
Factors Influencing Tolerances and Finish:
- AM-process: Different metal AM technologies (SLM, DMLS, EBM, Binder Jetting) have inherently different accuracy and finish capabilities. Laser Powder Bed Fusion (LPBF), encompassing SLM/DMLS, is commonly used for copper and offers a good balance. Met3dp utilizes advanced LPBF and SEBM systems designed for high accuracy.
- Machine Calibration & Condition: Well-maintained and accurately calibrated machines produce more precise parts.
- Material: Copper’s high thermal conductivity can sometimes influence the melt pool stability and affect fine feature resolution or surface finish compared to materials like steel or titanium. Optimized parameters are key.
- Part Geometry & Size: Larger parts are more susceptible to thermal distortion during the build, which can affect overall dimensional accuracy. Complex geometries with fine features or thin walls may have different achievable tolerances than simpler, blockier parts.
- Bygg orientering: How a part is oriented on the build plate significantly impacts surface finish (up-facing vs. down-facing surfaces) and the need for support structures, which leave witness marks upon removal.
- Skiktets tjocklek: Thinner layers generally result in better surface finish and finer detail resolution but increase build time.
- Laser Parameters: Spot size, power, scan speed, and scan strategy all influence the melt pool characteristics and thus the final accuracy and surface quality.
- Efterbearbetning: As-built tolerances and finish can be significantly improved through various post-processing steps like machining, polishing, or abrasive finishing.
Typical As-Built Values (LPBF Copper/CuCrZr):
- Dimensionell noggrannhet: For well-controlled processes, typical dimensional accuracy for LPBF metal parts is often cited in the range of ±0.1 mm to ±0.2 mm for smaller dimensions (e.g., up to 100 mm), or ±0.1% to ±0.2% for larger dimensions. However, this is highly dependent on the factors listed above. Achieving tighter tolerances often requires post-machining critical features.
- Surface Roughness (Ra):
- Top Surfaces (Parallel to build plate): Generally smoother, perhaps Ra 5-10 µm.
- Vertical Walls (Perpendicular to build plate): Moderate roughness, potentially Ra 8-15 µm, showing layer lines.
- Down-Facing/Supported Surfaces: Typically the roughest due to contact with support structures or partially melted powder, potentially Ra 15-25 µm or higher before support removal and finishing.
- Internal Channels: Surface finish inside channels is harder to control and measure, often rougher than external surfaces, and heavily dependent on orientation and whether supports were needed (which are typically avoided internally). Roughness can enhance turbulent flow but also increases pressure drop.
Improving Tolerances and Surface Finish:
If the as-built state doesn’t meet requirements, various post-processing methods are employed:
- CNC Machining: The most common method for achieving tight tolerances on critical features like mating surfaces, sealing interfaces, or connection ports. Features requiring high precision (e.g., sub-±0.05 mm) are typically designed with extra stock material (‘machining allowance’) for removal during post-machining.
- Polishing/Lapping: Used to achieve very smooth surfaces (low Ra values) for aesthetic reasons, improved fluid dynamics (reduced friction in channels), or specific functional requirements. This can be done manually or using automated processes like abrasive flow machining (AFM) for internal channels.
- Sandblasting/Bead Blasting: Provides a uniform matte finish, removes loose powder particles, and can slightly improve surface roughness, but does not significantly alter dimensional accuracy.
- Electropolishing: An electrochemical process that can smooth surfaces and deburr edges, often used for medical or food-grade applications. Its effectiveness can vary with alloy and part complexity.
Quality Control and Verification:
Ensuring parts meet the specified dimensional accuracy standards and finish requirements is critical, especially for aerospace, medical, or other high-stakes applications. Common quality control methods include:
- Coordinate Measuring Machine (CMM): Provides high-accuracy dimensional measurements.
- 3D Scanning: Captures the overall geometry of the part for comparison against the original CAD model.
- Surface Profilometry: Measures surface roughness (Ra, Rz, etc.).
- CT Scanning: Increasingly used for non-destructively inspecting internal features, channel integrity, and detecting internal defects like porosity, which is crucial for cooling channels. Met3dp employs rigorous quality management systems and inspection methods to ensure parts meet customer specifications.
Setting Expectations:
It’s vital for designers and procurement managers to:
- Clearly define critical tolerances and surface finish requirements on drawings and specifications.
- Distinguish between critical features requiring tight control (often post-machined) and less critical areas where as-built conditions may suffice.
- Discuss these requirements upfront with the AM service provider (like Met3dp) to understand capabilities and plan necessary post-processing steps.
- Factor the cost and time associated with required post-processing into the overall project budget and timeline when submitting an RFQ (Request for Quotation).
By understanding the achievable precision of 3D-utskrift av metall and planning accordingly, businesses can successfully leverage AM for complex copper cooling channels while ensuring the final parts meet all functional and quality requirements.
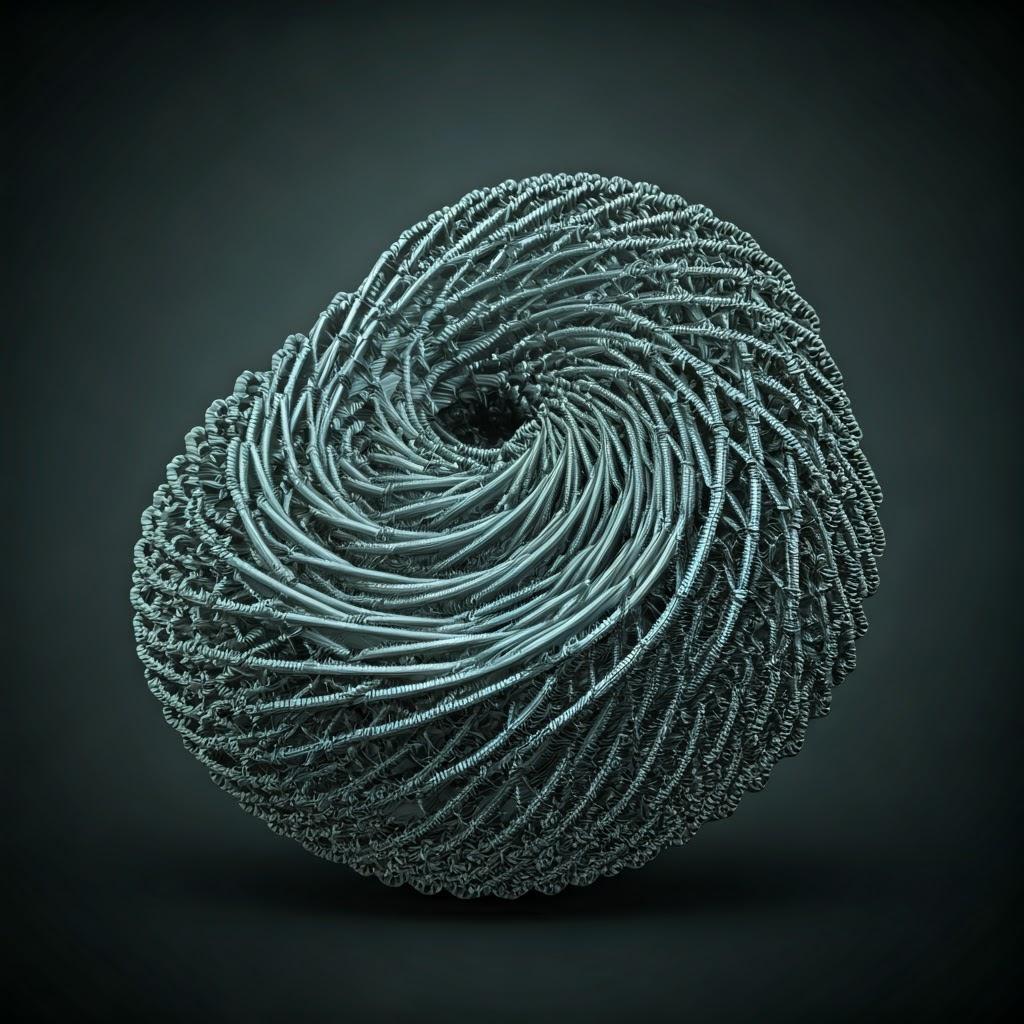
Essential Post-Processing Steps for 3D Printed Copper Channels
A metal 3D printed part, especially a complex component like a high-flow copper cooling channel, rarely comes off the build plate ready for immediate use. Metal AM post-processing is a critical phase that transforms the raw printed part into a functional, reliable component meeting all engineering specifications. The specific steps required depend on the application, material (Cu vs. CuCrZr), design complexity, and quality requirements. Neglecting proper post-processing can compromise performance, durability, and safety.
Here’s a breakdown of common and essential post-processing steps for 3D printed copper and CuCrZr cooling channels:
- Stress Relief / Heat Treatment:
- Syfte: The rapid heating and cooling cycles inherent in LPBF create internal stresses within the printed part. A stress relief heat treatment (typically performed while the part is still attached to the build plate or immediately after removal) is crucial to reduce these stresses, preventing distortion or cracking during subsequent steps or in service.
- Process (Copper): Pure copper typically requires annealing cycles at specific temperatures and durations in a controlled atmosphere (vacuum or inert gas) to relieve stress and potentially soften the material slightly.
- Process (CuCrZr): This alloy benefits from a more complex heat treatment cycle for optimal properties:
- Lösningsglödgning: Heating to a high temperature (e.g., ~950-1000°C) to dissolve the chromium and zirconium into the copper matrix, followed by rapid quenching.
- Aging (Precipitation Hardening): Reheating to a moderate temperature (e.g., ~450-500°C) for a specific duration allows fine precipitates of Cr and Zr to form, significantly increasing strength and hardness while retaining good conductivity.
- Importance: Rätt heat treatment for copper alloys is essential to achieve the desired final mechanical properties (especially for CuCrZr) and ensure long-term dimensional stability. Met3dp possesses the necessary furnace equipment and metallurgical expertise to perform these critical treatments correctly.
- Part Removal from Build Plate:
- Syfte: Separating the printed part(s) from the metal build plate they were fused to during the printing process.
- Metoder: Commonly done using wire EDM (Electrical Discharge Machining) or a bandsaw. Care must be taken to avoid damaging the parts.
- Borttagning av stödstruktur:
- Syfte: Removing the temporary support structures required during printing to anchor the part and support overhanging features.
- Metoder: This is often a manual process involving pliers, cutters, and hand tools. For more intricate or harder-to-reach supports, CNC machining or EDM may be used. This step can be labor-intensive and requires skilled technicians to avoid damaging the part surface. DfAM plays a role here – well-designed supports are easier to remove.
- Surface Impact: Support removal leaves witness marks or ‘scars’ on the surfaces where they were attached. These areas typically require further finishing if a smooth surface is needed.
- Powder Removal (Depowdering):
- Syfte: Thoroughly removing all residual, unfused metal powder from the part, especially from internal cooling channels. Trapped powder can obstruct flow, reduce thermal performance, or become loose during operation, causing contamination or damage downstream.
- Metoder: This involves compressed air blasting, vibration, ultrasonic cleaning baths, and sometimes specialized flushing rigs, particularly for complex internal passages. Designing for depowdering (adequate channel diameters, access ports) is crucial. Verification might involve weighing the part or using borescope inspection or CT scanning.
- Utmaningar: Complete powder removal from long, narrow, or highly complex internal channels is a significant challenge in AM and requires meticulous attention.
- Ytbehandling:
- Syfte: Achieving the required surface roughness (Ra) for functional or aesthetic reasons.
- Metoder:
- Blasting (Sand, Bead, Shot Peening): Creates a uniform matte finish, cleans the surface. Shot peening can also impart compressive stress, improving fatigue life.
- Tumbling/Vibratory Finishing: Uses abrasive media in a rotating or vibrating drum to deburr edges and smooth surfaces (generally for external features).
- Polishing/Lapping: Manual or automated processes to achieve mirror-like finishes on specific surfaces.
- Abrasive Flow Machining (AFM) / Extrude Hone: Forces an abrasive putty through internal channels to smooth walls and radius edges, improving flow characteristics.
- Electropolishing: Electrochemical smoothing.
- CNC Machining:
- Syfte: To achieve tight tolerances on critical dimensions, create precise mating surfaces, thread holes, or produce features not possible in the as-printed state.
- Process: Uses traditional milling, turning, or grinding operations on specific areas of the 3D printed part. Requires careful fixture design to hold the complex AM part securely. Designing parts with appropriate machining allowances is necessary. Achieving tight CNC machining tolerances on AM parts requires expertise.
- Cleaning & Inspection:
- Syfte: Final cleaning to remove any machining fluids, debris, or handling contaminants. Comprehensive inspection ensures the part meets all specifications before shipment.
- Metoder: Final cleaning using appropriate solvents or aqueous solutions. Inspection methods include:
- Visuell inspektion
- Dimensional Inspection (CMM, 3D Scanning)
- Surface Roughness Measurement
- Leak Testing: Essential for cooling channels to ensure integrity under pressure. Parts are pressurized (e.g., with air or helium) underwater or using mass spectrometry leak detection.
- Icke-förstörande testning (NDT): CT scanning is invaluable for verifying internal channel clearance, wall thickness, and detecting internal defects like porosity. Dye penetrant or radiographic testing might also be used.
Supplier Capability:
Handling these diverse metal AM post-processing steps efficiently and correctly requires significant investment in equipment, process control, and skilled personnel. When selecting a manufacturing partner, ensure they have the in-house capabilities or established relationships with trusted vendors to manage the entire workflow, from printing to final inspection and quality inspection services. Met3dp offers comprehensive solutions, managing these steps to deliver finished, application-ready components.
Common Challenges in Printing Copper Cooling Channels and Mitigation Strategies
Medan 3D-utskrift av metall unlocks incredible possibilities for copper cooling channels, it’s not without its challenges, particularly due to the inherent physical properties of copper and its alloys. Successfully manufacturing high-quality, dense, and reliable copper components requires deep process understanding, optimized equipment, and robust quality control. Awareness of these potential issues allows engineers and manufacturers like Met3dp to implement effective mitigation strategies.
Key Challenges & Mitigation Approaches:
- Laser Reflectivity & Absorption:
- Utmaning: Pure copper and its alloys have high reflectivity (reflecting >90%) and low absorptivity for the infrared (IR) lasers (~1 µm wavelength) commonly used in standard LPBF machines. This makes it difficult for the powder to absorb enough laser energy consistently for stable melting, potentially leading to incomplete fusion, high porosity, and poor layer-to-layer bonding.
- Mitigation Strategies:
- High-Power Lasers: Using lasers with significantly higher power (e.g., >500W or 1kW) helps deliver sufficient energy density to overcome reflectivity.
- Optimized Laser Parameters: Employing specific parameters like modified spot sizes, slower scan speeds, specific hatch patterns (e.g., re-melting scans), and adjusted energy density inputs tailored for copper.
- Alternative Laser Wavelengths: Green lasers (~515 nm) or blue lasers (~450 nm) offer significantly higher absorption rates in copper compared to IR lasers, leading to more stable processing and better part density. Machines equipped with these lasers are increasingly available but represent a higher investment.
- Powder Modification (Less Common): Surface treatment of powders to improve absorption (potential contamination risk).
- Process Expertise: Relying on providers like Met3dp with proven experience and validated parameter sets specifically developed for copper 3D printing challenges.
- Hög värmeledningsförmåga:
- Utmaning: Copper’s excellent thermal conductivity, while beneficial for the end application, poses challenges during printing. Heat dissipates very quickly from the small melt pool generated by the laser, making it difficult to maintain a stable melt pool size and temperature. This rapid cooling can lead to solidification issues, potential keyholing (vapor depressions causing porosity), balling (powder forming spheres instead of melting smoothly), and high residual stresses.
- Mitigation Strategies:
- Optimized Scan Strategies: Using specific patterns (e.g., island scanning, checkerboard patterns) to manage heat distribution across the layer.
- Build Plate Heating: Preheating the build plate to elevated temperatures reduces thermal gradients between the molten material and the surrounding powder/solidified part, promoting more stable melting and reducing residual stress.
- Careful Parameter Control: Fine-tuning laser power, scan speed, and hatch spacing is critical to balance energy input and heat dissipation.
- Thermal Simulation: Using build process simulation tools to predict heat buildup and potential distortion, allowing for adjustments to parameters or support strategies.
- Porositetskontroll:
- Utmaning: Achieving near-full density (>99.5% or higher) is critical for the mechanical integrity and thermal performance of cooling channels. Porosity can arise from incomplete fusion (due to reflectivity/conductivity issues), keyholing (process instability), or gas trapped within the powder or melt pool.
- Mitigation Strategies:
- Optimized Print Parameters: As discussed above, stable melt pool conditions achieved through careful parameter selection are key.
- High-Quality Powder: Using spherical powder with controlled PSD and low internal gas porosity (achieved via methods like Met3dp’s gas atomization or PREP).
- Inert Atmosphere Control: Maintaining a high-purity inert gas atmosphere (Argon or Nitrogen) in the build chamber minimizes oxidation and contamination, which can contribute to porosity.
- Het isostatisk pressning (HIP): A post-processing step where the part is subjected to high temperature and high isostatic pressure. HIP can effectively close internal pores, significantly increasing density and improving mechanical properties. Often required for critical applications (e.g., aerospace).
- Cracking:
- Utmaning: High thermal gradients and rapid solidification can induce significant residual stresses, potentially leading to solidification cracking or delamination between layers, especially in complex geometries or large parts.
- Mitigation Strategies:
- Stress Relief Heat Treatments: Performing appropriate thermal cycles post-build (or even intermediate treatments during long builds).
- Optimized Scan Strategies & Part Orientation: Minimizing large cross-sectional areas per layer and orienting parts to reduce stress buildup.
- Build Plate Heating: Reduces thermal gradients.
- Alloy Selection: Some alloys might be more prone to cracking than others; process parameters must be adjusted accordingly.
- Support Removal Difficulties:
- Utmaning: Copper and CuCrZr are relatively ductile but strong (especially CuCrZr after heat treatment). Support structures made from the same material can be difficult and time-consuming to remove cleanly without damaging the part, especially intricate supports or those in confined areas. Internal supports in cooling channels are particularly problematic.
- Mitigation Strategies:
- DfAM for Minimal Supports: Designing parts with self-supporting angles (>45°) wherever possible, optimizing build orientation.
- Optimized Support Structures: Using support designs (e.g., thin tips, perforations, specific patterns) that provide adequate anchoring during the build but are easier to break away or machine off later.
- Specialized Removal Techniques: Utilizing wire EDM or precision CNC machining for support removal in critical areas.
- Avoid Internal Supports: Redesigning internal channels to be self-supporting is highly preferable.
- Powder Handling & Safety:
- Utmaning: Fine metal powders, including copper, can be reactive and pose inhalation risks. Proper handling procedures and personal protective equipment (PPE) are essential. Copper powder’s high conductivity also requires specific considerations regarding electrostatic discharge.
- Mitigation Strategies:
- Controlled Environments: Using dedicated powder handling stations with appropriate ventilation and grounding.
- Personlig skyddsutrustning (PPE): Mandating respirators, gloves, and protective clothing.
- Inert Gas Handling: Storing and handling powder under inert gas where possible to prevent oxidation.
- Training: Ensuring personnel are properly trained on safe powder handling protocols.
Successfully navigating these AM troubleshooting scenarios requires a combination of advanced machine capabilities, optimized materials, validated process parameters, and deep engineering expertise. Partnering with a knowledgeable provider like Met3dp, who proactively addresses these copper 3D printing challenges, is crucial for obtaining high-quality, reliable custom cooling channels.
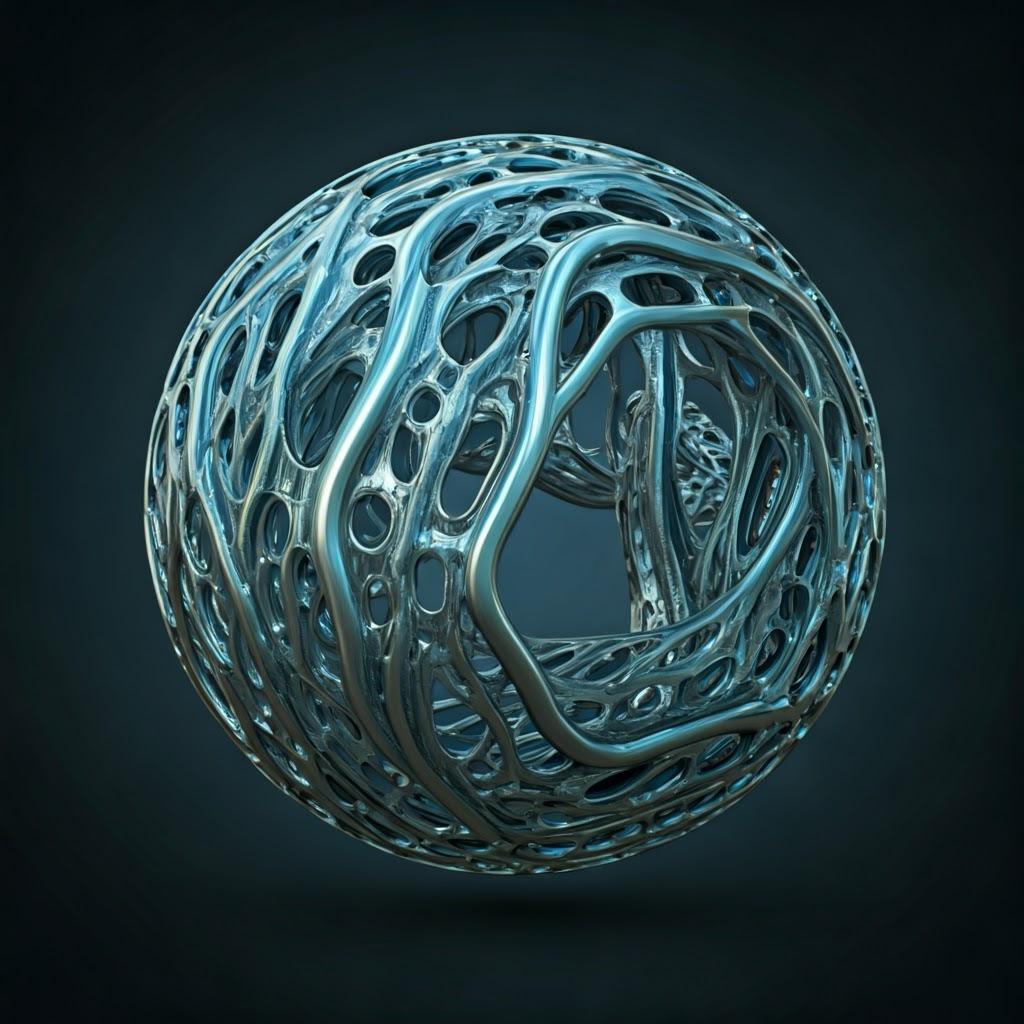
Selecting the Right Metal 3D Printing Service Provider for Copper Components
Choosing the right manufacturing partner is as critical as the design and material selection when embarking on a project involving 3D printed copper cooling channels. The unique challenges associated with printing copper alloys demand specialized expertise and equipment that not all additive manufacturing service bureaus possess. For engineers and procurement managers, conducting thorough supplier evaluation is essential to ensure project success, component quality, and supply chain reliability. Making the right choice establishes a B2B manufacturing partner capable of delivering complex, high-performance thermal solutions.
Here are key criteria to consider when selecting a metal AM service bureau för din copper printing needs:
- Proven Expertise with Copper Alloys:
- Track Record: Does the provider have demonstrable experience successfully printing pure copper and/or CuCrZr? Ask for case studies, sample parts (if possible), or references related to thermal management components or similar challenging geometries in copper.
- Technical Understanding: Do their engineers understand the specific challenges of copper AM (reflectivity, conductivity, parameter optimization, heat treatment)? Can they discuss DfAM principles relevant to your cooling channel design?
- Problem Solving: Inquire about their approach to potential issues like porosity, cracking, or powder removal specific to copper.
- Appropriate Technology and Equipment:
- Machine Capability: Do they operate LPBF machines suitable for copper? This often means high-power lasers (IR or Green/Blue), excellent inert atmosphere control, and potentially build plate heating capabilities. What is their build volume capacity?
- Materialhantering: Do they have established procedures for safely handling and recycling copper powders to maintain purity and ensure traceability?
- Post-Processing Facilities: Evaluate their in-house or validated third-party capabilities for critical post-processing steps like heat treatment (vacuum/inert atmosphere furnaces), CNC machining, support removal, powder removal (especially for internal channels), surface finishing, and comprehensive inspection (including leak testing and potentially CT scanning).
- Material Quality and Traceability:
- Powder Sourcing & Quality: Where do they source their copper and CuCrZr powders? Do they use high-quality, spherical powders with controlled PSD, optimized for AM? Reputable providers like Met3dp manufacture their own powders or source from qualified suppliers with rigorous quality control and provide material certifications.
- Material Certifications: Can they provide certificates of conformity (CoC) and full material traceability documentation linking the powder batch to the final part? This is often mandatory for aerospace, medical, and defense applications.
- Quality Management Systems (QMS) and Certifications:
- Formal QMS: Does the provider operate under a robust QMS, such as ISO 9001? This indicates a commitment to consistent processes, quality control, and continuous improvement.
- Industry-Specific Certifications: For industries like aerospace or medical, certifications such as AS9100 (Aerospace) or ISO 13485 (Medical Devices) are often required. These demonstrate adherence to the stringent quality and documentation standards demanded by those sectors. Check if their certifications are relevant to your application’s requirements. Met3dp’s commitment to quality is reflected in our operational standards and pursuit of relevant certifications.
- Engineering and DfAM Support:
- Collaboration: Are they willing to work collaboratively with your design team? Can they provide expert feedback on your design’s printability (DfAM review)?
- Optimization Services: Do they offer services like topology optimization, flow simulation, or build simulation to help enhance performance and manufacturability? Early engagement with a knowledgeable partner like Met3dp during the design phase can prevent costly redesigns later. Learn more about our approach on our om oss sida.
- Capacity, Lead Times, and Communication:
- Produktionskapacitet: Can they handle your required volume, from prototypes to potential series production?
- Quoting Process (RFQ): Is their RFQ process clear, transparent, and timely? Do quotes provide a detailed breakdown of costs?
- Lead Time Estimates: Är deras lead time estimations realistic and consistently met? Understand the factors that influence lead times (complexity, quantity, post-processing).
- Communication: Is their communication clear, responsive, and professional? A good partner keeps you informed throughout the manufacturing process.
- Cost vs. Value:
- While cost is always a factor, the cheapest option may not offer the necessary expertise or quality for demanding copper applications. Evaluate the overall value proposition, considering technical capability, quality assurance, support, and reliability alongside the price. The cost of failure due to poor component quality often far outweighs upfront savings.
Due Diligence Steps:
- Request quotes from multiple qualified providers.
- Conduct technical interviews or audits (virtual or in-person).
- Ask for references or case studies specific to copper or thermal applications.
- Review their quality certifications and material documentation procedures.
- Start with a pilot project or prototype run to assess capabilities before committing to larger volumes.
Selecting the right provider is an investment in the success of your project. A capable partner like Met3dp brings not only advanced manufacturing technology but also the crucial materials science and process expertise needed to reliably produce high-performance custom copper cooling channels.
Understanding Cost Drivers and Lead Times for Custom Cooling Channels
Budget and schedule are critical constraints for any engineering project. When considering metal additive manufacturing for custom copper cooling channels, understanding the factors that influence metal 3D printing cost och lead time estimation is essential for effective planning, budgeting, and managing expectations through the procurement cycle. The pricing for AM parts is typically more complex than traditional volume manufacturing due to the interplay of various factors.
Key Cost Drivers:
- Part Design & Complexity:
- Volume & Bounding Box: The overall size of the part directly impacts material consumption and the amount of machine time required. Larger parts occupying more space in the build chamber cost more.
- Geometric Complexity: Highly intricate designs with complex internal channels, thin walls, or features requiring extensive support structures increase build time and post-processing effort (especially support and powder removal), driving up costs.
- Stödstrukturer: The volume of required support material adds to both material cost and the labor needed for removal. Designs optimized via DfAM to minimize supports can reduce costs.
- Material Cost:
- Powder Price: High-performance metal powders, especially specialized alloys or highly refined copper powders suitable for AM, are significantly more expensive than raw material stock used in traditional manufacturing. The cost of CuCrZr powder generally differs from pure copper. The price per kilogram is a major cost component. Reliable metal powder suppliers ensure quality, impacting final part cost and reliability.
- Material Consumption: Includes the material making up the final part, the material used for support structures, and potential material waste or recycling losses inherent in the process.
- Maskintid:
- Byggtid: This is often the most significant cost driver. It’s influenced by:
- Part Height: The primary factor determining the number of layers and thus the overall print duration.
- Part Density & Volume: The amount of material that needs to be melted per layer affects the time taken for laser scanning.
- Number of Parts per Build: Economies of scale can be achieved by nesting multiple parts efficiently within a single build chamber, distributing the machine setup and operation costs.
- Machine Depreciation & Operational Costs: The high capital cost of industrial metal AM systems, plus consumables (filters, inert gas) and energy, are factored into the hourly machine rate.
- Byggtid: This is often the most significant cost driver. It’s influenced by:
- Krav på efterbearbetning:
- Labor Intensity: Many post-processing steps (support removal, manual finishing) are labor-intensive and require skilled technicians, adding significant cost.
- Required Steps: The specific steps needed (heat treatment, machining, polishing, leak testing, HIP, CT scanning) each add their own costs related to equipment, consumables, and labor/expertise. Complex finishing or tight CNC machining tolerances increase these costs substantially.
- Quality Assurance & Inspection:
- The level of required inspection (visual, dimensional CMM, surface roughness, leak testing, NDT like CT scanning) adds time and cost. Stringent requirements for aerospace or medical parts naturally increase the QA cost component.
- Order Volume:
- Prototyping vs. Production: One-off prototypes are typically expensive due to setup costs not being amortized.
- Volymrabatter: For larger quantities or series production, order volume discounts may apply as setup costs are spread, machine utilization is optimized (full builds), and process efficiencies can be realized. Discuss bulk pricing options during the RFQ process.
Typical Lead Times:
Lead time refers to the total time from order confirmation to part shipment. For 3D printed copper cooling channels, it can vary significantly:
- Prototyping: For a single, moderately complex prototype, lead times might range from 1 to 4 weeks, depending on provider backlog, part size/complexity, and required post-processing.
- Low-Volume Production: For small batches (e.g., 5-50 units), lead times could be 3 to 8 weeks or more, influenced heavily by machine availability and the extent of post-processing and QA.
- Series Production: Establishing stable series production requires significant planning and dedicated capacity, with lead times agreed upon based on volume and delivery schedules.
Factors Influencing Lead Times:
- Machine Availability: Current workload and scheduling at the service provider.
- Byggtid: As described above, directly impacts the printing phase.
- Post-Processing Complexity: Extensive machining, finishing, or complex inspection adds considerable time. Heat treatment cycles also add specific durations.
- Materialets tillgänglighet: Ensuring the specific copper powder grade is in stock.
- Data Preparation: Time required for build file setup, support generation, and simulation (if needed).
- Frakt: Transit time to the final destination.
Obtaining Accurate Quotes:
To get reliable cost and lead time estimates:
- Provide a complete 3D CAD model (e.g., STEP format).
- Include a 2D drawing specifying critical dimensions, tolerances, surface finishes, material (Cu or CuCrZr), heat treatment requirements, and any specific inspection criteria.
- Clearly state the required quantity and desired delivery date.
- Engage with potential suppliers early to discuss DfAM possibilities that could reduce cost and lead time.
By understanding these cost and lead time drivers, businesses can better plan their projects, optimize designs for manufacturability, and engage effectively with metal AM service providers to procure high-performance copper cooling channels within budget and schedule constraints.
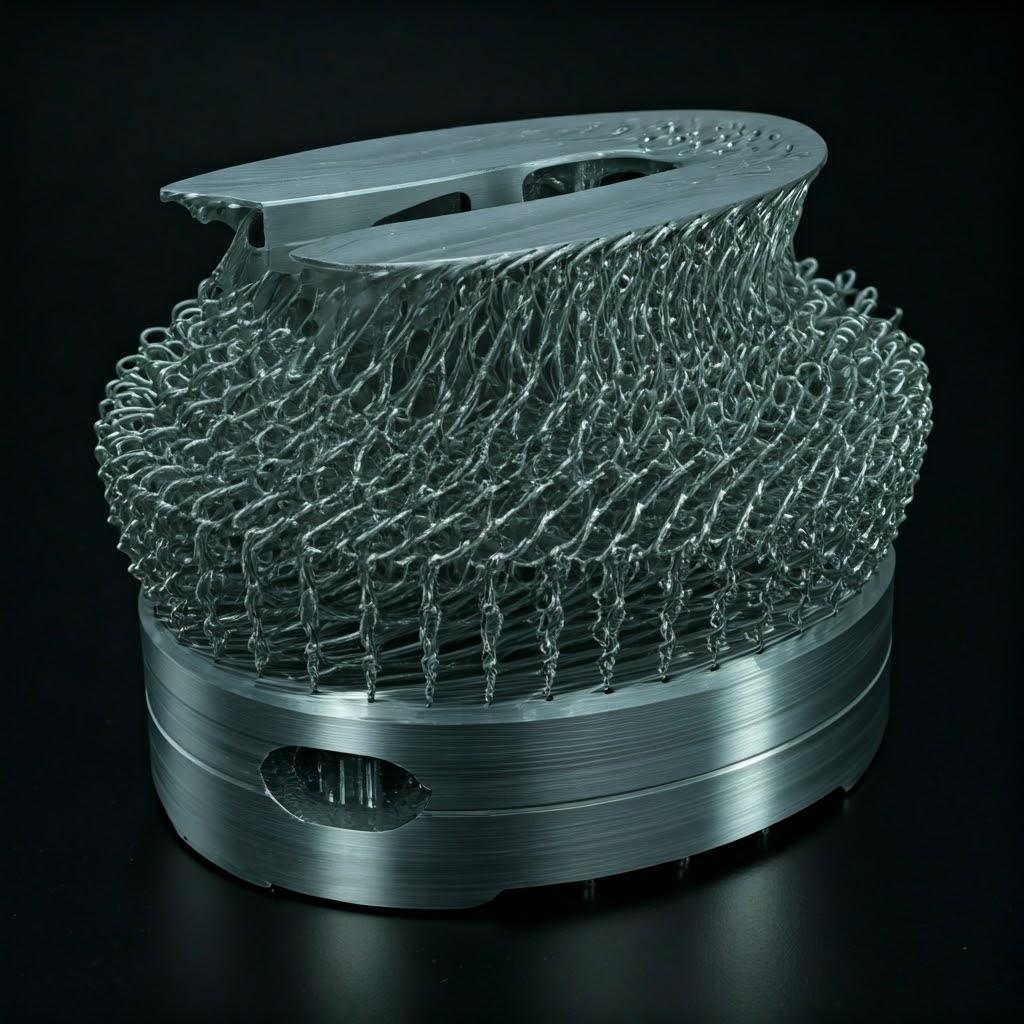
Frequently Asked Questions (FAQ) about 3D Printed Copper Cooling Channels
Here are answers to some common questions engineers and procurement managers have about using additive manufacturing for copper cooling channels:
1. How does the thermal performance of 3D printed copper channels compare to traditionally made ones?
- Generally, 3D printed copper cooling channels can offer överlägsen thermal performance compared to traditionally manufactured counterparts (e.g., drilled or cast channels). This is primarily due to AM’s ability to create:
- Conformal Channels: Paths that closely follow the heat source reduce the thermal distance and improve heat extraction efficiency.
- Komplexa geometrier: Internal fins, turbulators, or lattice structures can be integrated to increase surface area and induce turbulence, significantly enhancing the heat transfer coefficient.
- Optimized Flow Paths: Simulation-driven design allows for smoother bends and variable cross-sections, minimizing pressure drop while maximizing heat removal where needed.
- While the as-printed surface finish might be slightly rougher internally (which can actually promote beneficial turbulence), the geometric advantages enabled by AM typically outweigh this, leading to overall better 3D printing thermal performance. Material properties (thermal conductivity) of dense, properly processed AM copper are comparable to wrought copper.
2. What are the typical cost differences between AM and traditional methods for complex cooling channels?
- The cost comparison depends heavily on the complexity of the design and the production volume:
- Simple Channels (e.g., straight drilled holes): Traditional machining is almost always cheaper.
- Moderately Complex Channels: Costs may be comparable, depending on the specific geometry and traditional manufacturing steps involved (e.g., multi-axis machining, brazing multiple components).
- Highly Complex / Conformal Channels: For geometries that are very difficult or impossible to make traditionally (requiring extensive EDM, multiple complex brazed joints, or are simply unmanufacturable), AM vs traditional cooling cost becomes highly favorable for AM, especially when considering the performance benefits. AM excels where complexity is high, and volume is low-to-medium.
- Del Konsolidering: If AM allows consolidating multiple parts into one, it can reduce assembly costs, inventory management, and potential failure points, improving the total cost of ownership even if the per-part print cost seems higher initially.
- Tooling Costs: AM avoids the high upfront tooling costs associated with casting or injection molding, making it cost-effective for custom parts and low volumes.
3. Which copper alloy (Pure Cu vs. CuCrZr) is better for my specific application?
- The choice depends on balancing thermal conductivity needs with mechanical strength and operating temperature requirements:
- Choose Pure Copper (Cu) if:
- Maximizing thermal conductivity (~400 W/m·K) is the absolute highest priority.
- Mechanical loads and operating temperatures are relatively low (generally below 200°C where strength significantly drops).
- Examples: High-performance heat sinks, certain electronics cooling, cryogenic applications.
- Choose Copper Chromium Zirconium (CuCrZr) if:
- A combination of high thermal conductivity (~320 W/m·K) och good mechanical strength/hardness is needed.
- The component will operate at elevated temperatures (up to ~450-500°C), requiring strength retention.
- Resistance to wear or deformation under load is important.
- Examples: Rocket engine components, injection mold inserts, structural heat exchangers, welding electrodes.
- Consult the material property comparison table (in Part 1) and discuss your specific operating conditions (temperature, pressure, mechanical loads) with your copper alloy selection guide or AM provider like Met3dp.
- Choose Pure Copper (Cu) if:
4. What is the maximum size/complexity achievable for 3D printed copper cooling channels?
- Size: Limited by the build volume of the available metal AM machines. Common industrial LPBF systems have build volumes ranging from ~250x250x300 mm to ~500x300x400 mm, with larger format machines (e.g., 800 mm or more in X/Y) becoming available. Met3dp offers systems with industry-leading print volumes. Extremely large components might need to be printed in sections and joined post-build (e.g., via welding or brazing), though this reintroduces joints.
- Komplexitet: AM allows for extremely high geometric complexity, including intricate internal channels, lattices, and freeform shapes. The primary limitations are:
- Minsta funktionsstorlek: Related to laser spot size and powder particle size (typically ~0.1-0.2 mm).
- Minimum Wall Thickness: Practically ~0.4-0.8 mm for robust channels.
- Internal Channel Access: Channels must be designed for effective powder removal (typically >1-2 mm diameter, with clear exit paths).
- Borttagning av stödstruktur: Overly complex designs requiring extensive, inaccessible internal supports may not be feasible. DfAM practices are key to designing complexity that is also manufacturable.
5. Can 3D printed copper channels be easily integrated into existing assemblies?
- Yes, absolutely. Integration is a key consideration:
- Machined Interfaces: Critical mating surfaces, mounting holes, and connection ports (e.g., for fluid fittings) are typically CNC machined after printing to achieve the precise tolerances required for seamless integration with other components.
- Joining: 3D printed copper parts can often be joined to other components using traditional methods like brazing, welding, or mechanical fasteners, provided the joint is designed appropriately and the post-print material condition is suitable.
- Designöverväganden: Integration points should be considered during the DfAM phase, ensuring adequate material is present for machining or joining features.
Addressing these FAQs highlights the capabilities and considerations surrounding 3D printed copper cooling channels, empowering better decision-making for adopting this advanced manufacturing technology.
Conclusion: Partnering for Superior Thermal Performance with Met3dp
The challenge of managing heat effectively is a critical bottleneck across numerous high-performance industries. Traditional manufacturing methods, while established, often impose limitations on the complexity and efficiency of cooling channel designs. As we’ve explored, additiv tillverkning av metall, specifically utilizing the exceptional thermal properties of copper and CuCrZr alloys, offers a transformative solution. By enabling the creation of highly complex, conformal, and integrated high-flow cooling channels, AM unlocks unprecedented levels of thermal performance, component reliability, and design freedom.
From enhancing the efficiency of rocket engines and electric vehicles to optimizing industrial tooling and cooling high-power electronics, the applications are vast and growing. The advantages – superior heat transfer, part consolidation, reduced lead times for complex prototypes, and the ability to realize optimized designs previously thought impossible – provide compelling value propositions for both engineers striving for performance breakthroughs and procurement managers seeking reliable, efficient sourcing solutions.
However, harnessing the full potential of copper 3D printing requires navigating specific challenges related to material properties and process complexities. Success hinges on a combination of advanced DfAM principles, optimized printing processes, meticulous post-processing, and rigorous quality control. This underscores the importance of selecting the right manufacturing partner.
Met3dp stands as a leader in providing comprehensive additiv tillverkningslösningar. Our capabilities extend from the development and production of high-quality, spherical metallpulver using industry-leading gas atomization and PREP technologies, to operating state-of-the-art 3D-utskrift av metall systems delivering exceptional accuracy and reliability. Our team possesses deep expertise in processing challenging materials like copper alloys and offers crucial engineering support throughout the design and manufacturing lifecycle.
We partner with organizations in aerospace, automotive, medical, and industrial sectors to implement cutting-edge thermal management solutions, leveraging our decades of collective expertise in metal AM. Whether you need rapid prototypes or series production of complex custom copper components, Met3dp provides the technology, materials, and knowledge to accelerate your innovation.
Ready to revolutionize your thermal management strategy? Explore the possibilities of 3D printed copper cooling channels.
Contact Met3dp today to discuss your project requirements, request a quote, or learn more about how our capabilities can power your organization’s additive manufacturing goals.
Dela på
Facebook
Twitter
LinkedIn
WhatsApp
E-post
MET3DP Technology Co, LTD är en ledande leverantör av lösningar för additiv tillverkning med huvudkontor i Qingdao, Kina. Vårt företag är specialiserat på 3D-utskriftsutrustning och högpresterande metallpulver för industriella tillämpningar.
Förfrågan för att få bästa pris och anpassad lösning för ditt företag!
Relaterade artiklar
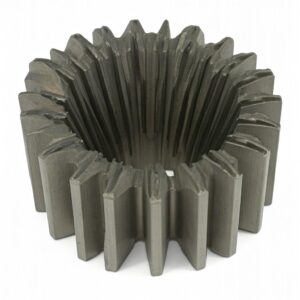
Högpresterande segment för munstycksvingar: Revolutionerande turbineffektivitet med 3D-utskrift i metall
Läs mer "
13 maj 2025
Inga kommentarer
Om Met3DP
Senaste uppdateringen
Vår produkt
KONTAKTA OSS
Har du några frågor? Skicka oss meddelande nu! Vi kommer att betjäna din begäran med ett helt team efter att ha fått ditt meddelande.
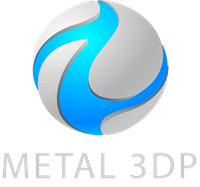
Metallpulver för 3D-printing och additiv tillverkning
FÖRETAG
PRODUKT
cONTACT INFO
- Qingdao City, Shandong, Kina
- [email protected]
- [email protected]
- +86 19116340731