3D Printing Aluminum Steering Knuckles for Lightweighting
Obsah
Introduction: Revolutionizing Automotive Components with 3D Printed Aluminum Steering Knuckles
The automotive industry is in a constant state of evolution, driven by relentless demands for improved performance, enhanced fuel efficiency, stringent safety standards, and the seismic shift towards electrification. At the heart of vehicle dynamics lies the suspension and steering system, where components must balance strength, durability, and weight with meticulous precision. Among these critical parts is the steering knuckle, a vital link connecting the steering, suspension, and braking systems to the wheel hub. Traditionally manufactured through casting or forging, steering knuckles face inherent limitations in design complexity and weight optimization. However, the advent of výroba aditiv kovů (AM), běžně známý jako kov 3D tisk, is ushering in a new era for components like these, particularly with the use of advanced aluminum alloys. This technology allows engineers to reimagine steering knuckle design, unlocking unprecedented levels of lightweighting and performance optimization previously unattainable.
What is a Steering Knuckle?
Before delving into the transformative potential of 3D printing, let’s establish the fundamental role of the steering knuckle. Also referred to as an upright or hub carrier, the steering knuckle is a pivotal component in a vehicle’s chassis system. Its primary functions include:
- Housing the Wheel Hub/Bearing: It provides a secure mounting point for the wheel hub assembly, allowing the wheel to rotate freely.
- Connecting Suspension Components: It serves as the attachment point for upper and lower control arms (or MacPherson strut), connecting the suspension system to the wheel assembly.
- Linking Steering Components: The tie rod end attaches to the steering knuckle, translating steering inputs from the driver into angular movement of the wheel.
- Mounting Brake Components: The brake caliper and rotor are typically mounted directly onto the steering knuckle.
Given these critical roles, the steering knuckle must withstand significant dynamic loads, including cornering forces, braking torque, impacts from road imperfections, and the static weight of the vehicle. Therefore, its structural integrity, stiffness, and fatigue resistance are paramount for vehicle safety and handling performance.
Traditional Manufacturing: Challenges and Limitations
For decades, steering knuckles have been predominantly produced using established manufacturing processes:
- Obsazení: Molten metal (typically cast iron or aluminum alloys) is poured into a mold shaped like the desired knuckle. While cost-effective for high-volume production, casting often results in heavier parts due to design constraints needed for mold filling and solidification. Achieving complex internal structures or highly optimized geometries is difficult, and porosity can be a concern, potentially impacting strength.
- Kování: A metal billet is heated and shaped under immense pressure using dies. Forging generally produces parts with excellent strength and fatigue properties due to the refined grain structure. However, forging tooling is expensive, making it less suitable for low-to-medium volume production or frequent design iterations. The process also offers less design freedom compared to casting or AM, often requiring significant post-machining to achieve final dimensions and features.
Both traditional methods face challenges when it comes to odlehčení – a critical goal in modern automotive design. Reducing unsprung mass (the mass of components not supported by the suspension, including wheels, tires, brakes, and knuckles) significantly improves vehicle handling, ride comfort, acceleration, and fuel efficiency (or battery range in EVs). Every kilogram saved in unsprung mass can feel like multiple kilograms saved in sprung mass in terms of dynamic performance. However, achieving substantial weight reduction in a structurally critical part like a steering knuckle using casting or forging often requires compromises in strength or necessitates the use of more expensive materials and complex, costly processing steps. Design optimization is limited by the constraints of mold creation or die forging.
The Advent of Additive Manufacturing (AM)
Metal 3D printing, particularly Laserová fúze v práškovém loži (LPBF) – also known as Selective Laser Melting (SLM) – offers a radical departure from these traditional constraints. LPBF builds parts layer by layer directly from a digital CAD model by selectively melting fine metal powder particles with a high-power laser. This process fundamentally changes the design paradigm.
- Svoboda designu: Engineers are no longer bound by the limitations of molds or dies. Complex geometries, intricate internal channels (e.g., for cooling or hydraulic lines), and highly optimized, topology-driven shapes become feasible.
- Potenciál odlehčení: AM enables optimalizace topologie, where software algorithms determine the most efficient material distribution to meet specific load requirements. This allows for the creation of skeletal, organic-looking structures that minimize weight while maintaining or even enhancing stiffness and strength compared to their traditionally manufactured counterparts.
- Material Possibilities: AM opens the door to using advanced alloys that might be difficult or impossible to cast or forge effectively. This includes high-strength aluminum alloys specifically designed for demanding applications.
- Rapid Prototyping & Iteration: New steering knuckle designs can be prototyped and tested much faster and more cost-effectively than with traditional methods requiring expensive tooling. This accelerates the development cycle and allows for greater design refinement.
Focus on Aluminum Lightweighting
Aluminum alloys are particularly attractive for steering knuckles due to their excellent strength-to-weight ratio. While aluminum knuckles are already used in many vehicles (often cast or forged), AM allows for even greater weight savings. By combining the inherent low density of aluminum with the design freedom of 3D printing and topology optimization, engineers can achieve weight reductions of 30-50% or even more compared to traditional steel or even optimized cast aluminum designs, without compromising structural integrity. This is particularly crucial for:
- Electric Vehicles (EVs): Reducing overall vehicle weight is critical for maximizing battery range. Lightweighting components like steering knuckles contributes directly to this goal.
- Performance Vehicles: Lowering unsprung mass drastically improves handling responsiveness, grip, and braking performance.
- Motorsport: Every gram counts, and 3D printed aluminum knuckles offer a competitive edge through optimized weight and customized geometry.
Companies at the forefront of metal additive manufacturing, like Met3dp, are playing a crucial role in this transition. With expertise in both advanced powder production and industrial 3D printing systems, Met3dp provides the foundational technologies necessary to realize these next-generation automotive components. Their focus on high-quality, spherical metal powders, produced using advanced gas atomization techniques, ensures the consistency and material properties required for mission-critical parts like steering knuckles. The ability to leverage alloys like AlSi10Mg and high-strength A7075 through AM processes empowers automotive engineers and procurement managers to push the boundaries of vehicle performance and efficiency. The integration of AM into the supply chain allows automotive component distributors and wholesale suppliers to offer cutting-edge, lightweight solutions to their clients, meeting the evolving demands of the market.
In summary, the application of metal 3D printing, specifically using aluminum alloys like AlSi10Mg and A7075, represents a significant leap forward in steering knuckle design and manufacturing. It moves beyond the incremental improvements offered by traditional methods, enabling truly optimized, lightweight components that directly contribute to safer, more efficient, and better-performing vehicles.
Core Applications: Where are 3D Printed Steering Knuckles Driving Innovation?
The unique advantages offered by metal additive manufacturing, particularly lightweighting and design freedom, make 3D printed aluminum steering knuckles a compelling solution across various segments of the automotive industry. While not yet ubiquitous in mass-market volume production due to cost considerations, their application is rapidly expanding in areas where performance, customization, and rapid development are paramount. These applications showcase the tangible benefits and highlight the trajectory for broader adoption as the technology matures and costs decrease. Key areas benefiting from this innovation include performance vehicles, electric vehicles (EVs), prototyping and development, and niche or custom vehicle builds. Understanding these applications is crucial for automotive engineers exploring advanced materials, procurement managers seeking innovative component suppliers, and tier 1 automotive suppliers aiming to integrate cutting-edge technology.
1. High-Performance Vehicles and Motorsport:
This is arguably the most prominent and impactful application area for 3D printed steering knuckles today. In motorsport and high-performance road cars, the pursuit of lower lap times, improved handling dynamics, and competitive advantage justifies the higher initial cost of AM components.
- Extreme Lightweighting: As mentioned earlier, reducing unsprung mass is critical for handling. Topology-optimized, 3D printed aluminum knuckles can shave significant weight compared to even the most refined forged aluminum parts, leading to faster suspension response, better tire contact with the road, improved grip during cornering, and more effective braking. Teams and manufacturers can gain a measurable performance edge.
- Customized Geometry: AM allows for tailoring the knuckle geometry to specific suspension kinematics, tire sizes, brake packages, and aerodynamic requirements. Suspension pick-up points can be precisely located for optimal camber gain, roll center height, and steering axis inclination (SAI), tuning the vehicle’s handling characteristics with a level of precision difficult to achieve with tooling-based methods.
- Integrated Features: Cooling ducts for brakes can be integrated directly into the knuckle design, improving brake performance and reducing fade during demanding track use. Sensor mounting points can also be seamlessly incorporated.
- Rapid Iteration: Motorsport teams operate under tight deadlines. AM enables rapid design changes and production of updated knuckles between races or testing sessions, allowing for continuous performance refinement. A steering knuckle manufacturer specializing in AM can deliver prototypes in days rather than weeks or months.
Example Scenario: A GT racing team needs to optimize the front suspension geometry for a specific track. Using topology optimization software and LPBF printing with a high-strength aluminum alloy like A7075, they design and manufacture knuckles that are 40% lighter than the previous forged versions and feature revised pick-up points. This results in improved tire grip and reduced lap times.
2. Electric Vehicles (EVs): The Range and Efficiency Imperative
The transition to electric mobility presents unique engineering challenges, primarily centered around battery weight and maximizing driving range. Lightweighting is no longer just about performance; it’s fundamental to the vehicle’s viability.
- Offsetting Battery Weight: Batteries are heavy. Reducing mass elsewhere in the vehicle, especially unsprung mass, helps compensate for the battery pack’s weight, improving overall efficiency and extending range. Lighter knuckles contribute directly to lowering the vehicle’s energy consumption (kWh/100km or kWh/mile).
- Improved Dynamics: While range is key, EVs also benefit from improved handling. Instant torque from electric motors requires a suspension system that can manage forces effectively. Lighter knuckles enhance the dynamic responsiveness of EVs, contributing to a more engaging driving experience.
- Packaging Constraints: EV platforms often have unique packaging requirements due to battery placement and electric motor integration. The design freedom of AM allows knuckles to be shaped around these constraints more easily than traditional components.
- NVH Considerations: Lighter components can sometimes alter noise, vibration, and harshness (NVH) characteristics. AM allows for designs that are optimized not just for weight and strength, but potentially for specific damping or frequency response characteristics, helping to manage NVH in quiet EV powertrains.
Example Scenario: An EV manufacturer is developing a new platform and aims for a class-leading range. By employing 3D printed AlSi10Mg steering knuckles (optimized via topology studies) across the platform, they achieve a significant reduction in unsprung mass, contributing to a measurable increase in the vehicle’s EPA or WLTP range rating compared to using conventional cast aluminum knuckles. This becomes a key selling point promoted by their automotive component distributors.
3. Prototyping and Vehicle Development:
Before committing to expensive tooling for mass production (casting dies or forging molds), automotive manufacturers undergo rigorous prototyping and testing phases. AM is an invaluable tool during this stage.
- Accelerated Development Cycles: Creating functional metal prototypes of steering knuckles using AM is significantly faster than traditional prototyping methods involving extensive machining or temporary tooling. Designs can be physically realized and tested on prototype vehicles within days or weeks.
- Cost-Effective Iteration: If testing reveals the need for design modifications (e.g., strengthening a specific area, adjusting a suspension point), changes can be made in the CAD model, and a new prototype can be printed relatively quickly and affordably. This avoids the sunk costs associated with modifying or scrapping hard tooling.
- Early Performance Validation: AM prototypes, especially those printed in the target material (like AlSi10Mg or A7075), allow engineers to validate performance characteristics (stiffness, clearance checks, initial fatigue testing) early in the development process, reducing risks before committing to mass production methods.
- Testing Multiple Design Variants: AM makes it feasible to produce and test several design variations of a steering knuckle concurrently, allowing engineers to compare different optimization strategies or kinematic solutions empirically.
Example Scenario: An automotive OEM is developing a new SUV platform. During the chassis development phase, they use LPBF to print several iterations of the front steering knuckle in AlSi10Mg, testing different rib patterns and wall thicknesses derived from topology optimization. This allows them to finalize a lightweight yet robust design for mass production (which might eventually be cast or forged, but based on the AM-validated optimal geometry) much faster and with higher confidence. They partner with an AM service provider capable of delivering high-quality prototypes quickly.
4. Niche Vehicles, Custom Builds, and Aftermarket:
For low-volume production runs, custom vehicles, or the performance aftermarket, the economics of traditional tooling are often prohibitive. AM provides a viable manufacturing solution.
- Tooling-Free Production: AM eliminates the need for expensive molds or dies, making it economically feasible to produce small batches (dozens or hundreds) of steering knuckles for limited edition vehicles, bespoke builds, or aftermarket upgrades.
- High Degree of Customization: AM allows for unique, customized knuckle designs tailored to specific vehicle modifications, such as accommodating larger brakes, different suspension setups, or unusual wheel offsets, catering to the needs of specialty vehicle manufacturers and the performance tuning market.
- Legacy Part Replacement: For classic or rare vehicles where original tooling no longer exists, AM offers a way to reverse engineer and remanufacture obsolete steering knuckles, keeping these vehicles on the road.
Example Scenario: A company specializing in restoring and upgrading classic sports cars needs stronger, lighter steering knuckles to handle modern tire grip and engine power upgrades. Original parts are unavailable or inadequate. They partner with a metal AM specialist like Met3dp to design and produce topology-optimized A7075 knuckles in small batches, offering them as a premium upgrade package to their clientele. This positions them as an innovative supplier in the restoration market.
Implications for Tier 1 Automotive Suppliers and Distributors:
The rise of AM in these applications signals a shift in the automotive supply chain. Tier 1 suppliers and automotive component distributors need to recognize the potential of AM:
- New Service Offerings: Suppliers can incorporate metal AM capabilities to offer rapid prototyping, low-volume production, and highly optimized lightweight components.
- Competitive Advantage: Offering AM-produced parts can differentiate a supplier in the market, attracting clients looking for cutting-edge solutions, particularly in the performance and EV sectors.
- Adapting Sourcing Strategies: Procurement managers within these companies need to identify and partner with reliable poskytovatelé služeb kovového 3D tisku a high-quality aluminum powder suppliers like Met3dp, who possess the necessary expertise, technology (like advanced LPBF printers), and quality control systems (e.g., rigorous powder analysis, process monitoring) essential for producing safety-critical automotive parts. Building relationships with wholesale 3D printed parts providers becomes strategically important.
In conclusion, the applications of 3D printed aluminum steering knuckles span critical areas of the automotive industry, from the demanding world of motorsport to the efficiency-driven EV market and the flexible needs of prototyping and niche manufacturing. As the technology continues to advance and scale, its role in shaping the future of automotive components will only grow, making it essential for stakeholders across the value chain to understand and engage with its capabilities.
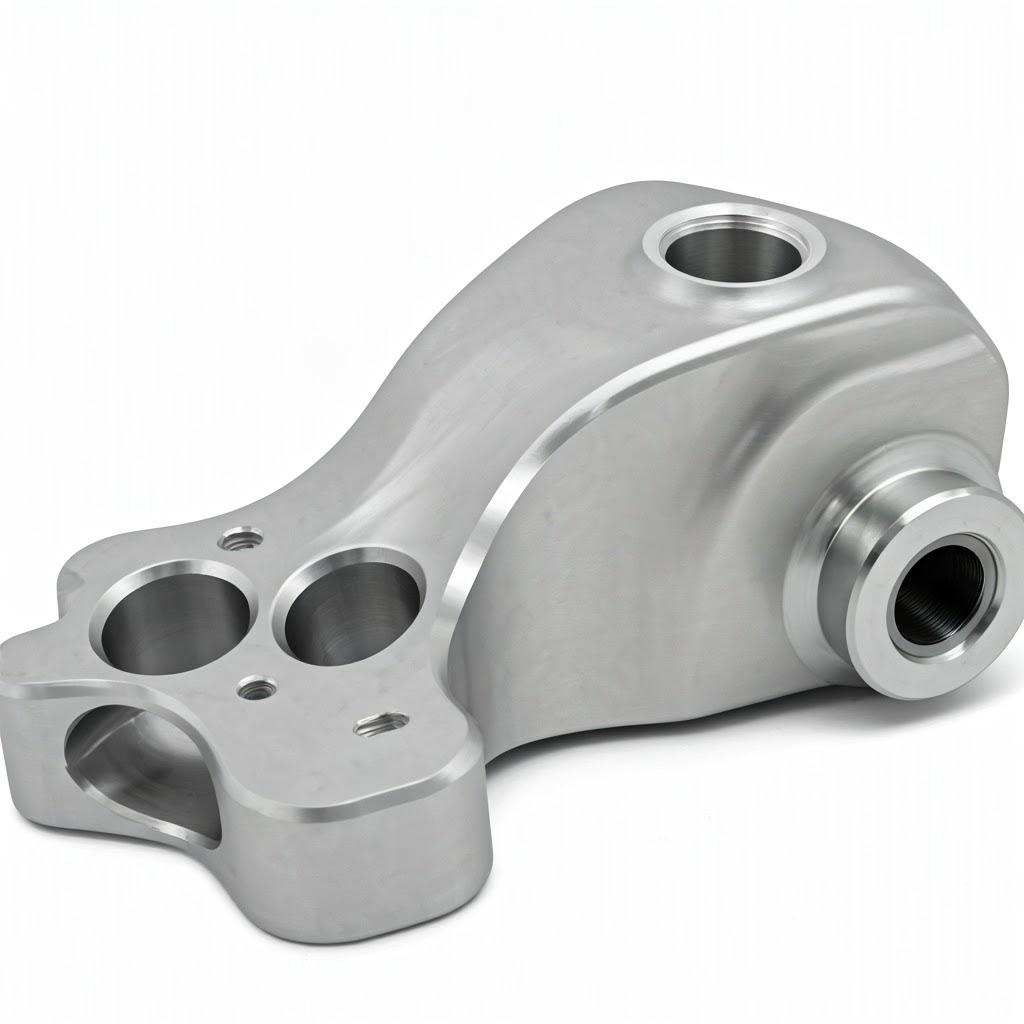
The AM Advantage: Why Choose Metal 3D Printing for Steering Knuckle Production?
While traditional methods like casting and forging have long served the automotive industry for producing steering knuckles, metal additive manufacturing (AM) presents a compelling suite of advantages that directly address the evolving demands for higher performance, increased efficiency, and faster development cycles. Opting for AM, specifically Laser Powder Bed Fusion (LPBF), for steering knuckle production is not merely about adopting a novel technology; it’s about leveraging distinct benefits that translate into tangible improvements in the final product and the manufacturing process. Understanding these advantages is crucial for engineers designing next-generation vehicles, procurement managers evaluating sourcing options, and automotive component distributors seeking innovative products.
Let’s compare AM with traditional casting and forging for steering knuckles and highlight the key reasons why AM stands out:
1. Unparalleled Design Freedom & Complexity:
- Traditional Methods: Casting requires drafts angles for mold removal, limitations on internal features, and relatively uniform wall thicknesses. Forging shapes metal under pressure, restricting designs to what can be practically formed between dies, often resulting in simpler, bulkier geometries requiring extensive post-machining.
- AM (LPBF): Builds parts layer by layer, freeing designers from many traditional constraints. This enables:
- Složité geometrie: Intricate internal lattices, hollow structures, and organically shaped, topology-optimized forms are possible.
- Negative Draft Angles: Features that would be impossible to demold in casting can be easily created.
- Undercuts & Internal Channels: Complex internal passages for cooling, hydraulics, or sensor integration can be designed directly into the part.
- Thin Walls & Fine Features: Greater precision in creating thin structural elements and fine details, contributing to weight reduction.
- Advantage: AM allows engineers to design the optimal steering knuckle based on performance requirements (stress, stiffness, load paths) rather than being limited by manufacturing process constraints. This leads to designs that are truly optimized for function and weight.
2. Significant Lightweighting through Topology Optimization:
- Traditional Methods: Weight reduction typically involves switching to lighter materials (e.g., aluminum instead of iron) or extensive, often iterative, machining after casting/forging. Optimization is often based on engineering intuition and standard Finite Element Analysis (FEA), constrained by the manufacturing process.
- AM (LPBF): Perfectly suited for optimalizace topologie. This computational process uses FEA to simulate loads on the part and then intelligently removes material from non-critical areas, leaving behind an optimized load-bearing structure.
- Organic Structures: Results often resemble natural bone structures – highly efficient and lightweight.
- Targeted Stiffness: Material is placed exactly where needed to achieve desired stiffness and strength targets.
- Dramatic Weight Savings: As previously mentioned, weight reductions of 30-50% or more are achievable compared to traditional designs, directly impacting unsprung mass and vehicle performance/efficiency.
- Advantage: AM enables mathematically optimized lightweighting far beyond what’s practical with casting or forging, directly addressing a key automotive industry driver. A steering knuckle manufacturer using AM can offer significantly lighter parts without compromising safety.
3. Part Consolidation:
- Traditional Methods: Complex assemblies often require multiple individual components (castings, forgings, machined parts, brackets) to be manufactured separately and then joined together (welding, bolting). Each joint adds weight, potential failure points, and assembly time/cost.
- AM (LPBF): The design freedom allows engineers to consolidate multiple parts into a single, monolithic component. For example, mounting brackets for sensors or brake lines, which might normally be separate pieces, can be integrated directly into the 3D printed steering knuckle design.
- Reduced Part Count: Simplifies inventory, logistics, and assembly.
- Increased Structural Integrity: Eliminates joints, which are often stress concentration points.
- Reduced Weight: Eliminates fasteners and overlapping material at joints.
- Simplified Supply Chain: Fewer individual components to source and manage.
- Advantage: AM simplifies the overall system, potentially reducing manufacturing and assembly costs, improving reliability, and further contributing to weight reduction. This is a significant benefit for automotive parts manufacturers looking to streamline production.
4. Rapid Prototyping and Accelerated Development:
- Traditional Methods: Creating prototypes often involves expensive temporary tooling (soft molds) or extensive CNC machining from billet, both taking considerable time (weeks or months) and cost. Design iterations require modifying or recreating this tooling/process.
- AM (LPBF): Prototypes can be printed directly from CAD data in a matter of days.
- Rychlost: Drastically reduces the time from design concept to physical, testable part.
- Cost-Effective Iteration: Changes are made in CAD, and a new part is printed without tooling modification costs. Multiple design variants can be tested quickly.
- Functional Prototypes: Prototypes can be printed in the final intended material (e.g., AlSi10Mg, A7075), allowing for realistic functional testing early in the development cycle.
- Advantage: AM significantly shortens the vehicle development timeline, allows for more thorough design exploration and validation, and reduces the financial risks associated with committing to hard tooling too early. This agility is invaluable in the fast-paced automotive sector.
5. Elimination/Reduction of Tooling Costs:
- Traditional Methods: Require substantial upfront investment in dedicated tooling:
- Obsazení: Complex molds and cores.
- Kování: Hardened steel dies.
- Tooling costs can run into tens or hundreds of thousands of dollars, making it uneconomical for low volumes or frequent design changes. Tooling also requires maintenance and storage.
- AM (LPBF): A “tooling-less” process. The complexity is embedded in the digital design file and the AM machine itself.
- No Dedicated Tooling: Eliminates the high upfront investment and long lead times associated with creating molds or dies.
- Economic for Low-to-Medium Volumes: Makes production of niche parts, custom components, prototypes, and small series runs economically viable.
- Flexibilita designu: Designs can be changed without any tooling cost penalty.
- Advantage: AM drastically lowers the barrier to entry for producing custom or low-volume steering knuckles and makes design changes financially feasible throughout the product lifecycle. This benefits wholesale 3D printed parts suppliers and their customers requiring flexibility.
Comparative Summary Table:
Vlastnosti | Metal Additive Manufacturing (LPBF) | Tradiční casting | Tradiční kování |
---|---|---|---|
Svoboda designu | Very High (complex geometries, internal features) | Moderate (draft angles, wall thickness) | Low (simpler shapes, requires machining) |
Odlehčení | Excellent (Topology optimization, lattices) | Moderate (material choice, basic ribs) | Limited (material choice, machining) |
Konsolidace částí | High Potential | Omezený | Very Limited |
Prototyping Speed | Very Fast (Days) | Slow (Weeks/Months – tooling/machining) | Slow (Weeks/Months – tooling/machining) |
Iteration Cost | Low (CAD changes) | High (Tooling modification/rework) | Very High (New dies/modification) |
Náklady na nástroje | None (Digital) | High (Molds, cores) | Very High (Dies) |
Volume Economics | Best for Low-to-Medium Volumes, Prototypes | Best for High Volumes | Best for Very High Volumes (simpler parts) |
Materiálový odpad | Low (Powder recycling) | Moderate (Runners, gates) | Moderate (Flash, machining scrap) |
Min. Feature Size | Vysoké rozlišení | Limited by casting process | Limited by forging process & machining |
Export do archů
Considering Met3dp’s Role:
Choosing AM requires partnering with a capable provider. Met3dp, with its dual expertise in high-performance 3D tisk z kovu systems and premium metal powder production, embodies the AM advantage. Their advanced printers are designed for the accuracy and reliability needed for critical components like steering knuckles. Furthermore, their in-house production of optimized aluminum powders (like AlSi10Mg and potentially custom variants) ensures the material quality essential for achieving the desired mechanical properties and performance benefits promised by AM. Partnering with a vertically integrated company like Met3dp streamlines the process, from material selection consultancy to final part production, ensuring that the full advantages of AM are realized.
In conclusion, while casting and forging remain relevant for certain high-volume, established designs, metal AM offers transformative advantages in design freedom, lightweighting, part consolidation, development speed, and economic flexibility, particularly for aluminum steering knuckles in performance, EV, and prototyping applications. These benefits provide compelling reasons for automotive manufacturers and suppliers to integrate AM into their production strategies.
Material Focus: Selecting Optimal Aluminum Alloys (AlSi10Mg & A7075) for Steering Knuckles
The success of a 3D printed steering knuckle hinges critically on the choice of material. While metal additive manufacturing opens the door to a wide range of alloys, aluminum alloys stand out for this application due to their inherent low density and good mechanical properties. Within the aluminum family, two alloys are frequently considered and utilized for demanding structural components like steering knuckles via Laser Powder Bed Fusion (LPBF): AlSi 10Mg a A7075 (often an AM-processed variant). Understanding the specific characteristics, strengths, weaknesses, and processing considerations of these materials is vital for engineers designing the part and for procurement managers sourcing from aluminum powder suppliers or AM service providers.
Why Aluminum? The Lightweighting Imperative
Aluminum’s primary appeal is its density, roughly one-third that of steel, while still offering respectable strength, especially in alloyed forms and after appropriate heat treatment. This low density is the cornerstone of lightweighting efforts aimed at reducing unsprung mass, improving fuel/energy efficiency, and enhancing vehicle dynamics. Aluminum alloys also generally offer good corrosion resistance and machinability.
AlSi10Mg: The Workhorse AM Aluminum Alloy
AlSi10Mg is one of the most common and well-understood aluminum alloys used in metal AM, particularly LPBF. It’s essentially a casting alloy adapted for additive processes. Its composition typically includes ~9-11% Silicon (Si) and 0.2-0.45% Magnesium (Mg).
- Key Properties & Characteristics:
- Excellent Processability: AlSi10Mg behaves very well during the LPBF process. The silicon content improves fluidity in the melt pool and reduces the solidification range, minimizing the risk of hot cracking during the rapid heating and cooling cycles inherent to AM. This makes it relatively easy to print dense, high-quality parts.
- Dobrý poměr pevnosti a hmotnosti: In the as-printed state, it exhibits moderate strength. However, it responds well to heat treatment (typically a T6 cycle: solution heat treatment followed by artificial aging). Post-T6 treatment, AlSi10Mg achieves mechanical properties (yield strength, ultimate tensile strength) comparable to or exceeding those of common A356/A357 casting alloys.
- Dobrá odolnost proti korozi: Exhibits good resistance to atmospheric corrosion.
- Svařitelnost: Can be welded, although specific procedures are recommended.
- Fine Microstructure: The rapid solidification during LPBF results in a very fine dendritic microstructure, which contributes positively to its mechanical properties compared to the same alloy in cast form.
- Strengths for Steering Knuckles:
- Osvědčené výsledky: Widely used in AM for various applications, including automotive prototypes and some functional parts. Extensive research and data are available on its properties and processing.
- Reliable Printing: Its good processability leads to more predictable and repeatable results, crucial for quality control in series production.
- Good Balance of Properties: Offers a solid combination of strength, ductility (especially after stress relief), and corrosion resistance suitable for many automotive chassis applications after appropriate heat treatment.
- Efektivita nákladů: Generally more cost-effective (powder cost and printing ease) compared to higher-strength alloys like AM-processed A7075.
- Weaknesses/Considerations:
- Lower Absolute Strength: Compared to high-strength aluminum alloys like the 7xxx series (e.g., A7075) or steels, its absolute strength and fatigue limits are lower. Designs may need to be slightly bulkier (though still lighter than steel) to compensate in highly stressed areas compared to what might be achievable with A7075.
- Požadováno tepelné zpracování: To achieve optimal mechanical properties (T6 condition), a post-printing heat treatment process is necessary, adding time and cost. As-printed parts have lower strength and ductility.
- Typical LPBF Processing & Properties (Post T6 Heat Treatment): (Note: Values are approximate and depend heavily on specific powder quality, machine parameters, build orientation, and heat treatment specifics.) | Property | Typical Value Range (AlSi10Mg T6) | Unit | | :———————– | :——————————– | :———— | | Density | ~2.67 | g/cm³ | | Yield Strength (Rp0.2) | 230 – 300 | MPa | | Ultimate Tensile Strength| 330 – 430 | MPa | | Elongation at Break | 6 – 15 | % | | Young’s Modulus | ~70 – 75 | GPa | | Hardness | 100 – 120 | HV | | Relative Density Achieved| > 99.5 | % |
A7075: The High-Strength Contender
A7075 is a well-known high-strength wrought aluminum alloy (part of the 7xxx series, typically alloyed with Zinc, Magnesium, and Copper). It’s prized in aerospace and performance applications for its exceptional strength-to-weight ratio, significantly exceeding that of AlSi10Mg or standard casting alloys. Adapting it for LPBF has been challenging but is becoming increasingly viable.
- Key Properties & Characteristics:
- Very High Strength: Offers significantly higher yield and ultimate tensile strength compared to AlSi10Mg, approaching the strength of some steels but at a fraction of the weight. This is its primary advantage.
- Good Fatigue Strength: Generally exhibits good resistance to fatigue failure under cyclic loading, crucial for components like steering knuckles.
- Challenging Processability: A7075 is notoriously difficult to process using fusion-based AM methods like LPBF. Its wide solidification range and susceptibility to vaporization of low-boiling-point elements (like Zinc) make it prone to defects such as solidification cracking (hot tearing) and porosity if not processed under carefully controlled conditions with optimized parameters and potentially modified alloy compositions specifically for AM.
- Heat Treatment Complexity: Requires specific, often multi-stage, heat treatments (like T6 or T7x temper) to achieve its full strength potential. These treatments must be carefully controlled to avoid issues like distortion or property degradation.
- Nižší odolnost proti korozi: Generally less resistant to corrosion than AlSi10Mg, especially stress corrosion cracking (SCC), although specific tempers (like T73) improve SCC resistance at a slight cost to peak strength. Surface treatments are often necessary.
- Strengths for Steering Knuckles:
- Maximum Lightweighting Potential: Its superior strength allows for more aggressive topology optimization and thinner structural sections, potentially leading to the absolute lightest possible aluminum knuckle design for a given load case. Ideal for motorsport and extreme performance applications.
- Vysoký výkon: Enables components capable of handling very high loads and stresses.
- Weaknesses/Considerations:
- Printing Difficulty & Quality Control: Requires specialized expertise, finely tuned LPBF parameters, and potentially modified AM-specific 7xxx series powder compositions to reliably produce dense, crack-free parts. Quality control and non-destructive testing (NDT) are critical.
- Náklady: A7075 powder is typically more expensive than AlSi10Mg, and the more complex printing and heat treatment processes add further cost.
- Potential for Defects: Higher risk of printing defects if process control is not stringent.
- Corrosion Management: May require protective coatings or specific temper selection depending on the operating environment.
- Typical LPBF Processing & Properties (Heat Treated – e.g., T6): (Note: Values are highly dependent on achieving a high-quality, defect-free print and proper heat treatment. Processability is key.) | Property | Typical Target Value Range (AM A7075 T6) | Unit | | :———————– | :————————————— | :———— | | Density | ~2.81 | g/cm³ | | Yield Strength (Rp0.2) | 450 – 520 | MPa | | Ultimate Tensile Strength| 520 – 590 | MPa | | Elongation at Break | 4 – 10 | % | | Young’s Modulus | ~71 – 73 | GPa | | Hardness | 150 – 180 | HV | | Relative Density Achieved| > 99.0 (Requires expert processing) | % |
Making the Choice: AlSi10Mg vs. A7075
The selection between AlSi10Mg and A7075 for a 3D printed steering knuckle depends heavily on the specific application requirements and constraints:
- Choose AlSi10Mg if:
- Cost is a major driver.
- Proven process reliability and predictability are paramount.
- Good, but not extreme, strength and lightweighting are sufficient.
- Faster development/prototyping iterations are needed using a well-established material.
- Corrosion resistance is a significant factor without relying heavily on coatings.
- Choose A7075 (or AM-specific variant) if:
- Absolute maximum strength-to-weight ratio is the primary goal (e.g., motorsport, aerospace-level performance).
- The highest possible lightweighting is required.
- The budget allows for higher material and processing costs.
- Partnering with an AM provider with demonstrated expertise in processing high-strength, crack-sensitive aluminum alloys is possible.
- Appropriate post-processing (heat treatment, surface protection) and rigorous quality control are planned.
The Importance of Powder Quality and Sourcing
Regardless of the alloy chosen, the quality of the metal powder is fundamental to the success of the LPBF process and the final part’s integrity. Key powder characteristics include:
- Sféricita: Highly spherical powder particles ensure good flowability in the printer’s recoating system and uniform packing density on the powder bed, leading to more consistent melting and denser parts.
- Distribuce velikosti částic (PSD): A controlled PSD is crucial for achieving high packing density and predictable melting behavior. Fines can affect flowability and pose safety hazards, while oversized particles may not melt completely.
- Chemical Purity: Strict adherence to the specified alloy composition is essential. Contaminants can drastically affect mechanical properties and processability. Low oxygen and moisture content are particularly important.
- Absence of Satellites: Small particles attached to larger ones (satellites) can impede flowability and packing.
This is where specialized metal powder suppliers like Met3dp play a critical role. Met3dp employs advanced production techniques like Vacuum Induction Melting Gas Atomization (VIGA) a Proces plazmové rotující elektrody (PREP). These methods are designed to produce powders with:
- Vysoká sféricita: Ensuring excellent flowability.
- Controlled PSD: Tailored for optimal performance in LPBF systems.
- Vysoká čistota: Minimizing contaminants like oxygen and nitrogen.
- Low Satellite Content: Further enhancing powder performance.
By sourcing high-quality, batch-consistent AlSi10Mg or specialized A7075 powders from a reputable aluminum powder distributor or manufacturer like Met3dp, engineers and procurement managers can significantly increase the likelihood of successfully printing high-integrity, high-performance steering knuckles that meet demanding automotive specifications. Met3dp’s capability extends beyond standard alloys, including research into innovative compositions like TiAl, TiNbZr, and others, showcasing their deep materials expertise relevant for future automotive advancements available through their product offerings.
In summary, selecting the right aluminum alloy—balancing the reliable processability of AlSi10Mg against the high-strength potential of A7075—and ensuring the use of high-quality, AM-optimized powder are critical first steps in leveraging 3D printing for advanced, lightweight automotive steering knuckles.
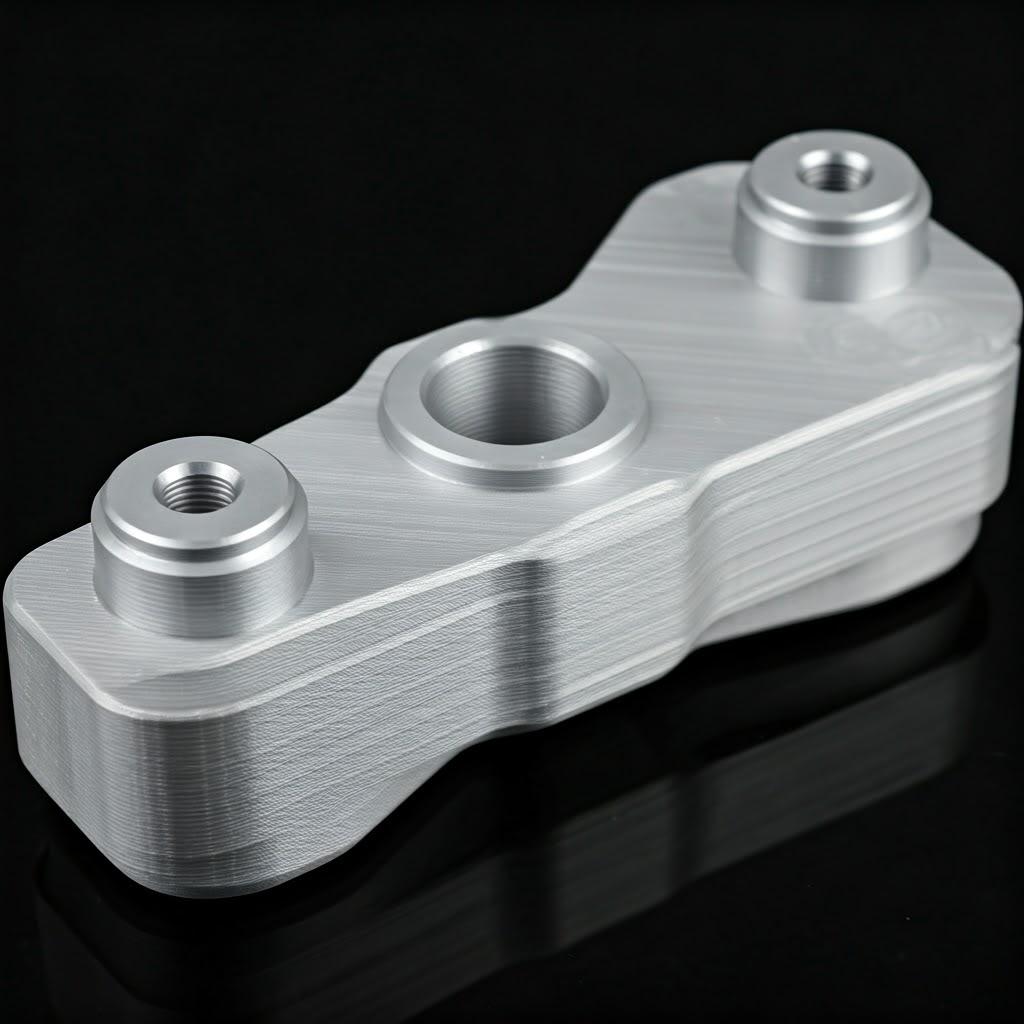
Design for Additive Manufacturing (DfAM): Optimizing Steering Knuckles for 3D Printing
Simply taking a design intended for casting or forging and sending it to a metal 3D printer is a recipe for disappointment. To truly unlock the potential of additive manufacturing for components like steering knuckles, engineers must embrace Design pro aditivní výrobu (DfAM). DfAM is not just a suggestion; it’s a fundamental shift in design philosophy that considers the unique capabilities and constraints of the layer-by-layer building process right from the concept stage. Applying DfAM principles is essential to maximize lightweighting, ensure structural integrity, minimize printing time and cost, and reduce post-processing efforts. For automotive parts manufacturers transitioning towards AM, mastering DfAM is crucial for producing efficient and reliable components.
1. Topology Optimization: Designing by Load Path
This is often the starting point and the most visually striking aspect of DfAM for structural parts. Instead of starting with a solid block and removing material (subtractive thinking) or filling a predefined mold shape (casting thinking), topology optimization works differently:
- Define the Space: Start with the maximum allowable design volume for the steering knuckle, including all connection points (wheel hub, control arms, tie rod, brake caliper mounts) fixed in space.
- Apply Loads and Constraints: Define all the expected load cases the knuckle will experience during its operational life (cornering forces, braking torque, impacts, suspension travel limits) using Finite Element Analysis (FEA). Define constraints such as keep-out zones and fixed mounting points.
- Set Optimization Goals: Typically, the goal is to minimize mass (or volume) while satisfying constraints on maximum allowable stress and deflection (stiffness).
- Run the Algorithm: Specialized software iteratively removes material from areas experiencing low stress, leaving material only where it’s needed to carry the defined loads efficiently.
- Interpret and Refine: The raw output of topology optimization is often an organic, skeletal mesh that needs interpretation and refinement by a designer. This involves smoothing surfaces, ensuring manufacturability (considering AM constraints discussed below), and potentially running further analysis loops.
Steering Knuckle Specifics: Pro a topology optimization steering knuckle project, accurately defining the multiple complex load cases (combinations of braking, cornering, and bump loads) is critical. The optimization should aim to maintain stiffness at critical mounting points while drastically reducing material elsewhere. The resulting designs often look radically different from their traditional counterparts, showcasing efficient load paths.
2. Lattice Structures and Infill Strategies
Beyond removing bulk material, DfAM allows for the strategic use of internal lattice structures:
- Further Lightweighting: Replacing solid sections with carefully designed internal lattices (e.g., gyroids, diamond, honeycomb structures) can further reduce weight while maintaining significant structural support and stiffness.
- Vlastnosti na míru: Different lattice types and densities can be used to tune specific properties like energy absorption (for crashworthiness) or vibration damping.
- Tepelný management: Lattices can potentially improve heat dissipation, although this is less common as a primary driver for knuckles.
- Výzvy: Designing and validating the performance of lattice structures requires advanced software and simulation capabilities. Ensuring powder removal from complex internal lattices after printing is also a key consideration.
Steering Knuckle Specifics: Lattices might be selectively applied in lower-stress regions of the knuckle identified during topology optimization, providing local stiffness with minimal mass penalty.
3. Designing for Self-Support and Support Structure Strategy
LPBF requires support structures for overhanging features and to anchor the part to the build plate, preventing warping. However, supports add material cost, printing time, and significant post-processing effort for removal, potentially damaging the part surface. Effective DfAM aims to minimize reliance on supports:
- Orientation Strategy: Choosing the optimal orientation of the steering knuckle on the build plate is crucial. This impacts the amount and location of required supports, surface quality of different faces, residual stress distribution, and build time. Often, multiple orientations are evaluated digitally.
- Self-Supporting Angles: Designing overhangs below a critical angle (typically around 45 degrees from the horizontal for aluminum alloys like AlSi10Mg, though machine and parameter dependent) allows them to be printed without supports. Designers can use chamfers or fillets instead of sharp horizontal overhangs.
- Internal Channels: Designing internal channels with self-supporting shapes (e.g., diamond or teardrop cross-sections instead of circular) can eliminate the need for difficult-to-remove internal supports.
- Support Optimization: Where supports are unavoidable, DfAM involves designing them for easy removal (e.g., using minimal contact points, specific perforation patterns) and placing them on non-critical surfaces where removal marks are acceptable or will be machined away later. Specialized software can help generate optimized support structures.
Steering Knuckle Specifics: The complex geometry of a knuckle with multiple arms and mounting points makes orientation and support strategy critical. Minimizing supports on functional interfaces (bearing bores, mounting faces) is essential to reduce post-machining needs.
4. Managing Thermal Stress and Warpage
The intense localized heating and rapid cooling during LPBF create significant thermal gradients and residual stresses within the part. If not managed, these stresses can cause:
- Warpage/Distortion: The part curling up or detaching from the build plate during printing.
- Cracking: Particularly in sensitive alloys like A7075.
- Reduced Mechanical Performance: High residual stress can negatively impact fatigue life.
DfAM Mitigation Strategies: * Filleting: Avoiding sharp internal corners and using generous fillets reduces stress concentrations. * Orientace: Building tall, thin sections vertically can sometimes reduce stress compared to building them horizontally. * Thermal Compensation: Advanced techniques might involve subtly adjusting the CAD model to compensate for predicted shrinkage or distortion. * Strategic Material Distribution: Avoiding large, abrupt changes in cross-sectional area can help manage heat buildup and cooling rates. * Robust Anchoring: Ensuring sufficient support connecting the part to the build plate, especially during the initial layers.
Steering Knuckle Specifics: The varying thicknesses and complex shape make knuckles susceptible to thermal stresses. Careful filleting at junctions between arms and the main body, along with optimized orientation and support, is vital.
5. Minimum Wall Thickness and Feature Resolution
LPBF has limitations on how thin a wall or how small a feature can be reliably printed.
- Tloušťka stěny: For aluminum alloys like AlSi10Mg and A7075, minimum achievable wall thickness is typically in the range of 0.4mm to 1.0mm, depending on the machine, parameters, and wall height/orientation. Designers must ensure structurally relevant sections adhere to practical minimums.
- Feature Resolution: The laser spot size and powder particle size limit the resolution of fine details, small holes, and sharp edges. Very small features might not resolve accurately or could be fused shut.
Steering Knuckle Specifics: While topology optimization might suggest extremely thin sections, designers must enforce manufacturable minimum thicknesses. Small non-critical holes might be better drilled during post-processing than printed directly if high accuracy is needed.
6. Designing for Post-Processing
A crucial DfAM aspect often overlooked is designing the part with subsequent post-processing steps in mind:
- Přídavky na obrábění: Surfaces requiring high precision or specific finishes (e.g., bearing bores, brake caliper mounts, control arm interfaces) should be designed with extra material (“machining stock” or “offset”) that will be removed during CNC machining.
- Workholding: Consider how the complex shape of the knuckle will be securely held during machining or other post-processing steps. Adding temporary fixtures or reference features that can be easily removed later might be necessary.
- Support Access: Ensure support structures are located where they can be physically accessed for removal tools without damaging the final part surface.
- Powder Removal: For parts with internal channels or lattices, design includes features or access points to facilitate the removal of unfused powder after printing.
Steering Knuckle Specifics: Adding 0.5mm to 1.5mm of stock on critical mating surfaces and bearing bores is common practice. Ensuring clear access for tools to remove supports around suspension pick-up points is essential.
Partnering for DfAM Expertise:
Successfully implementing DfAM, especially for safety-critical parts like steering knuckles, requires expertise. Partnering with an experienced AM service provider like Met3dp offers significant advantages. Met3dp’s engineers understand the nuances of LPBF with aluminum alloys and can collaborate on design optimization, support strategy, orientation analysis, and ensuring the design is truly manufacturable. Their experience with automotive component production provides valuable insights into translating performance requirements into optimized, printable designs. Adhering to established additive manufacturing design guidelines while leveraging the unique possibilities of the technology is key to success.
In essence, DfAM transforms the design process from conforming to manufacturing limitations to leveraging manufacturing possibilities. For 3D printed aluminum steering knuckles, it is the critical enabler for achieving maximum lightweighting, performance, and cost-effectiveness.
Achieving Precision: Tolerance, Surface Finish, and Dimensional Accuracy in 3D Printed Knuckles
While metal 3D printing offers unprecedented design freedom, a common question from engineers and procurement managers, especially those accustomed to the high precision of CNC machining, concerns the achievable levels of tolerance, surface finish, and overall dimensional accuracy. For a component like a steering knuckle, where precise interfaces with bearings, suspension arms, and brakes are critical, understanding the inherent precision of Laser Powder Bed Fusion (LPBF) and the factors influencing it is essential. Relying on experienced providers like Met3dp, known for their industry-leading print volume, accuracy and reliability, is key to meeting stringent automotive quality standards.
Typical Dimensional Accuracy and Tolerances in LPBF
LPBF is capable of producing metal parts with good dimensional accuracy, but it’s generally not as precise as multi-axis CNC machining out-of-the-box.
- General Tolerances: For well-calibrated industrial LPBF systems printing aluminum alloys like AlSi10Mg or A7075, typical achievable tolerances for medium-sized parts (like a steering knuckle) are often in the range of:
- ±0.1mm to ±0.3mm for smaller dimensions (e.g., below 100mm).
- ±0.1% to ±0.2% of the nominal dimension for larger features.
- ISO Standards: The accuracy of AM parts can be classified according to standards like ISO/ASTM 52900 series or specific process standards. However, achievable accuracy is highly dependent on the specific machine, material, part geometry, and process control.
- Critical Features: It’s crucial to understand that these general tolerances may not be sufficient for all features on a steering knuckle, particularly bearing bores, precise mounting faces for brake calipers, or tapered holes for ball joints. These features almost always require post-machining to achieve the necessary tight tolerances (often in the range of ±0.01mm to ±0.05mm).
Faktory ovlivňující přesnost:
Achieving the best possible accuracy in LPBF requires careful control over numerous factors:
- Kalibrace stroje: Regular and precise calibration of the printer’s lasers, scanners (galvanometers), and motion systems is fundamental.
- Tepelné účinky: Residual stress, shrinkage during cooling, and thermal expansion/contraction during the build significantly impact final dimensions. Factors like build plate heating, laser scanning strategy, and support structures influence the thermal history.
- Part Orientation: The orientation of the part on the build plate affects how thermal stresses accumulate and can influence the accuracy of different features due to the layer-wise building process (e.g., the “stair-stepping” effect on sloped surfaces).
- Kvalita prášku: Consistent particle size distribution and morphology of the aluminum powder contribute to uniform melting and solidification, impacting accuracy. Sourcing from quality-focused suppliers like Met3dp is beneficial.
- Laser Parameters: Settings like laser power, scan speed, hatch spacing, and layer thickness directly influence the melt pool size and stability, affecting dimensional precision.
- Gas Flow: Proper inert gas flow (typically Argon or Nitrogen) within the build chamber is needed to remove processing by-products and ensure consistent melting conditions. Uneven flow can affect part quality and accuracy.
- Následné zpracování: Stress relief heat treatment can cause slight dimensional changes (shrinkage or growth), which needs to be accounted for. Support removal can also affect nearby surfaces.
Surface Finish (Roughness)
The surface finish of as-printed LPBF parts is inherently rougher than machined surfaces. The texture arises from the partially melted powder particles adhering to the surface and the layer-wise construction.
- Typical Ra Values: Surface roughness (Ra – arithmetic average roughness) for as-printed aluminum alloys typically varies depending on the surface orientation relative to the build direction:
- Up-facing Surfaces (Top): Generally the smoothest, often Ra 5-10 µm.
- Vertical Walls: Moderate roughness, often Ra 8-15 µm.
- Down-facing Surfaces (Supported): Roughest surfaces due to contact with support structures and the nature of overhang formation, often Ra 15-25 µm or higher. Where supports have been removed, the finish can be even rougher and show witness marks.
- Sloped Surfaces: Exhibit a “stair-stepping” effect, with roughness depending on the angle and layer thickness.
- Influencing Factors: Layer thickness (thinner layers generally yield better finish), laser parameters, powder particle size, and contour scanning strategies all impact the final surface roughness.
- Implications for Knuckles: While the general surface finish might be acceptable for non-critical areas, surfaces requiring smooth operation (e.g., bearing seats) or sealing will need post-processing like machining or potentially polishing. Rough surfaces can also act as stress concentrators, potentially impacting fatigue life if not addressed (e.g., through shot peening).
Meeting Automotive Quality Standards
Achieving the required precision for automotive components like steering knuckles involves a combination of controlled printing and targeted post-processing:
- Process Control During Printing: Utilizing high-quality LPBF machines with robust process monitoring and control systems. This includes monitoring melt pool characteristics, laser power, and inert gas atmosphere. Met3dp’s focus on reliable, accurate printing systems aligns with this need. Their expertise in 3D tisk z kovu encompasses understanding and controlling these critical process variables.
- DfAM for Precision: Designing critical features with appropriate machining stock is essential. Tolerances should be clearly specified on drawings, indicating which are achievable “as-printed” and which require secondary operations.
- Targeted Post-Machining: Using multi-axis CNC machining to finish critical interfaces, bores, and mounting surfaces to the required tight tolerances and surface finishes.
- Rigorous Inspection: Employing Coordinate Measuring Machines (CMM) and 3D scanning technology to verify dimensional accuracy against the CAD model and drawing specifications after printing and after final machining. Statistical process control (SPC) may be implemented for series production.
Precision Summary Table:
Vlastnosti | As-Printed LPBF (Aluminum) | Post-Machined LPBF Part | Typical Requirement (Steering Knuckle Critical Feature) |
---|---|---|---|
General Tolerance | ±0.1 to ±0.3 mm or ±0.1-0.2% | Driven by Machining Capability | N/A (Feature Specific) |
Critical Tolerance | Not Typically Achievable | ±0.01 to ±0.05 mm | ±0.01 to ±0.05 mm |
Surface Finish (Ra) | 5 – 25+ µm (Orientation Dependent) | 0.4 – 3.2 µm (or better) | 0.8 – 1.6 µm (Bearing Bore Example) |
Export do archů
In conclusion, while as-printed LPBF parts offer good baseline accuracy, achieving the high precision required for critical features on an aluminum steering knuckle necessitates a combination of expert process control during printing, DfAM principles (especially adding machining stock), and essential post-machining operations. Understanding these capabilities and limitations allows engineers and procurement managers to set realistic expectations and plan the entire manufacturing workflow effectively, ensuring the final component meets stringent automotive quality standards pro rozměrová přesnost a povrchová úprava.
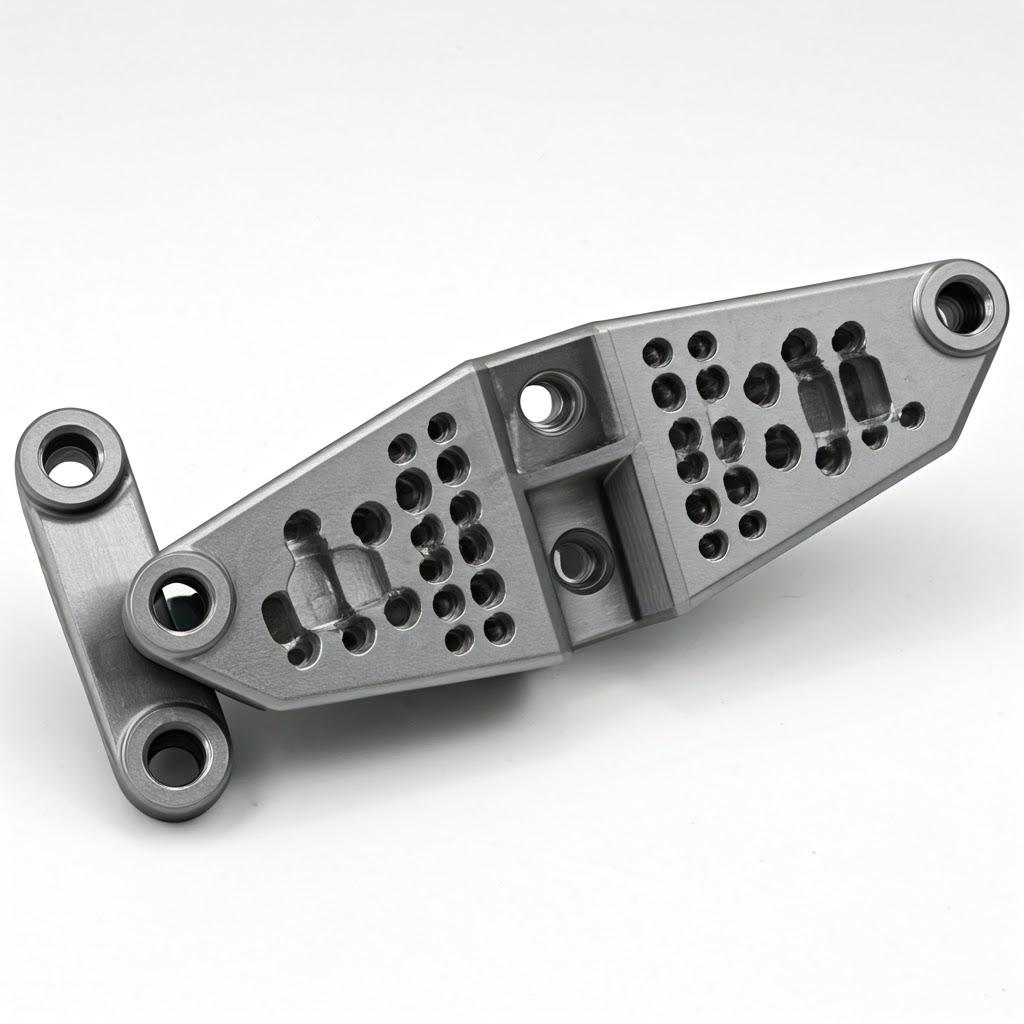
Beyond Printing: Essential Post-Processing Steps for Aluminum Steering Knuckles
The journey of a 3D printed aluminum steering knuckle doesn’t end when the LPBF machine finishes its build cycle. The “green” part, fresh off the build plate, requires several crucial post-processing steps to transform it into a functional, reliable automotive component ready for assembly. These steps are not optional extras; they are integral parts of the additive manufacturing workflow, necessary to relieve stress, remove temporary structures, achieve critical tolerances, enhance surface properties, and ensure overall quality. Understanding these steps is vital for accurately estimating costs, lead times, and ensuring the final part meets performance specifications – key considerations for automotive component wholesale suppliers and their customers.
1. Stress Relief Heat Treatment:
This is arguably the most critical post-processing step for LPBF aluminum parts, especially structural components like steering knuckles made from AlSi10Mg or A7075.
- Why it’s Needed: The rapid heating and cooling inherent in LPBF creates significant residual stresses within the printed part. These internal stresses can:
- Cause distortion or warpage, especially after the part is removed from the build plate.
- Lead to premature failure under load, particularly fatigue cracking.
- Make the part dimensionally unstable during subsequent machining.
- The Process: The printed part, often while still attached to the build plate, is placed in a furnace and subjected to a carefully controlled thermal cycle.
- For AlSi10Mg: A common stress relief cycle might involve heating to around 300°C (572°F) for 1-2 hours, followed by slow cooling. This relaxes internal stresses with minimal impact on the as-printed microstructure and strength.
- For A7075: Stress relief might be integrated as the first stage of a more complex temper heat treatment (like T6 or T7x).
- Výhody: Reduces residual stress, improves dimensional stability for machining, and can slightly improve ductility. It’s essential for predictable performance and preventing distortion.
2. Part Removal from Build Plate:
Once stress-relieved (if done on the plate), the part needs to be separated from the build plate.
- Metody: Typically done using:
- Wire EDM (Electrical Discharge Machining): Precise, minimal force, good for complex or delicate parts.
- Band Sawing: Faster and more common for robust parts, but less precise and requires a flatter base/support structure.
- Machining: Milling or turning off the base.
- Úvahy: The method chosen depends on the part geometry, the required precision of the base surface, and the support structure design. Care must be taken not to damage the part during removal.
3. Support Structure Removal:
The temporary support structures generated during printing must be removed. This can be one of the most labor-intensive and challenging post-processing steps.
- Metody:
- Ruční odstranění: Breaking or cutting supports away using hand tools (pliers, cutters, grinders). Suitable for accessible supports but risks damaging the part surface.
- CNC obrábění: Milling or grinding supports away, more controlled and precise, especially for supports on functional surfaces.
- Wire EDM: Can be used for precise cutting of supports in some cases.
- Výzvy:
- Přístupnost: Supports in complex internal geometries or hard-to-reach areas can be very difficult to remove completely. This reinforces the importance of DfAM to minimize internal supports.
- Surface Marks: Support removal often leaves witness marks or rough patches (“nubs”) on the part surface where they were attached. These may need further finishing.
- Delicate Features: Care must be taken not to break thin walls or delicate features during support removal.
- DfAM Impact: Designing supports with minimal contact points, specific break-away features, and placing them on non-critical surfaces significantly eases removal.
4. Solution Annealing and Aging Heat Treatment (e.g., T6 Temper)
For alloys like AlSi10Mg and A7075 to achieve their optimal strength and hardness, a full heat treatment cycle beyond just stress relief is usually required. The T6 temper is common for aluminum alloys.
- Process (Simplified T6 Example):
- Solution Heat Treatment: Heating the part to a high temperature (e.g., ~520-540°C for AlSi10Mg, ~470-490°C for A7075) for a specific duration to dissolve alloying elements into a solid solution.
- Kalení: Rapidly cooling the part (typically in water) to lock the elements in solution.
- Artificial Aging: Reheating the part to a lower temperature (e.g., ~160-180°C for AlSi10Mg, ~120-150°C for A7075) for several hours to allow controlled precipitation of strengthening phases within the metal matrix.
- Výhody: Significantly increases yield strength, tensile strength, and hardness compared to the as-printed or stress-relieved state. This is crucial for achieving the necessary mechanical properties for a steering knuckle.
- Úvahy: Heat treatment requires precise temperature and time control. Quenching can induce distortion, which might need to be managed through fixtures or subsequent straightening/machining. Different tempers (e.g., T7x for A7075) can offer different balances of strength and stress corrosion cracking resistance. Performing heat treatment AlSi10Mg T6 correctly is vital for part performance.
5. CNC Machining:
As discussed under ‘Precision’, machining is nearly always required for critical features.
- Účel: To achieve tight dimensional tolerances, specific geometric tolerances (e.g., perpendicularity, concentricity), and required surface finishes on functional interfaces.
- Areas Machined: Typically includes:
- Wheel bearing mounting bore.
- Mounting faces for brake caliper and backing plate.
- Tapered holes for control arm and tie rod end ball joints.
- Other critical mating surfaces or interfaces.
- Proces: Utilizes multi-axis (often 5-axis) CNC milling machines. Requires careful fixture design due to the complex, often organic shapes produced by topology optimization. CNC machining 3D printed parts requires expertise in handling potentially complex geometries and ensuring proper workholding.
6. Surface Finishing:
Depending on requirements, further surface treatments may be applied:
- Zpevňování povrchu: Bombarding the surface with small spherical media (shot) introduces compressive residual stresses into the surface layer. This is highly beneficial for improving fatigue life, particularly important for cyclically loaded components like steering knuckles.
- Tumbling/Vibratory Finishing: Using abrasive media in a rotating or vibrating bowl to deburr edges, smooth surfaces, and provide a uniform matte finish. Effective for removing minor support nubs and improving overall surface aesthetics.
- Leštění: Can achieve very smooth, mirror-like finishes if required for specific applications (less common for knuckles unless for show vehicles).
- Anodizing/Coating: Applying protective or cosmetic surface layers. Anodizing improves corrosion and wear resistance for aluminum. Specific paints or coatings might be applied for environmental protection or branding.
7. Inspection and Quality Control:
Throughout the post-processing chain, inspection is critical.
- Rozměrová kontrola: CMM or 3D scanning after printing, after heat treatment (to check distortion), and after final machining.
- Nedestruktivní zkoušení (NDT):
- Visual Inspection: Basic check for obvious defects.
- Dye Penetrant Inspection (DPI): Detekuje trhliny porušující povrch.
- X-ray Computed Tomography (CT Scanning): Essential for detecting internal defects like porosity or lack-of-fusion, particularly important for validating the integrity of safety-critical parts. Provides a full 3D view of the internal structure.
- Material Property Verification: Hardness testing, or potentially tensile testing of representative samples printed alongside the main part, to confirm successful heat treatment and material properties.
Post-Processing Workflow Summary:
Build Plate -> Stress Relief -> Part Removal -> Support Removal -> Heat Treatment (T6) -> CNC Machining -> Surface Finishing (e.g., Shot Peening) -> Final Inspection (CMM, NDT) -> Ready for Assembly
Each step adds time and cost to the overall production process. Efficiently integrating these steps requires careful planning and expertise from the AM service provider. Companies like Met3dp, offering end-to-end solutions, understand this integrated workflow, from powder production to finished part, ensuring quality at each stage.
Navigating Challenges: Common Issues in 3D Printing Steering Knuckles and Mitigation Strategies
While metal additive manufacturing, particularly LPBF, offers significant advantages for producing lightweight aluminum steering knuckles, the process is not without its challenges. Achieving consistent, high-quality results, especially with complex geometries and demanding materials like A7075, requires a deep understanding of potential issues and robust mitigation strategies. Awareness of these challenges is crucial for engineers specifying parts and for procurement managers selecting capable suppliers who possess the necessary process control and expertise. Many of these challenges are actively addressed by advancements in machine technology, process monitoring, and materials science, often pioneered by companies focused on industrial AM solutions like Met3dp.
1. Porosity:
Porosity refers to small voids or pores within the printed material, which can significantly degrade mechanical properties, particularly fatigue strength.
- Typy:
- Gas Porosity: Caused by gas (often hydrogen in aluminum alloys) trapped within the melt pool during solidification. Can arise from contaminated powder (moisture) or insufficient shielding gas.
- Lack-of-Fusion (LoF) Porosity: Irregularly shaped voids caused by incomplete melting and fusion between adjacent scan tracks or subsequent layers. Often results from insufficient energy input (laser power too low, scan speed too high) or poor powder layer recoating.
- Detection: Difficult to detect visually on the surface. Best detected internally using X-ray CT scanning.
- Mitigation:
- Vysoce kvalitní prášek: Using clean, dry, spherical powder with controlled PSD, like those produced using Met3dp’s advanced atomization processes, minimizes gas sources. Proper powder handling and storage are essential.
- Optimalizované parametry: Developing and validating robust printing parameters (laser power, speed, hatch spacing, layer thickness) specific to the alloy and machine to ensure complete melting and fusion. Extensive process development is key.
- Effective Shielding Gas: Ensuring high-purity inert gas (Argon) flow to protect the melt pool from atmospheric contamination.
- Monitorování procesů: Advanced systems can monitor melt pool emissions or temperature in real-time to detect anomalies indicative of potential pore formation.
- Izostatické lisování za tepla (HIP): A post-processing step involving high temperature and pressure, which can close internal porosity. However, it adds significant cost and time and is typically reserved for the most critical applications (e.g., aerospace).
2. Cracking:
Cracks can form during or after the printing process, representing critical defects that compromise structural integrity.
- Typy:
- Solidification Cracking (Hot Tearing): Occurs during solidification in alloys with a wide freezing range (like A7075) due to thermal stresses pulling apart the semi-solid structure. Often occurs between grain boundaries.
- Residual Stress Cracking (Cold Cracking): Can occur after cooling due to high internal stresses exceeding the material’s strength or ductility.
- Detection: Can sometimes be visible on the surface but may also be internal (detectable by CT or potentially dye penetrant if surface-breaking).
- Mitigation:
- Alloy Selection/Modification: AlSi10Mg is significantly less prone to solidification cracking than A7075. For high-strength needs, using AM-specific 7xxx series variants with modified compositions or grain refiners can help.
- Parameter Optimization: Tuning laser parameters (e.g., pre-heating scans, specific energy density) can influence the thermal gradients and microstructure to reduce cracking susceptibility. Research into optimized A7075 printing challenges is ongoing.
- Scan Strategy: Using specific scan patterns (e.g., island scanning, chessboard patterns) can help distribute thermal stress more evenly.
- Úleva od stresu: Prompt and appropriate stress relief heat treatment is crucial to reduce residual stresses that could lead to cold cracking.
- Support Strategy: Proper support design helps manage thermal stresses during the build.
3. Warping and Distortion:
Uneven shrinkage and the buildup of residual stresses can cause the part to warp or distort during printing or after removal from the build plate.
- Causes: Primarily driven by thermal gradients between the hot melt pool and cooler surrounding material/substrate. Large, flat sections or abrupt changes in thickness are particularly prone.
- Detection: Visual inspection, CMM, or 3D scanning.
- Mitigation:
- Build Plate Heating: Pre-heating the build plate reduces the thermal gradient between the first layers and the substrate.
- Robustní podpůrné struktury: Securely anchoring the part to the build plate, especially large or overhanging sections, helps resist warping forces.
- Optimalizovaná orientace: Orienting the part to minimize large flat surfaces parallel to the build plate or long unsupported overhangs.
- Scan Strategy: As with cracking, specific scan strategies can help manage stress buildup.
- Prompt Stress Relief: Performing stress relief heat treatment before removing the part from the build plate (if possible) or immediately after helps stabilize the component.
- Design Modification (DfAM): Adding ribs or changing geometry slightly to increase stiffness against warping forces.
4. Support Removal Difficulties and Surface Quality:
While necessary, support structures present challenges in removal and impact surface quality.
- Výzvy: Accessing internal supports, breaking delicate features during removal, time/labor cost, leaving witness marks/scars on the part surface.
- Mitigation:
- DfAM: Designing for self-support, optimizing orientation, using easily removable support types (e.g., tree supports, conical supports), placing supports on non-critical surfaces.
- Optimized Support Parameters: Fine-tuning the connection points between the support and the part for easier detachment.
- Vhodné techniky odstraňování: Using the right tools and methods (manual, machining, EDM) for the specific support type and location.
- Post-Removal Finishing: Planning for subsequent machining or surface finishing (tumbling, shot peening) to remove witness marks on critical areas.
5. Powder Management and Recycling:
Handling fine metal powders requires care, and reusing unsintered powder is important for cost-effectiveness but needs control.
- Výzvy:
- Bezpečnost: Metal powders (especially aluminum) can be flammable or pose respiratory hazards if not handled correctly (requires proper PPE, grounding, inert atmosphere handling).
- Degradace prášku: Repeated exposure to heat in the build chamber or handling can cause powder oxidation or changes in PSD, affecting processability and part quality in subsequent builds.
- Cross-Contamination: Ensuring powder types are not mixed.
- Mitigation:
- Proper Handling Procedures: Using closed-loop powder handling systems, inert gas environments, appropriate PPE.
- Powder Quality Monitoring: Regularly testing recycled powder (e.g., chemistry analysis, PSD, flowability) to ensure it still meets specifications.
- Controlled Recycling Strategy: Blending recycled powder with virgin powder in controlled ratios, establishing limits on the number of reuse cycles.
- Supplier Quality: Starting with high-quality, consistent powder from suppliers like Met3dp minimizes initial variability. Their expertise in powder production ensures a reliable starting material. Understanding Met3dp’s tiskových metod and how they relate to powder interaction is beneficial.
Navigating these challenges requires a combination of advanced machine technology, optimized and validated process parameters, high-quality materials, rigorous process control, and skilled operators and engineers. Partnering with an AM service provider or technology supplier like Met3dp, who invests in research, process development, and quality systems, significantly increases the probability of successfully overcoming these hurdles and producing reliable, high-performance 3D printed aluminum steering knuckles.
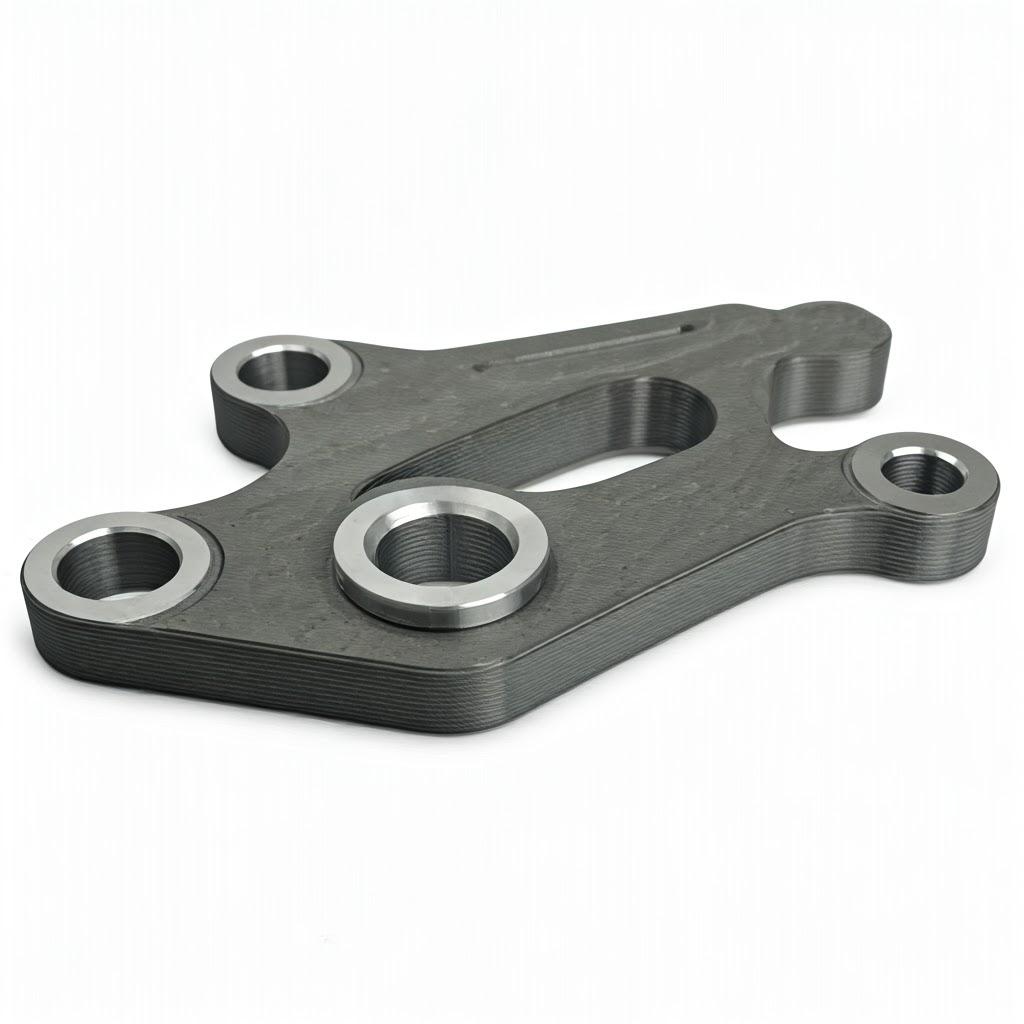
Supplier Selection: Choosing the Right Metal 3D Printing Partner for Automotive Components
Transitioning to additive manufacturing for a critical component like a steering knuckle is a significant step. The success of this transition hinges not only on the design and material but profoundly on the capabilities and reliability of your chosen poskytovatel služeb 3D tisku kovů. Selecting the right partner is paramount, especially within the demanding automotive sector where quality, repeatability, and traceability are non-negotiable. For procurement managers and engineering teams vetting potential suppliers, a thorough evaluation process based on key criteria is essential. Not all AM providers are created equal, and partnering with one that possesses deep expertise in automotive applications, aluminum alloys, and robust quality systems is critical for mitigating risks and achieving desired outcomes.
Here are key factors to consider when evaluating and selecting an automotive additive manufacturing supplier:
1. Quality Management Systems and Certifications:
- ISO 9001: This is the foundational requirement, demonstrating a commitment to documented processes and continual improvement in quality management.
- IATF 16949: While full IATF certification might still be emerging among AM-focused providers, demonstrating adherence to its principles or having experience supplying into IATF-compliant supply chains is a significant advantage. It shows an understanding of automotive requirements regarding process control, risk management (FMEA), traceability, and documentation (PPAP – Production Part Approval Process elements).
- Aerospace Certifications (AS9100): If a provider holds AS9100, it often indicates highly mature quality systems and process controls, which can be beneficial even for automotive applications.
2. Technical Expertise and Engineering Support:
- Materials Science: Deep knowledge of the specific aluminum alloys (AlSi10Mg, A7075) is crucial, including their behavior during LPBF, required heat treatments, and achievable mechanical properties. Ask about their experience with these specific materials.
- DfAM Capability: The supplier should not just be a “print shop” but a true partner who understands and can actively contribute to Design for Additive Manufacturing (DfAM). They should be able to provide feedback on design printability, suggest optimizations for lightweighting or support reduction, and collaborate on developing the optimal geometry.
- Process Physics Understanding: A thorough grasp of the LPBF process, including thermal management, laser-material interaction, parameter development, and defect mitigation strategies.
- Post-Processing Knowledge: Expertise in the required sequence of post-processing steps (stress relief, heat treatment, machining strategies for AM parts, finishing) and their impact on the final component.
3. Machine Technology and Capabilities:
- Industrial-Grade Equipment: Ensure the provider uses modern, well-maintained industrial LPBF machines specifically suited for processing aluminum alloys reliably. Key features include adequate build volume, inert atmosphere control, and potentially in-situ process monitoring capabilities.
- Accuracy and Reliability: Look for evidence of machine calibration procedures and data supporting claims of accuracy and repeatability. Providers like Met3dp emphasize their printers’ industry-leading print volume, accuracy and reliability, which are critical metrics for components requiring precision.
- Capacity: Assess their machine capacity and utilization to ensure they can meet your required lead times for prototypes and potential small series production runs.
4. Material Sourcing and Control:
- Kvalita prášku: How does the supplier source and qualify their aluminum powder? Do they have stringent incoming inspection processes? What are their powder handling and recycling protocols to prevent contamination and degradation?
- Material Traceability: Can they provide full traceability for the powder batch used for your specific parts, linking back to the original powder manufacturer’s certificate of conformity?
- In-House Powder Production: Companies like Met3dp, which manufacture both the printers and the high-quality spherical metal powders using advanced methods like gas atomization and PREP, offer a distinct advantage. This vertical integration provides greater control over material quality and consistency, directly impacting the final part’s integrity. Their O nás page details their background and commitment to comprehensive AM solutions.
5. Comprehensive Post-Processing Capabilities:
- Integrated Workflow: Ideally, the provider should offer a seamless workflow that includes necessary post-processing steps either in-house or through tightly controlled, qualified partners. This includes:
- Controlled atmosphere furnaces for stress relief and heat treatment (with calibration records).
- Multi-axis CNC machining capabilities suitable for complex AM part geometries.
- Support removal expertise.
- Surface finishing options (e.g., shot peening, tumbling).
- Comprehensive inspection and NDT capabilities.
- Supply Chain Management: If outsourcing post-processing, evaluate their supplier management and quality control processes for these external steps.
6. Robust Quality Control and Inspection:
- Monitorování procesů: Do they utilize any in-situ monitoring during the build? What are their standard procedures for build log review and parameter verification?
- Rozměrová kontrola: Availability and calibration records for CMMs and/or 3D scanners. Standard inspection reporting formats.
- Nedestruktivní zkoušení (NDT): Crucial for safety-critical parts like steering knuckles. Assess their capabilities and experience with:
- CT Scanning: Highly recommended for internal defect detection (porosity, cracks).
- Dye Penetrant Inspection (DPI): For surface crack detection.
- Other methods as required (e.g., ultrasonic testing, radiographic testing).
- Material Testing: Capability to perform hardness testing, tensile testing (on representative samples), or metallographic analysis to verify material properties and heat treatment success.
7. Track Record, Case Studies, and References:
- Relevant Experience: Have they successfully produced similar parts in terms of complexity, material, and criticality? Specific experience with automotive components is highly desirable.
- Případové studie: Request examples or case studies demonstrating their capabilities and problem-solving skills.
- References: Ask for customer references, particularly from the automotive or similarly demanding industries.
8. Cost, Lead Time, and Communication:
- Transparent Quoting: Provides detailed quotes outlining all cost components (material, build time, labor, post-processing, NDT).
- Realistic Lead Times: Communicates clear and achievable lead times for different stages (prototyping, production).
- Communication & Project Management: Clear points of contact, regular updates, and responsiveness to inquiries.
Evaluation Checklist Summary:
Kritéria | Klíčové otázky | Importance (Steering Knuckle) |
---|---|---|
Quality Certs (ISO/IATF) | What certifications do you hold? How do you align with automotive standards? | Velmi vysoká |
Technické znalosti | What is your experience with AlSi10Mg/A7075? Can you support DfAM? How do you optimize print parameters? | Velmi vysoká |
Machine Capability | What machines do you use? What is their build volume/accuracy? How are they maintained/calibrated? | Vysoký |
Material Control | Where do you source powder? How do you qualify/handle/recycle it? Can you ensure traceability? | Velmi vysoká |
Následné zpracování | What steps are in-house vs. outsourced? How do you control quality for heat treat/machining/NDT? | Velmi vysoká |
Quality Control/NDT | What are your standard inspection procedures? Do you offer CT scanning? How do you ensure part integrity? | Velmi vysoká |
Track Record | Can you share case studies or references for similar automotive/critical parts? | Vysoký |
Náklady a dodací lhůta | Can you provide detailed quotes? What are your typical lead times? How is project communication managed? | Vysoký |
Export do archů
Výběr správného partner pro kovový 3D tisk is an investment in quality and reliability. Conducting due diligence using these criteria will help ensure you select a supplier, like Met3dp, capable of delivering high-performance, lightweight aluminum steering knuckles that meet the rigorous demands of the automotive industry.
Cost Analysis and Lead Times: Understanding the Economics of 3D Printed Steering Knuckles
While the technical advantages of 3D printed aluminum steering knuckles are compelling, automotive procurement managers and engineers must also carefully consider the economic viability and production timelines. The cost structure and lead times for additive manufacturing differ significantly from traditional casting or forging. Understanding these differences is key to making informed decisions about when and where to implement AM for components like steering knuckles.
Key Cost Drivers for 3D Printed Steering Knuckles (LPBF):
Unlike traditional methods dominated by tooling costs at low volumes, AM costs are more directly tied to the part itself and the time it takes to produce it.
- Náklady na materiál:
- Typ prášku: The raw material cost varies significantly between alloys. High-strength alloys like A7075 are generally more expensive than standard AM alloys like AlSi10Mg.
- Část Objem: The sheer volume of material in the final part, including any necessary support structures, directly impacts cost. This highlights the economic benefit of topology optimization and DfAM – reducing material usage saves direct costs.
- Powder Utilization/Recycling: Efficient powder handling and recycling strategies employed by the service provider can influence the effective material cost passed on to the customer. Inefficient use or excessive waste increases costs.
- Machine Time (Build Time):
- Primary Cost Driver: This is often the largest single cost component. It’s determined by how long the LPBF machine is occupied printing the part(s).
- Influencing Factors:
- Part Volume & Height: Larger and taller parts take longer to print layer by layer.
- Část Složitost: More complex geometries might require slower scan speeds or more intricate laser scanning paths.
- Number of Parts per Build (Nesting): Printing multiple parts simultaneously in one build job optimizes machine utilization and can reduce the per-part machine time cost. Efficient nesting is key for small series production.
- Tloušťka vrstvy: Thinner layers improve surface finish but significantly increase the number of layers and thus the build time.
- Hodinová sazba stroje: This rate encompasses machine depreciation, energy consumption, inert gas usage, maintenance, and facility overheads. Rates vary depending on the machine’s sophistication and the provider’s cost structure.
- Náklady na pracovní sílu:
- Pre-Processing: Engineering time for DfAM consultation, build preparation (orientation, support generation), slicing, and machine setup.
- Následné zpracování: This can be a very significant labor component, including:
- Part removal from build plate.
- Manual or semi-automated support removal (can be time-consuming for complex parts).
- CNC machine setup and operation.
- Manual finishing, deburring, or surface treatment.
- Inspection and quality control procedures.
- Post-Processing Costs (Beyond Labor):
- Tepelné zpracování: Furnace time, energy consumption, cost of controlled atmospheres.
- CNC obrábění: Machine time, tooling wear, programming effort. Machining complex, topology-optimized shapes can be more challenging than machining simple blocks.
- Povrchové úpravy: Costs associated with shot peening, tumbling, anodizing, or coating.
- NDT: Costs for specialized inspection like CT scanning, including equipment time and analysis labor.
- Quality Assurance & Documentation:
- The level of inspection, testing, and documentation required (e.g., detailed inspection reports, material certifications, traceability records) adds to the overall cost. Requirements for automotive production (even small series) are typically higher than for general industrial prototypes.
Cost Comparison: AM vs. Traditional Methods (Casting/Forging)
- Low Volumes (Prototypes, Custom Parts, < ~100-500 units): AM is often nákladově efektivnější. The elimination of high upfront tooling costs (tens to hundreds of thousands of dollars for molds/dies) makes AM highly competitive. Design changes are also cheap in AM, whereas tooling modifications are expensive.
- Medium Volumes (~500 – ~5,000 units): This is often a grey area. The per-part cost of AM remains relatively stable, while the amortized tooling cost for casting/forging decreases significantly. The crossover point depends heavily on part complexity, material, and the specific costs of tooling vs. AM production. AM might still be viable if its unique benefits (lightweighting, consolidation) provide significant downstream value.
- High Volumes (> ~5,000 – 10,000+ units): Traditional casting or forging typically becomes significantly cheaper per part, as the high tooling investment is amortized over many units, and cycle times per part are generally much faster than AM build times.
Cost Factors Summary Table:
Nákladový faktor | Key Influences | Typical Impact on AM Cost | Poznámky |
---|---|---|---|
Materiál | Alloy type (A7075 > AlSi10Mg), Part Volume (Topology Opt. helps) | Vysoký | Powder cost per kg |
Machine Time | Part Height/Volume, Nesting Efficiency, Layer Thickness | Velmi vysoká | Often the largest cost component |
Labor (Pre/Post) | DfAM Effort, Build Setup, Support Removal, Machining Setup, Inspection | Vysoký | Support removal & machining can be very labor-intensive |
Následné zpracování | Heat Treatment, CNC Time, Surface Treatment Choice, NDT Requirements | Mírná až vysoká | NDT (esp. CT) can add significant cost |
Zajištění kvality | Level of documentation, testing, certification needs | Nízká až střední | Higher for automotive production |
VS. Traditional | No Tooling Cost | Major Advantage (Low Vol) | Casting/Forging Tooling = High Upfront Cost |
VS. Traditional | Slower Cycle Time | Disadvantage (High Vol) | Casting/Forging = Fast cycle times once tooling is ready |
Export do archů
Lead Times for 3D Printed Steering Knuckles:
Lead times in AM are also structured differently compared to traditional methods.
- Vytváření prototypů:
- AM: Significantly faster. Once the design is finalized, printing can often start within days. Including printing time (which could be 1-3 days depending on size/complexity) and essential post-processing (stress relief, basic support removal, maybe quick machining of one feature), functional prototypes can often be delivered in 1 to 3 weeks.
- Traditional: Requires tooling design and fabrication first, which can take 6-12 weeks or more. The total time for the first cast/forged prototypes is often measured in months.
- Small Series Production (e.g., 10-100 units):
- AM: Lead times depend heavily on machine availability, total build time (can multiple builds be nested?), and the full post-processing workflow. Typical lead times might range from 4 to 8 weeks, potentially longer if extensive machining or complex NDT is required. Production can often start relatively quickly after order confirmation.
- Traditional: Once tooling is approved (after the lengthy prototype phase), production of parts can be fast (e.g., hundreds per week). However, the initial tooling lead time dominates the overall project timeline.
- Factors Influencing AM Lead Times:
- Part Complexity & Size: Directly impacts print time.
- Požadavky na následné zpracování: Extensive machining or specialized treatments add significant time. Heat treatment cycles alone take hours/days.
- Supplier Capacity: Machine availability and backlog at the chosen service provider.
- Quantity Ordered: Larger batches may require multiple print runs.
- Quality Requirements: Stringent inspection and documentation add time.
In summary, the metal 3D printing cost analysis reveals AM’s strength in eliminating tooling costs, making it ideal for prototypes and low-volume production where flexibility and speed are paramount. While per-part costs are higher than mass-produced traditional components, the value derived from lightweighting, performance gains, and accelerated development often justifies the investment. Understanding the doba realizace aditivní výroby advantages for prototyping and initial production runs is crucial for project planning in the fast-moving automotive industry.
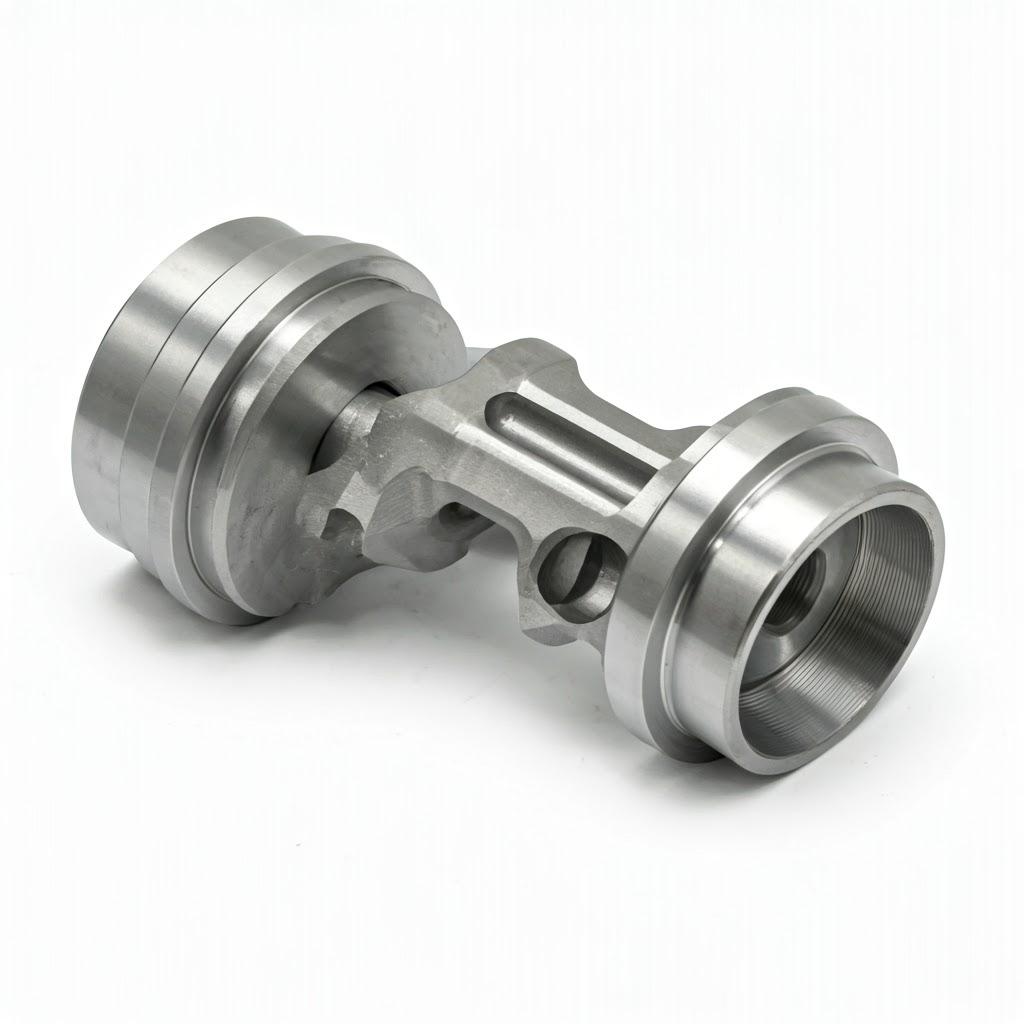
Conclusion: The Future is Lightweight – Embracing AM for Advanced Steering Knuckle Design
The journey through the intricacies of 3D printing aluminum steering knuckles reveals a technology poised to reshape aspects of automotive component design and manufacturing. By leveraging the power of Laser Powder Bed Fusion (LPBF) with advanced aluminum alloys like AlSi10Mg and high-strength A7075, engineers can break free from the constraints of traditional casting and forging. The benefits of lightweight steering knuckles realized through additive manufacturing are undeniable: significant reductions in unsprung mass leading to enhanced vehicle dynamics, improved fuel/energy efficiency critical for EVs, and unparalleled design freedom enabling topology optimization and part consolidation.
We’ve explored the critical role of Design for Additive Manufacturing (DfAM), the achievable levels of precision and the essential nature of post-processing steps like heat treatment and CNC machining. We’ve also navigated the common challenges, emphasizing that with expert knowledge, process control, and high-quality materials, these hurdles are surmountable. The economic analysis highlights AM’s clear advantages in rapid prototyping and low-volume production, accelerating development cycles and enabling customized solutions previously out of reach.
The future of automotive components, particularly in performance segments, electric vehicles, and niche applications, will increasingly involve additive manufacturing. Steering knuckles serve as a prime example of how AM can deliver tangible performance benefits by producing complex, highly optimized, lightweight structures that were simply not feasible before.
However, realizing this potential requires collaboration and expertise. Choosing the right AM partner – one with proven capabilities in materials, process control, quality assurance, and a deep understanding of automotive requirements – is crucial for success.
Met3dp stands ready to be that partner. With our integrated capabilities spanning advanced metal powder production using industry-leading atomization techniques and the manufacture of high-performance SEBM and LPBF printers known for their accuracy and reliability, we offer comprehensive solutions. Our team possesses decades of collective expertise in metal additive manufacturing, ready to assist you in exploring the possibilities of AM for your automotive components.
Are you ready to explore how 3D printed aluminum components can revolutionize your next automotive project? Whether you are an engineer seeking to push performance boundaries or a procurement manager looking for innovative and agile manufacturing solutions, we invite you to connect with us.
Visit our website at https://met3dp.com/ to learn more about our technologies and services, or contact us directly to discuss your specific application needs. Let Met3dp help you unlock the future of lightweight, high-performance automotive manufacturing.
Často kladené otázky (FAQ)
Q1: Are 3D printed aluminum steering knuckles strong and safe enough for automotive use?
A: Yes, absolutely, provided they are designed, manufactured, and validated correctly. Safety and strength depend on several factors:
- Proper Design (DfAM): Utilizing topology optimization and FEA to ensure the design can withstand all operational loads with appropriate safety factors.
- Výběr materiálu: Choosing the right alloy (e.g., AlSi10Mg or A7075) for the required strength and fatigue properties.
- Quality Printing Process: Using industrial-grade LPBF machines with validated parameters to produce fully dense parts (>99.5%) with minimal defects (porosity, cracks). This requires high-quality powder and stringent process control.
- Correct Post-Processing: Performing necessary stress relief and heat treatments (like T6 temper) to achieve optimal mechanical properties.
- Rigorous Testing & Validation: Conducting thorough mechanical testing (tensile, fatigue) and NDT (especially CT scanning) to verify structural integrity and ensure the part meets or exceeds OEM or regulatory performance standards. When these steps are followed meticulously by experienced providers, 3D printed knuckles can be as safe and strong, or even stronger in terms of specific strength (strength-to-weight), as traditionally manufactured counterparts.
Q2: Is 3D printing cost-effective for steering knuckles compared to casting or forging?
A: The cost-effectiveness is highly dependent on the production volume:
- Prototypes & Very Low Volume (< 10-50 units): AM is generally highly cost-effective because it completely avoids the massive upfront investment in casting molds or forging dies.
- Low-to-Medium Volume (~50 – ~1000 units): AM remains competitive and is often the most economical choice, especially when considering the speed and design flexibility benefits. The crossover point varies based on complexity.
- High Volume (>> 1000 units): Traditional casting or forging typically becomes significantly cheaper per part due to economies of scale and faster cycle times, assuming a stable design where tooling costs can be amortized over many units. However, even at higher volumes, AM might be justified if it enables a design that provides substantial lightweighting or performance benefits unachievable through traditional means, leading to greater overall system value (e.g., significantly extended EV range).
Q3: Can you achieve the same fatigue life with a 3D printed aluminum knuckle compared to a forged one?
A: Achieving comparable or potentially even superior fatigue life is feasible with 3D printed aluminum knuckles, but it requires careful attention to detail throughout the process:
- Optimalizace designu: Topology optimization can create designs with smoother stress flow, potentially reducing stress concentrations compared to some traditional designs.
- Material Integrity: Minimizing internal defects like porosity through optimized printing is critical, as pores act as fatigue initiation sites. High-quality powder and process control are key.
- Povrchová úprava: As-printed surfaces can be rough, potentially impacting fatigue. Post-processing steps like shot peening are highly effective in introducing beneficial compressive stresses on the surface, significantly enhancing fatigue resistance, often beyond that of non-peened forged parts. Machining critical areas also removes potentially rough as-printed surfaces.
- Material Choice & Heat Treatment: Using high-strength alloys like A7075 (processed correctly) and ensuring proper heat treatment contributes to better fatigue performance. While forged parts benefit from a wrought microstructure, a well-designed, defect-minimized, and appropriately post-processed AM part can meet or exceed the fatigue requirements for demanding applications. Validation through rigorous fatigue testing is essential.
Sdílet na
Facebook
Cvrlikání
LinkedIn
WhatsApp
E-mailem
MET3DP Technology Co., LTD je předním poskytovatelem řešení aditivní výroby se sídlem v Qingdao v Číně. Naše společnost se specializuje na zařízení pro 3D tisk a vysoce výkonné kovové prášky pro průmyslové aplikace.
Dotaz k získání nejlepší ceny a přizpůsobeného řešení pro vaše podnikání!
Související články
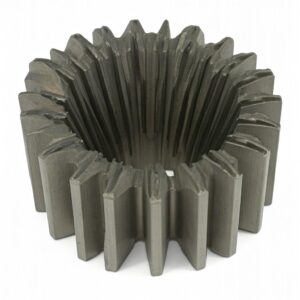
Vysoce výkonné segmenty lopatek trysek: Revoluce v účinnosti turbín díky 3D tisku z kovu
Přečtěte si více "
13. května 2025
Žádné komentáře
O Met3DP
Nedávná aktualizace
Náš produkt
KONTAKTUJTE NÁS
Nějaké otázky? Pošlete nám zprávu hned teď! Po obdržení vaší zprávy obsloužíme vaši žádost s celým týmem.
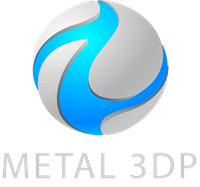
Kovové prášky pro 3D tisk a aditivní výrobu
SPOLEČNOST
PRODUKT
kontaktní informace
- Město Qingdao, Shandong, Čína
- [email protected]
- [email protected]
- +86 19116340731