Custom 3D Printed Heat Shields for Vehicles: Advanced Thermal Management with Metal AM
Obsah
Introduction: Revolutionizing Automotive Thermal Management with Metal 3D Printed Heat Shields
The relentless pursuit of performance, efficiency, and safety in the automotive industry constantly pushes the boundaries of engineering and manufacturing. One critical, yet often overlooked, aspect of vehicle design is thermal management. Effectively controlling heat generated by engines, exhaust systems, turbochargers, batteries, and other components is paramount not only for protecting sensitive parts and ensuring passenger comfort but also for optimizing vehicle performance and longevity. Traditionally, automotive heat shields – the primary components tasked with this thermal control – have been manufactured using conventional methods like stamping and forming sheet metal. While effective for mass production of simple geometries, these techniques often impose limitations on design complexity, weight optimization, and the speed of development, particularly for niche applications, performance vehicles, or the rapidly evolving electric vehicle (EV) sector.
Vstupte kov 3D tisk, also known as additive manufacturing (AM). This transformative technology is rapidly reshaping how complex components are designed and produced across various industries, and the automotive sector is no exception. Specifically for automotive heat shields, metal AM offers a paradigm shift, enabling the creation of highly customized, complex, and lightweight solutions that were previously unfeasible or prohibitively expensive. By building parts layer by layer directly from digital designs using high-performance metal powders, engineers can now design heat shields optimized precisely for their specific thermal environment, integrating intricate features, conformal shapes, and optimized airflow channels. This capability is crucial for managing heat in tightly packaged engine bays, protecting advanced battery systems in EVs, and boosting the performance of turbocharged engines.
The significance of this technological advancement cannot be overstated. As vehicles become more sophisticated, incorporating denser electronics, powerful hybrid systems, and high-performance powertrains, the demands on thermal management systems intensify. Traditional heat shields, often designed as compromises between cost, manufacturability, and performance, struggle to meet these escalating requirements. Metal additive manufacturing provides the design freedom necessary to create bespoke řešení tepelného managementu tailored to the unique challenges of modern vehicle architectures. From rapid prototyping during development cycles to producing small-to-medium batches of specialized custom vehicle components, metal AM offers unprecedented agility and performance potential.
This blog post delves into the world of custom 3D printed heat shields for vehicles. We will explore their applications, the compelling advantages of using 3D tisk z kovu over traditional methods, recommended materials like Aluminum alloy AlSi10Mg and Nickel superalloy IN625, crucial design considerations, achievable quality metrics, post-processing needs, potential challenges, and factors influencing cost and lead time. Furthermore, we will guide engineers and procurement managers in selecting the right additive manufacturing automotive partner.
Leading this charge into advanced manufacturing solutions is Met3dp. Headquartered in Qingdao, China, Met3dp Technology Co., LTD specializes in state-of-the-art 3D tisk z kovu equipment and the production of high-performance metal powders crucial for industrial applications. Our expertise in powder metallurgy, leveraging advanced gas atomization and Plasma Rotating Electrode Process (PREP) technologies, ensures the highest quality spherical metal powders – the foundation for reliable and high-performance 3D printed components. With a portfolio including innovative alloys and decades of collective expertise, Met3dp provides comprehensive solutions, empowering industries like aerospace, medical, and particularly automotive, to harness the full potential of additive manufacturing for demanding applications like custom heat shields. We partner with organizations globally to accelerate their digital manufacturing transformations, offering industry-leading print volume, accuracy, and reliability.
Join us as we explore how metal AM is not just improving automotive heat shields but revolutionizing the approach to thermal management in vehicles, paving the way for lighter, more efficient, and higher-performing automobiles. Whether you are an automotive engineer seeking innovative solutions, a Tier 1 supplier looking for advanced manufacturing capabilities, or a procurement manager sourcing custom automotive components, understanding the potential of 3D printed heat shields is essential in today’s competitive landscape.
What are Automotive Heat Shields Used For? Key Applications and Industries
Automotive heat shields are essential passive thermal insulation components designed to protect vehicle parts, occupants, and the surrounding environment from excessive heat generated primarily by the powertrain and exhaust system. Their fundamental heat shield function is to act as a barrier, reflecting radiant heat away from sensitive areas and reducing convective heat transfer. Without effective heat shields, numerous problems can arise, including component degradation, reduced performance, increased emissions, potential fire hazards, and compromised passenger comfort due to heat radiating into the cabin. The strategic placement and design of these shields are critical for the overall reliability and safety of any vehicle.
The applications of heat shields within a vehicle are diverse, addressing specific thermal challenges in various locations:
- Exhaust System Heat Shields: This is perhaps the most common application. Exhaust manifolds, catalytic converters, mufflers, and exhaust pipes can reach extremely high temperatures (often exceeding 600°C or 1100°F, especially near the engine or catalytic converter). Heat shields are crucial here to:
- Protect underbody components (fuel lines, brake lines, wiring harnesses, fuel tank).
- Prevent heat from damaging chassis coatings or structural elements.
- Reduce heat radiated towards the passenger cabin floor.
- Protect surrounding components during stationary periods (e.g., preventing damage to asphalt or drying vegetation).
- Keywords: Exhaust heat shield, catalytic converter shield, muffler heat shield, underbody heat shield.
- Engine Compartment Shielding: The engine bay is a densely packed environment with numerous heat sources (engine block, turbocharger, exhaust manifold) and heat-sensitive components (electronics, sensors, hoses, plastic parts). Heat shields are used to:
- Protect specific components like the starter motor, alternator, ECU (Engine Control Unit), and various sensors from direct heat radiation.
- Shield plastic components (e.g., engine covers, intake manifolds) from warping or melting.
- Manage under-hood temperatures to ensure optimal engine performance and prevent vapor lock in fuel lines.
- Protect the hood paint from excessive heat.
- Keywords: Engine compartment shielding, turbocharger heat shield, manifold heat shield, firewall insulation.
- Turbocharger Heat Shields: Turbochargers operate at very high temperatures due to compressing intake air and being driven by hot exhaust gases. Specific turbocharger heat shields (often called “turbo blankets” when flexible, but rigid shields are also common) are essential to:
- Protect adjacent components (wiring, hoses, actuators) from extreme radiant heat.
- Keep heat within the turbine housing, improving turbo efficiency and response (reducing spool time).
- Lower overall under-hood temperatures.
- Keywords: Turbo heat shield, performance turbo parts, automotive thermal insulation.
- Electric Vehicle (EV) and Hybrid Battery Pack Shielding: While EVs don’t have hot exhaust systems, their high-voltage battery packs are sensitive to temperature extremes, both hot and cold. Thermal management is critical for battery performance, longevity, and safety. Heat shields can play a role in:
- Protecting the battery pack from external heat sources (e.g., ambient heat, heat from nearby motors or power electronics).
- Acting as part of a larger thermal management system to maintain optimal battery operating temperatures.
- Providing thermal runaway protection by acting as a barrier in case of cell failure.
- Keywords: EV battery thermal management, battery pack heat shield, electric vehicle components.
- Fuel Tank Protection: Heat shields are often placed between the exhaust system and the fuel tank to prevent fuel overheating, which could lead to excessive evaporation, vapor pressure issues, or, in extreme cases, safety hazards.
- Keywords: Fuel tank heat shield, automotive safety components.
- Další aplikace: Depending on the vehicle design, heat shields might also be used near brakes, transmissions, differentials, or specific electronic modules requiring thermal protection.
Industries Benefiting:
The need for effective heat shielding spans across the entire automotive ecosystem:
- Automotive OEMs (Original Equipment Manufacturers): Car manufacturers are the primary consumers, integrating heat shields into every vehicle they produce, from economy cars to luxury sedans and heavy-duty trucks. They require reliable, cost-effective solutions that meet stringent performance and durability standards. Sourcing OEM automotive parts like heat shields often involves partnering with specialized Tier 1 supplier solutions.
- Performance Aftermarket: Companies providing upgrades for performance vehicles often develop custom heat shields designed for higher heat loads generated by modified engines, larger turbos, or high-flow exhaust systems. Here, performance and weight savings are often prioritized, making advanced materials and manufacturing methods like 3D printing attractive.
- Motorsports: Racing applications push thermal management to the extreme. Teams require highly optimized, lightweight, and extremely durable heat shields capable of withstanding intense heat cycles and vibrations. Customization and rapid iteration are key, making metal AM an ideal fit.
- Electric Vehicle Manufacturers: As mentioned, EVs have unique thermal management needs, particularly around battery packs and power electronics. Custom-designed heat shields are becoming increasingly important in this rapidly growing sector.
- Specialty Vehicles: Manufacturers of vehicles like RVs, buses, agricultural machinery, and construction equipment also utilize heat shields tailored to their specific operational environments and heat sources.
Understanding these diverse applications highlights the critical role of heat shields and underscores the potential for advanced manufacturing techniques like metal 3D printing to provide superior solutions compared to traditional methods, especially where complexity, customization, or performance optimization are required. The ability to quickly design and produce application-specific shields offers significant advantages across all these automotive segments. Finding a reliable automotive heat shield supplier capable of leveraging these advanced methods is becoming increasingly crucial.
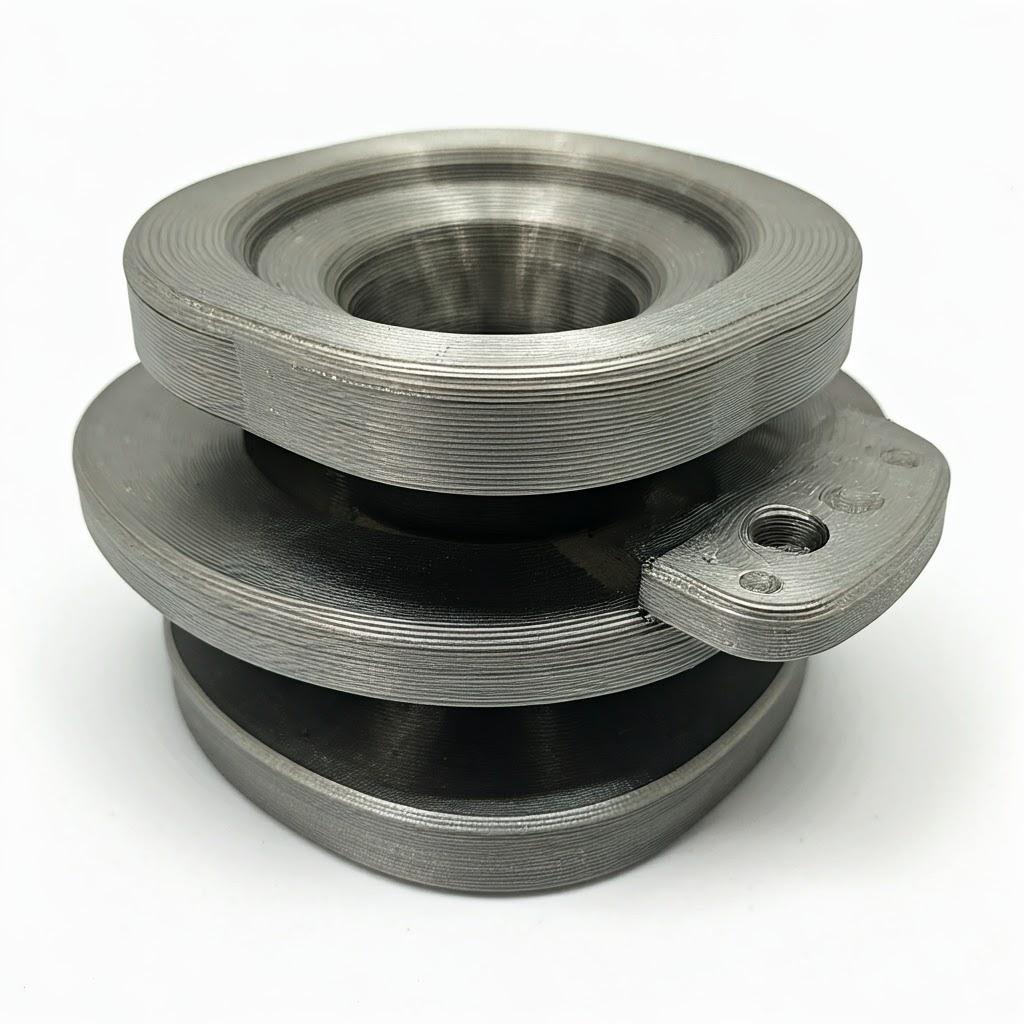
Why Use Metal 3D Printing for Automotive Heat Shields? Unlocking Design Freedom and Performance
While traditional manufacturing methods like stamping and hydroforming have served the automotive industry well for producing high volumes of relatively simple heat shields, they encounter limitations when faced with the increasing complexity, performance demands, and customization requirements of modern vehicles. 3D tisk z kovu, particularly Powder Bed Fusion (PBF) technologies such as Selective Laser Melting (SLM) or Direct Metal Laser Sintering (DMLS), offers a compelling alternative, unlocking significant advantages for heat shield design and production. The benefits of additive manufacturing in this context directly address the shortcomings of conventional processes.
Here’s a detailed comparison and exploration of why metal AM vs stamping or forming is advantageous for heat shields:
- Unparalleled Design Freedom & Complexity:
- Traditional: Stamping is limited by tooling constraints, draft angles, and the formability of sheet metal. Creating complex curves, integrated features, or hollow structures is difficult, costly, or impossible. Assembly of multiple stamped pieces is often required for complex shapes.
- Metal AM: Additive manufacturing builds parts layer-by-layer from a digital file, allowing for virtually unlimited geometric complexity. This enables:
- Complex Geometry Heat Shields: Shields can perfectly conform to surrounding components, maximizing protection in tightly packed spaces.
- Integrated Features: Mounting points, brackets, fasteners, stiffening ribs, and even airflow channels can be designed directly into the heat shield, reducing part count, assembly time, and potential points of failure.
- Internal Structures: Hollow sections or internal lattice/gyroid structures can be incorporated to reduce weight while maintaining stiffness and thermal performance.
- Optimalizace topologie: Software can be used to optimize the shield’s shape, removing material where it’s not needed for structural integrity or thermal performance, leading to significant weight savings.
- Odlehčení:
- Traditional: Sheet metal shields often have uniform thickness, and achieving significant weight reduction requires using thinner gauges (compromising stiffness/durability) or switching to more expensive, harder-to-form materials.
- Metal AM: The ability to create optimized geometries and internal lattice structures allows for drastic weight reduction without sacrificing performance. Using lightweight metal powders like AlSi10Mg further enhances this benefit. Lightweight automotive components contribute directly to improved fuel efficiency, better handling, and increased payload capacity.
- Rapid Prototyping and Development Speed:
- Traditional: Creating stamping dies is time-consuming and expensive. Design iterations require costly tooling modifications or entirely new tools, significantly slowing down the development cycle.
- Metal AM: Prototypes can be printed directly from CAD data in days, sometimes hours, without the need for tooling. This allows engineers to quickly test different designs, validate performance (thermal and structural), and make rapid iterations. This capability for rapid prototyping heat shields drastically accelerates product development and time-to-market.
- Customization and On-Demand Production:
- Traditional: Stamping is geared towards mass production. Producing small batches or custom variations is economically unviable due to high tooling costs.
- Metal AM: Ideal for low-to-mid volume production runs and customization. Each part can be unique without tooling changes. This enables on-demand heat shield production for niche vehicles, aftermarket parts, motorsports applications, or replacement parts for older models where original tooling no longer exists. This flexibility aligns perfectly with the growing trend towards vehicle personalization and specialized models.
- Material Options and Performance:
- Traditional: Limited to sheet metals that are readily formable (various steels, aluminum alloys). Incorporating higher-performance alloys can be challenging and expensive.
- Metal AM: A wider range of high-performance alloys, including nickel superalloys like IN625 (ideal for extreme heat) or specialized aluminum alloys like AlSi10Mg, can be readily processed. These materials offer superior thermal resistance, corrosion resistance, or strength-to-weight ratios compared to conventional options. Met3dp, as a specialized metal powder supplier, provides high-quality AlSi10Mg and IN625 powders optimized for AM processes, ensuring reliable material properties.
- Konsolidace částí:
- Traditional: Complex heat shield assemblies often consist of multiple stamped parts welded or fastened together.
- Metal AM: The design freedom allows multiple components of an assembly to be consolidated into a single, complex 3D printed part. This reduces assembly time, labor costs, inventory management, and potential leak paths or failure points associated with joints.
Summary Table: Metal AM vs. Traditional Manufacturing for Heat Shields
Vlastnosti | Metal 3D Printing (e.g., SLM/DMLS) | Traditional Stamping/Forming | Advantage of Metal AM |
---|---|---|---|
Složitost návrhu | Virtually unlimited; complex curves, internal features, lattices | Limited by tooling, material formability; simple shapes | Enables highly optimized, conformal, integrated designs |
Odlehčení | Significant potential via topology optimization, lattice structures | Limited; often requires thinner material (reduces stiffness) | Substantial weight reduction possible while maintaining or improving performance |
Prototyping Speed | Fast (hours/days); direct from CAD, no tooling | Slow (weeks/months); requires tooling creation/modification | Drastically reduces development time (lead time reduction automotive) |
Náklady na nástroje | Žádný | High (dies and molds) | Economical for prototypes, low-volume, and custom parts |
Přizpůsobení | High; easy to produce unique parts or small batches | Low; economically viable only for mass production | Ideal for niche applications, aftermarket, motorsports, výroba na vyžádání |
Konsolidace částí | High potential; multiple parts integrated into one | Low; often requires assembly of multiple components | Reduces assembly cost, complexity, and potential failure points |
Možnosti materiálu | Wider range, including high-performance alloys (e.g., IN625) | Primarily formable sheet metals (steel, aluminum) | Access to materials with superior thermal/mechanical properties for demanding apps |
Volume Suitability | Prototypes, Low-to-Mid Volume | High Volume | Cost-effective bridge before mass production or for specialized parts |
Export do archů
While metal AM may currently have higher per-part costs for very high volumes compared to stamping, its advantages in design flexibility, performance enhancement, weight reduction, and speed-to-market offer compelling value propositions, particularly for complex heat shields, performance applications, EVs, and situations requiring rapid development or customization. Partnering with a knowledgeable služby kovového 3D tisku provider like Met3dp, which possesses deep expertise in both the tiskových metod and materials, allows automotive companies to fully leverage these benefits for their next-generation thermal management challenges.
Recommended Materials for 3D Printed Heat Shields: AlSi10Mg vs. IN625
Choosing the right material is fundamental to the performance and longevity of any component, and 3D printed automotive heat shields are no exception. The operating environment – specifically the peak and sustained temperatures, potential for corrosion, structural loads, and weight targets – dictates the most suitable metal powder. For many automotive heat shield applications produced via metal additive manufacturing, two materials stand out due to their properties and processability: AlSi10Mg (an aluminum alloy) a IN625 (a nickel-chromium superalloy). Understanding their characteristics is key to selecting the optimal solution.
Met3dp, leveraging its advanced powder manufacturing capabilities including gas atomization and PREP technologies, produces high-quality, spherical metal powder for 3D printing automotive applications, ensuring consistent quality and performance. As a reliable AlSi10Mg powder supplier a IN625 powder distributor, Met3dp offers materials ideally suited for demanding thermal management tasks.
Let’s compare these two workhorse alloys for heat shield applications:
1. Aluminum Alloy – AlSi10Mg:
AlSi10Mg is a widely used aluminum alloy in additive manufacturing, known for its good strength-to-weight ratio, excellent thermal conductivity, good corrosion resistance, and relatively easy processability via laser powder bed fusion (L-PBF) methods like SLM/DMLS.
- Key Properties & Benefits for Heat Shields:
- Low Density (Lightweight): ~2.67 g/cm³. This is a primary advantage for automotive applications where weight reduction is critical for fuel efficiency and performance. Lightweight metal printing is a key driver for adopting AlSi10Mg.
- Vynikající tepelná vodivost: ~120-140 W/(m·K). High thermal conductivity allows the shield to effectively dissipate heat away from protected components or spread it over a larger area, which can be beneficial in certain designs, although for pure insulation, lower conductivity might be desired (achievable via design/coatings).
- Good Strength & Mechanical Properties: Offers decent tensile strength and hardness after appropriate heat treatment, sufficient for many structural requirements of a heat shield.
- Dobrá odolnost proti korozi: Generally performs well in typical automotive environments.
- Zpracovatelnost: Well-understood and widely available material for L-PBF processes, allowing for fine features and good surface finish.
- Efektivita nákladů: Generally more affordable than nickel superalloys like IN625, both in terms of raw powder cost and printing time (due to lower melting point).
- Omezení:
- Moderate Temperature Resistance: This is the main drawback. AlSi10Mg significantly loses strength above approximately 200-250°C (392-482°F). While suitable for heat shields in cooler locations (e.g., further down the exhaust, protecting electronics from moderate heat, some underbody applications, certain EV battery shield scenarios), it is generally not suitable for applications very close to the exhaust manifold, catalytic converter, or turbocharger turbine housing where temperatures routinely exceed 500-600°C (932-1112°F) or higher.
- Typical Heat Shield Applications: Underbody shielding away from primary exhaust heat, shielding for electronics or sensors experiencing moderate heat loads, some EV battery pack applications, brackets or mounting components associated with thermal management systems.
2. Nickel-Chromium Superalloy – IN625 (Inconel® 625):
IN625 is a nickel-based superalloy renowned for its exceptional high-temperature strength, excellent corrosion and oxidation resistance, and outstanding fatigue strength. It’s a go-to material for demanding aerospace and automotive applications involving extreme heat and harsh environments.
- Key Properties & Benefits for Heat Shields:
- Excellent High-Temperature Strength: Maintains significant strength and structural integrity at very high temperatures, up to ~800-900°C (1472-1652°F) or even higher for short excursions, depending on the load. This makes it ideal for shields near the hottest parts of the engine and exhaust. IN625 heat resistance is its defining characteristic.
- Outstanding Corrosion and Oxidation Resistance: Highly resistant to exhaust gases, road salts, and other corrosive elements encountered in automotive environments, ensuring long service life even under harsh conditions.
- Vysoká pevnost a odolnost: Possesses excellent tensile, fatigue, and creep strength across a wide temperature range.
- Weldability/Printability: While more challenging to print than AlSi10Mg (requiring careful parameter control due to residual stresses), IN625 is readily processable with L-PBF and Electron Beam Melting (EBM – a capability area for Met3dp, although L-PBF is more common for heat shields), yielding dense, high-quality parts.
- Omezení:
- Higher Density: ~8.44 g/cm³. Significantly denser and heavier than aluminum alloys. While design optimization (thin walls, lattices) can mitigate this, an IN625 shield will typically be heavier than an AlSi10Mg one of similar dimensions.
- Lower Thermal Conductivity: ~9.8 W/(m·K). This lower conductivity means IN625 is a better insulator than AlSi10Mg, which is often desirable for a heat shield’s primary function of blocking heat transfer.
- Vyšší náklady: Both the raw powder and the printing process are considerably more expensive than for AlSi10Mg due to the high cost of nickel and alloying elements, and the higher energy input and slower build rates required for printing.
- Typical Heat Shield Applications: Exhaust manifold shields, catalytic converter shields, turbocharger shields (turbine side), performance exhaust system components, shields in extremely hot engine bay locations, motorsport applications.
Material Selection Summary Table:
Vlastnosti | AlSi 10Mg | IN625 (Inconel® 625) | Kritéria výběru |
---|---|---|---|
Primary Advantage | Lightweight, Cost-effective, Good Thermal K | Excellent High-Temperature Strength & Corrosion Res. | Primary function driver (Weight vs. Max Temp) |
Hustota | Low (~2.67 g/cm³) | High (~8.44 g/cm³) | Weight sensitivity of the application |
Max Service Temp (Approx.) | ~200-250°C (392-482°F) | ~800-900°C+ (1472-1652°F+) | Peak operating temperature exposure |
Tepelná vodivost | High (~120-140 W/m·K) | Low (~9.8 W/m·K) | Heat dissipation vs. insulation requirement (can be tuned by design) |
Odolnost proti korozi | Dobrý | Vynikající | Severity of the operating environment (exhaust gases, salt) |
Náklady | Dolní | Vyšší | Budget constraints vs. performance requirements |
Typical Use Case | Moderate temp areas, weight-critical shields | Extreme heat areas (exhaust manifold, turbo) | Location within the vehicle |
Dodavatel | Met3dp (AlSi10Mg powder supplier) | Met3dp (IN625 powder distributor) | Ensure high-quality powder source for reliable results |
Export do archů
Why Powder Quality Matters (Met3dp Advantage):
The performance of the final 3D printed heat shield heavily relies on the quality of the metal powder used. Factors like:
- Sféricita: High sphericity ensures good powder flowability in the printer, leading to uniform powder layers and consistent melting. Met3dp’s gas atomization process produces highly spherical powders.
- Distribuce velikosti částic (PSD): A controlled PSD is crucial for achieving high packing density and minimizing porosity in the final part.
- Purity & Chemistry: Strict control over alloy composition and minimizing impurities (like oxygen or nitrogen) is essential for achieving the desired mechanical and thermal properties.
- Absence of Satellites: Fine particles attached to larger ones (satellites) can impede flowability and packing density. PREP technology, also employed by Met3dp, excels at producing satellite-free powders.
Využitím Met3dp metal powders, engineers and manufacturers can be confident they are starting with the best possible foundation for producing durable, high-performance 3D printed heat shields that meet the demanding requirements of the automotive industry. Accessing Met3dp’s comprehensive product range ensures the right material choice for optimal performance.
The choice between AlSi10Mg and IN625 ultimately depends on a careful analysis of the specific application’s thermal loads, environmental conditions, weight targets, and budget. Metal 3D printing provides the flexibility to utilize either material effectively, enabling tailored solutions for optimized automotive thermal management.
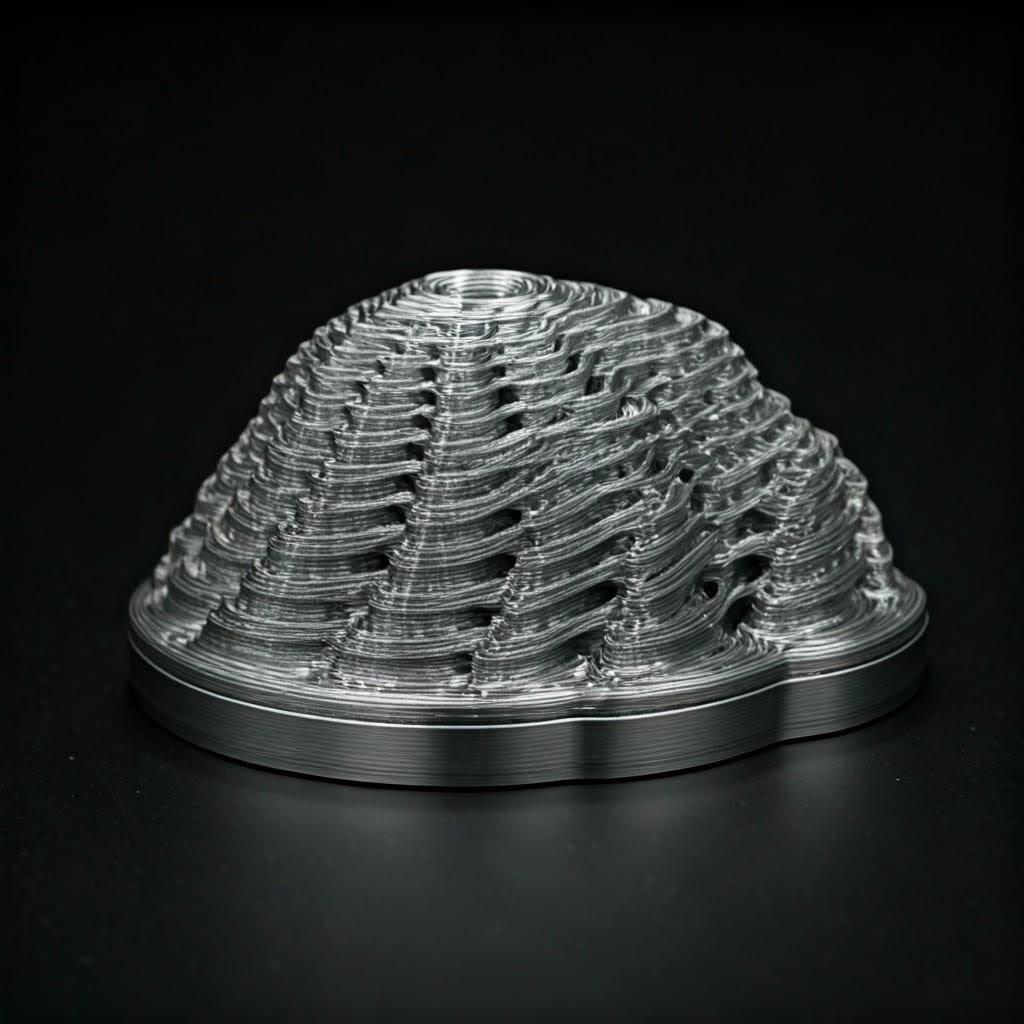
Design Considerations for Additively Manufactured Heat Shields: Optimizing for Printability and Function
One of the most significant advantages of employing metal additive manufacturing for automotive heat shields is the unprecedented design freedom it offers. However, this freedom comes with a responsibility: designers must embrace Design pro aditivní výrobu (DfAM) principles to create parts that are not only functionally superior but also optimized for the printing process itself. Simply transferring a design intended for sheet metal stamping into an AM workflow will likely result in suboptimal outcomes, potentially increasing cost, build time, and the risk of print failures. Effective DfAM for heat shields involves a holistic approach, considering geometry, material properties, support structures, thermal performance, and structural integrity concurrently. Partnering with an experienced metal 3D printing service bureau automotive provider, like Met3dp, who can offer DfAM support, is crucial for success.
Here are key design considerations specifically for 3D printed automotive heat shields:
- Leveraging Geometric Complexity for Performance:
- Conformal Shapes: Design shields that closely follow the contours of the components they protect or the available space within the vehicle chassis. This maximizes coverage and thermal protection efficiency, especially in tightly packaged engine bays or around complex exhaust routing. Metal AM easily produces these freeform shapes, unlike the limitations of stamping dies.
- Integrated Features: Eliminate assembly steps and potential failure points by integrating features directly into the shield design. This includes:
- Mounting Bosses and Brackets: Design attachment points directly onto the shield body.
- Stiffening Ribs: Add complex rib patterns precisely where needed for structural support, minimizing material usage elsewhere. These ribs can also influence airflow and heat dissipation.
- Airflow Channels: Incorporate channels or ducts within or on the surface of the shield to direct cooling air or manage hot gas flow, enhancing thermal performance actively rather than just passively blocking heat.
- Wire/Hose Clips: Integrate clips or routing features for securing nearby components.
- Topology Optimization and Lightweighting:
- Material Reduction: Use Finite Element Analysis (FEA) based topology optimization software to identify areas where material can be removed without compromising the shield’s structural integrity or thermal function. This is a powerful tool for creating lehké automobilové komponenty.
- Lattice and Gyroid Structures: Replace solid sections with internal lattice or gyroid structures. These complex, porous geometries offer several benefits for heat shields:
- Significant Weight Reduction: Dramatically lowers part mass compared to a solid design.
- Enhanced Stiffness-to-Weight Ratio: Can provide excellent structural support with minimal material.
- Improved Thermal Insulation: The trapped air within the lattice/gyroid structure can reduce heat transfer via conduction, potentially enhancing the shield’s insulating properties, especially when using lower conductivity materials like IN625. The specific lattice type (e.g., open vs. closed cell) can be tuned for desired thermal behavior.
- Úvahy o návrhu: Requires careful analysis to ensure manufacturability (powder removal from internal voids) and to understand the impact on thermal conductivity and radiative properties. Minimum strut diameters and node connections must respect the chosen printing process and material capabilities.
- Managing Support Structures:
- Minimizing Supports: Support structures are often necessary in PBF processes to anchor the part to the build plate, support overhanging features (typically angles below 45 degrees from horizontal), and dissipate heat during printing. However, supports add material cost, increase build time, and require post-processing for removal, which can be difficult and leave surface marks (“witness marks”).
- Design for Self-Support: Where possible, design features to be self-supporting. Use chamfers or fillets instead of sharp horizontal overhangs. Orient the part strategically on the build plate to minimize the need for supports on critical or hard-to-reach surfaces. Aim for overhang angles greater than 45 degrees.
- Accessible Supports: When supports are unavoidable, design them for easy access and removal. Avoid complex internal supports in enclosed cavities unless absolutely necessary and planned for (e.g., designing access holes for powder removal and support extraction). Consider frangible support structures that break away more easily.
- Impact on Heat Shields: For thin-walled heat shields, supports are critical for preventing warping and anchoring the part. Careful consideration of support placement is needed to avoid distortion and ensure easy removal without damaging the thin structure.
- Wall Thickness and Feature Size:
- Minimum Wall Thickness: Adhere to minimum printable wall thicknesses for the chosen material (AlSi10Mg or IN625) and AM process. This typically ranges from 0.4mm to 1.0mm, but depends on the specific machine, parameters, and unsupported span length. Thinner walls reduce weight and material cost but can be more prone to warping or damage during handling and post-processing.
- Jednotnost: While AM allows variable thickness, sudden large changes in cross-section can lead to differential cooling and increased residual stress. Aim for smooth transitions where thickness changes are necessary.
- Small Features: Consider the minimum resolvable feature size (holes, slots, pins) for the process, which is usually around 0.3-0.5mm.
- Thermal Considerations in Design:
- Heat Flow Path: Consciously design the shield to manage heat flow. Use geometry to direct heat away from sensitive areas. Consider how integrated ribs or features might act as cooling fins or heat sinks, which may or may not be desirable depending on the goal.
- Surface Properties: Recognize that the as-built surface roughness can influence radiative heat transfer. While often addressed in post-processing (e.g., coatings), initial design choices can impact the final surface characteristics.
- Thermal Simulation: Utilize thermal simulation software early in the design phase (thermal simulation heat shield) to predict temperature distributions, heat flux, and the effectiveness of the proposed shield design. This allows for iterative optimization before committing to printing, saving time and cost. Simulation can help optimize rib placement, wall thickness variations, and the effectiveness of airflow channels or lattice structures for thermal insulation.
DfAM Summary Table for Heat Shields:
DfAM Principle | Application to Heat Shields | Key Benefit(s) |
---|---|---|
Geometrická svoboda | Conformal shapes, integrated mounts/ribs/channels | Improved fit, reduced part count, enhanced thermal/structural performance |
Optimalizace topologie | FEA-driven material removal | Maximum lightweighting, optimized stiffness |
Mřížové struktury | Replacing solid sections with internal lattices/gyroids | Drastic weight reduction, potential for improved insulation, high stiffness |
Support Minimization | Strategic orientation, self-supporting angles (>45°), fillets/chamfers | Reduced print time/cost, easier post-processing, better surface finish |
Accessible Supports | Designing supports for easy removal, avoiding trapped internal supports | Reduced post-processing labor/risk of damage |
Tloušťka stěny | Respecting minimums (0.4-1.0mm+), smooth transitions | Printability, structural integrity, controlled weight |
Thermal Design | Directing heat flow, considering surface effects, using simulation | Optimized thermal protection, predictable performance |
Export do archů
By thoughtfully applying these DfAM principles, engineers can fully exploit the capabilities of metal additive manufacturing to produce automotive heat shields that are lighter, more effective, and faster to develop than their traditionally manufactured counterparts. Collaboration with AM experts, such as the team at Met3dp, during the design phase can provide invaluable insights into optimizing parts for both function and manufacturability.
Achievable Tolerance, Surface Finish, and Dimensional Accuracy in Metal 3D Printed Heat Shields
When specifying components for automotive applications, engineers and procurement managers rightly focus on precision. Questions regarding metal 3D printing tolerances, achievable surface roughness metal AMa celkově dimensional accuracy automotive parts are critical. While metal AM offers incredible design freedom, it’s important to understand the levels of precision inherent in the process and how they compare to conventional methods like machining or stamping. For automotive heat shields, the required precision varies depending on the specific feature – mounting points might need tighter tolerances than large, open surfaces, for instance.
Rozměrová přesnost a tolerance:
Metal Powder Bed Fusion (PBF) processes like SLM/DMLS can achieve relatively good dimensional accuracy directly from the machine. However, several factors influence the final part accuracy:
- Kalibrace stroje: Regular and precise calibration of the printer’s lasers/electron beam, scanners, and motion systems is crucial.
- Vlastnosti materiálu: Different materials shrink and warp differently upon cooling (e.g., AlSi10Mg vs. IN625). Process parameters must be tuned accordingly.
- Thermal Stresses: The rapid heating and cooling inherent in PBF create internal stresses that can cause warping or distortion, affecting accuracy. Part design, orientation, and support strategy play a huge role in managing this.
- Part Size and Geometry: Larger parts or complex geometries with varying cross-sections are generally more challenging to print with high accuracy due to accumulated thermal stress.
- Build Parameters: Layer thickness, laser power, scan speed, etc., all influence the final dimensions.
Typical Tolerances:
- As-Built Tolerances: For well-controlled L-PBF processes, typical achievable tolerances are often cited in the range of:
- ±0.1 mm to ±0.2 mm for smaller features (e.g., up to 20-50 mm)
- ±0.1% to ±0.2% of the nominal dimension for larger features.
- Industry standards like ISO/ASTM 52900 series provide frameworks, but specific machine/material capabilities often dictate achievable limits.
- Post-Processed Tolerances: If tighter tolerances are required, especially for mating surfaces, mounting holes, or critical interfaces on the heat shield, post-process CNC machining is typically employed. Machining can achieve tolerances of ±0.025 mm to ±0.05 mm or even tighter, comparable to traditional manufacturing.
Povrchová úprava (drsnost):
The surface finish of as-built metal AM parts is inherently rougher than machined or even stamped surfaces. This is due to the layer-by-layer nature of the process and the partially melted powder particles adhering to the surface.
- Factors Influencing Roughness:
- Tloušťka vrstvy: Thicker layers generally result in rougher surfaces.
- Velikost částic prášku: Finer powders can lead to smoother finishes but may pose challenges in flowability. Met3dp’s focus on optimized PSD helps balance this.
- Orientace: Surfaces parallel to the build plate (top surfaces) are typically smoother than vertical walls. Downward-facing surfaces (supported or near-horizontal) tend to be the roughest due to support contact or the “stair-stepping” effect. Upward-facing curved surfaces generally have better finishes than downward-facing ones.
- Build Parameters: Laser/beam energy, scan strategy, and gas flow (in L-PBF) affect melt pool dynamics and surface consolidation.
- Typical Ra Values (As-Built):
- AlSi10Mg (L-PBF): Ra values typically range from 6 µm to 15 µm (240 µin to 600 µin).
- IN625 (L-PBF): Ra values often range from 8 µm to 20 µm (320 µin to 800 µin).
- These values are highly dependent on orientation and process specifics.
- Zlepšení povrchové úpravy: For heat shields, the as-built finish might be acceptable for non-critical surfaces, but smoother finishes are often desired for aesthetics, improved fatigue life (less crack initiation sites), or preparation for coatings. Common post-processing surface finish techniques include:
- Tryskání kuličkami / kuličkování: Creates a uniform matte finish, removes loose powder, and can impart beneficial compressive stresses. Typical Ra might improve to 3-8 µm.
- Obrábění / vibrační úprava: Uses media to smooth surfaces and edges, particularly effective for batches of smaller parts. Can achieve Ra values down to 1-3 µm.
- CNC obrábění: Provides the smoothest finishes on specific features but only where tool access is possible.
- Electropolishing: Can achieve very smooth, mirror-like finishes, especially on materials like IN625, but requires specific electrolytes and part geometry considerations.
Quality Control and Met3dp’s Commitment:
Achieving consistent dimensional accuracy and surface finish requires rigorous quality control metal printing procedures. This includes:
- Regular machine maintenance and calibration.
- Strict control over powder quality and handling (Met3dp’s core strength).
- Optimized process parameter development for each material (AlSi10Mg, IN625).
- In-process monitoring where available.
- Post-build inspection using techniques like CMM (Coordinate Measuring Machines), 3D scanning, and surface profilometry.
Met3dp is committed to delivering parts with industry-leading accuracy and reliability. Our investment in advanced printing technology and high-quality powder production ensures that customers receive heat shields meeting their specifications. We work closely with clients to understand tolerance requirements and advise on the best approach, whether relying on as-built accuracy or incorporating secondary machining operations.
Summary Table: Accuracy & Finish in Metal AM Heat Shields
Parametr | Typical As-Built Range (L-PBF) | Influencing Factors | Možnosti dodatečného zpracování | Relevance to Heat Shields |
---|---|---|---|---|
Rozměrová tolerance | ±0.1-0.2mm or ±0.1-0.2% | Machine, Material, Thermal Stress, Size, Parameters | CNC obrábění | Critical for mounting points, interfaces; less critical for open surfaces |
Drsnost povrchu (Ra) | AlSi10Mg: 6-15 µm; IN625: 8-20 µm | Layer Thickness, Powder Size, Orientation, Parameters | Blasting, Tumbling, Machining | Affects aesthetics, coating adhesion, potentially fatigue/airflow |
Control Method | Calibration, Parameter Opt., QC checks | Design (DfAM), Process Control, Material Quality (Met3dp) | Secondary Operations | Ensures parts meet engineering specifications consistently |
Export do archů
Understanding these achievable metrics allows engineers to design heat shields appropriately and set realistic expectations. While metal AM might not match the finest tolerances of precision machining directly off the build plate, its combination of design freedom with respectable accuracy, often enhanced by targeted post-processing, makes it a powerful tool for creating effective and complex automotive thermal solutions.
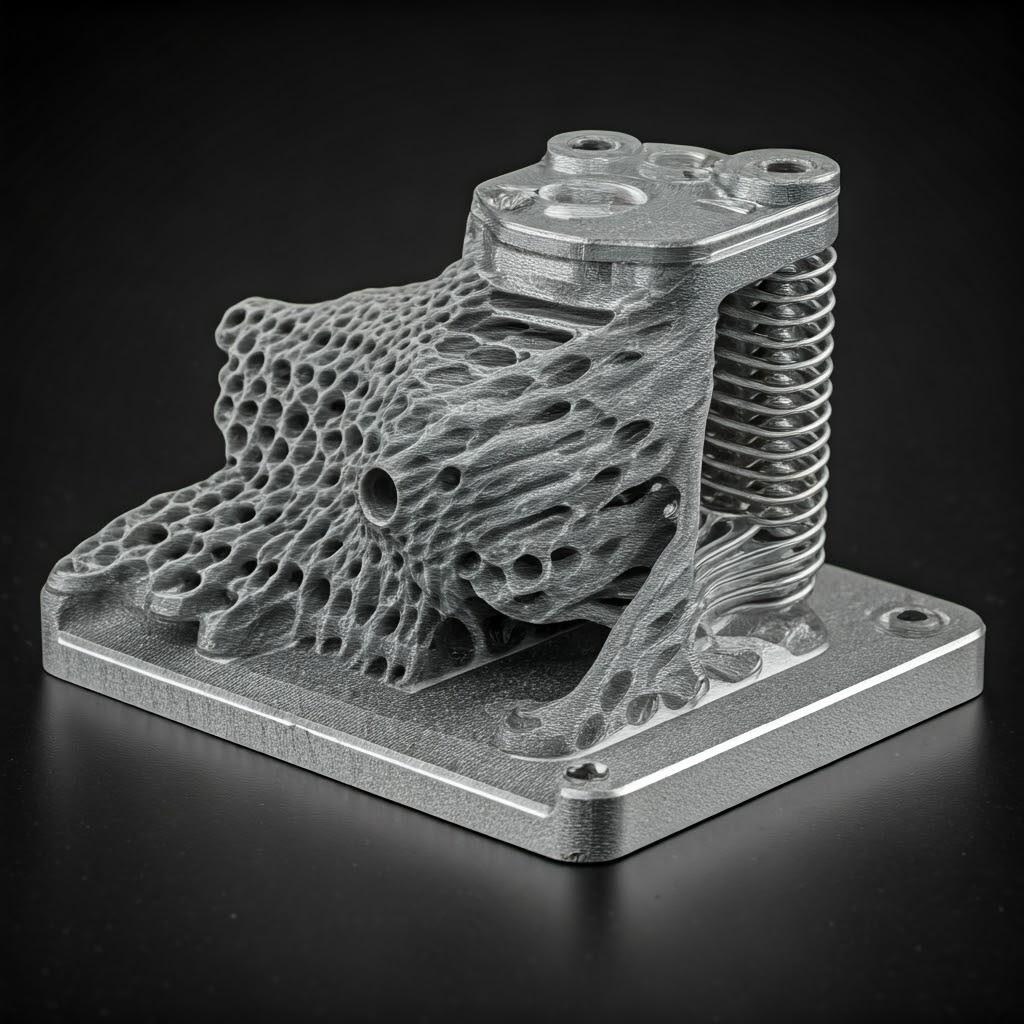
Post-Processing Requirements for 3D Printed Automotive Heat Shields
Creating a functional automotive heat shield using metal 3D printing doesn’t end when the printer stops. The as-built part, fresh off the build plate, requires several crucial následné zpracování steps to achieve the final desired properties, dimensions, surface finish, and ultimately, its fitness for purpose in a demanding automotive environment. These steps are essential for ensuring the reliability, durability, and performance of the component, whether it’s made from AlSi10Mg or the high-temperature capable IN625. Failing to execute these steps correctly can compromise the integrity of the heat shield.
Common post-processing requirements include:
- Úleva od stresu / tepelné ošetření:
- Why: This is arguably the most critical post-processing step for PBF metal parts. The rapid heating and cooling during printing induce significant residual stresses within the material. These stresses can cause warping or distortion (especially after removal from the build plate), reduce fatigue life, and even lead to cracking over time or during subsequent machining. Heat treatment metal 3D printing is essential to relax these stresses and stabilize the microstructure. Additionally, for alloys like AlSi10Mg, heat treatment (e.g., T6 solution treatment and aging) is required to achieve the optimal mechanical properties (strength, hardness). IN625 typically undergoes stress relief or annealing to improve ductility and relieve stress without necessarily targeting precipitation hardening.
- How: Parts are placed in a controlled atmosphere furnace (vacuum or inert gas like argon to prevent oxidation, especially critical for IN625 at high temperatures) and subjected to specific thermal cycles (heating rate, soak temperature, soak time, cooling rate). The exact cycle depends heavily on the alloy and the desired final properties.
- AlSi10Mg: Often involves a solution treatment (e.g., ~530°C) followed by quenching and artificial aging (e.g., ~160°C) for T6 condition. Simple stress relief might involve lower temperatures (~300°C).
- IN625: Stress relief typically occurs at higher temperatures, often in the range of 870°C to 980°C, followed by controlled cooling. Annealing might occur at even higher temperatures (~1100°C+).
- Úvahy: Heat treatment must often be performed before removing the part from the build plate, especially for large or thin-walled parts prone to warping, like many heat shield designs. The furnace atmosphere must be carefully controlled.
- Part Removal from Build Plate:
- Why: The part is fused to a thick metal build plate during printing.
- How: Typically done using wire EDM (Electrical Discharge Machining) or a bandsaw. Care must be taken not to damage the part during this process. Wire EDM is often preferred for precision and minimal mechanical stress induction.
- Odstranění podpůrné konstrukce:
- Why: Supports, while necessary for printing, are not part of the final component and must be removed.
- How: This can be a labor-intensive process depending on the complexity and location of the supports. Methods include:
- Manual Breaking/Cutting: For accessible supports, pliers, cutters, or small grinders can be used. Requires skill to avoid damaging the part surface.
- CNC obrábění: Milling or grinding can remove support structures precisely, especially contact points (“witness marks”).
- Wire EDM: Can be used for intricate or hard-to-reach supports if access allows.
- Úvahy: Support removal AM parts is a key area where DfAM plays a role – designing for easy support removal significantly reduces post-processing time and cost. Witness marks left after removal may require further finishing.
- CNC Machining (If Required):
- Why: To achieve tighter tolerances than as-built capabilities allow on critical features (e.g., mounting holes, mating surfaces, sealing faces). Also used to improve surface finish on specific areas.
- How: Standard CNC machining 3D prints techniques (milling, turning, drilling) are used. Fixturing the complex geometry of a 3D printed part can sometimes be challenging. Machining is typically performed po heat treatment to ensure dimensional stability.
- Úvahy: Must account for material properties (AlSi10Mg is relatively easy to machine; IN625 is notoriously difficult due to work hardening and toughness, requiring specific tooling, speeds, and feeds). DfAM should include adding extra stock material (machining allowance) on surfaces intended for machining.
- Povrchová úprava:
- Why: To improve aesthetics, remove loose powder particles, achieve a uniform appearance, prepare for coatings, or sometimes improve fatigue life or airflow characteristics.
- How: Různé surface finishing techniques can be applied:
- Bead Blasting/Shot Peening: Common for a uniform matte finish. Different media (glass beads, ceramic, steel shot) produce different effects. Peening induces beneficial compressive stress.
- Tumbling/Vibratory Finishing: Good for deburring edges and smoothing surfaces on batches of parts.
- Grinding/Polishing: Manual or automated processes for achieving smoother, brighter finishes on specific areas.
- Electropolishing: Electrochemical process for very smooth, clean surfaces, particularly effective on stainless steels and nickel alloys like IN625.
- Úvahy: The chosen method depends on the desired finish, part geometry (access), material, and cost. Aggressive methods can remove small amounts of material, affecting final dimensions.
- Cleaning and Inspection:
- Why: To remove any residual powder (especially from internal channels or lattice structures), machining fluids, or blasting media. Final inspection ensures the part meets all dimensional, surface finish, and quality requirements.
- How: Ultrasonic cleaning, compressed air blow-off. Inspection methods include visual checks, CMM, 3D scanning, profilometry, and potentially NDT (Non-Destructive Testing) like CT scanning for internal integrity if required.
- Povlak (volitelný):
- Why: To enhance specific properties, particularly thermal insulation or reflection. Coating 3D printed parts is common for performance optimization.
- How: For heat shields, Thermal Barrier Coatings (TBCs) are often considered. These are typically ceramic-based coatings (e.g., Yttria-Stabilized Zirconia – YSZ) applied via plasma spray or other techniques. They create a layer with very low thermal conductivity, significantly reducing heat transfer through the shield. Reflective coatings (e.g., ceramic-metallic or specialized paints) can also be used to reflect radiant heat.
- Úvahy: Surface preparation is critical for good coating adhesion. The coating adds thickness and may have temperature limitations itself.
Post-Processing Workflow Summary:
The typical sequence (though variations exist) is:
- Stress Relief / Heat Treatment (often on build plate)
- Removal from Build Plate (Wire EDM / Saw)
- Support Removal (Manual / Machining)
- CNC Machining (if required for tolerances/features)
- Surface Finishing (Blasting, Tumbling, etc.)
- Cleaning & Inspection
- Coating (if required)
Understanding these necessary automotive parts finishing standards and steps is crucial for accurately estimating the total cost and lead time for 3D printed heat shields. Each step adds time and expense, highlighting the importance of optimizing the design (DfAM) to minimize post-processing requirements where possible, particularly support removal and machining.
Common Challenges in 3D Printing Heat Shields and How to Mitigate Them
While metal additive manufacturing offers significant advantages for producing custom automotive heat shields, the process is not without its potential hurdles. Understanding these metal AM challenges automotive applications face, specifically in the context of heat shields made from AlSi10Mg or IN625, allows engineers and manufacturers to proactively implement mitigation strategies, ensuring successful outcomes and reliable components. Partnering with an experienced service provider is key to navigating these complexities.
Here are some common challenges and how to address them:
- Warping and Distortion (Residual Stress):
- Výzva: The high temperatures and rapid cooling inherent in PBF generate significant residual stresses within the printed part. As the part builds, these stresses can cause it to warp, curl away from the build plate, or distort, especially with thin-walled, large-area geometries typical of heat shields. This residual stress management issue is more pronounced with materials having higher thermal expansion coefficients or requiring higher processing temperatures (like IN625).
- Mitigation:
- DfAM: Design features to minimize stress concentration. Use fillets instead of sharp corners. Aim for more uniform wall thicknesses or gradual transitions.
- Orientace: Strategically orient the part on the build plate to minimize large flat areas parallel to the plate and reduce the height-to-width ratio where possible.
- Support Strategy: Robust support structures are crucial to anchor the part firmly to the build plate and act as heat sinks. Careful placement and density are key.
- Build Plate Heating: Pre-heating the build plate (common in many L-PBF machines, essential in EBM) reduces the thermal gradient and lowers residual stress.
- Parametry procesu: Optimized parameters (scan strategy, laser power, speed) developed for the specific material and geometry can minimize stress buildup.
- Tepelné zpracování: Performing stress relief heat treatment before removing the part from the build plate is often the most effective way to prevent distortion upon release.
- Support Removal Difficulties:
- Výzva: Heat shields often feature complex curves and potentially internal channels or cavities. Placing supports in these hard-to-reach areas makes removal difficult, time-consuming, and risks damaging the part. In lattice structures, removing internal supports can be nearly impossible if not planned for.
- Mitigation:
- DfAM for Access: Design the part with support removal in mind. Orient to minimize internal supports. If internal supports are needed (e.g., for internal channels in a lattice), design access ports for removal tools or powder evacuation.
- Support Types: Utilize optimized support structures (e.g., thin-walled, block, or cone supports with specific contact points) designed for easier detachment. Some software allows for generating easily breakable or soluble supports (less common in metal AM).
- Removal Techniques: Employ appropriate techniques like careful manual breaking, CNC machining, or wire EDM for support removal, depending on accessibility and precision requirements.
- Pórovitost:
- Výzva: Small voids or pores within the printed material (porosity metal AM) can compromise mechanical properties (especially fatigue strength) and potentially create leak paths if the shield needs to be sealed. Porosity can arise from trapped gas, incomplete fusion between layers, or keyholing (vapor depressions collapsing).
- Mitigation:
- Vysoce kvalitní prášek: Using high-quality, spherical powder with controlled PSD and low gas content, like those produced by Met3dp using advanced atomization techniques, is fundamental. Poor powder quality is a common source of porosity.
- Optimalizované parametry: Developing and strictly controlling print parameters (laser power, scan speed, hatch spacing, layer thickness, gas flow) is critical to ensure complete melting and fusion.
- Machine Condition: Ensuring the printer is well-maintained and the build chamber atmosphere (inert gas quality) is controlled prevents contamination and promotes stable melting.
- Izostatické lisování za tepla (HIP): For critical applications requiring near-zero porosity, HIP can be used as a post-processing step. It involves applying high temperature and isostatic gas pressure to close internal voids. This adds significant cost and time but yields fully dense parts.
- Surface Finish and Defects:
- Výzva: Achieving the desired surface finish, especially on downward-facing surfaces or complex internal features, can be difficult. Defects like “stair-stepping” on shallow angles, dross formation, or partially melted particles adhering to the surface (“spatter”) can occur.
- Mitigation:
- Orientace: Optimize part orientation to place critical surfaces in upward-facing or vertical positions where possible.
- Parameters: Fine-tune contour parameters and potentially use specific scan strategies for boundary definition.
- Následné zpracování: Rely on surface finishing techniques (blasting, tumbling, polishing) to achieve the required final finish after addressing major support witness marks.
- Kvalita prášku: High-quality powder reduces spatter and promotes smoother melt pool behavior.
- Achieving Internal Channel Requirements:
- Výzva: If a heat shield design incorporates internal channels for cooling or structural purposes (e.g., in lattice structures), ensuring these channels are free of powder and have an acceptable surface finish can be challenging. Powder removal can be difficult from tortuous paths, and internal surfaces are inherently rougher.
- Mitigation:
- DfAM: Design channels with sufficient diameter and smooth bends to facilitate powder removal. Include strategically placed drainage/access holes. Consider designing channels that are self-supporting to minimize internal supports.
- Powder Removal: Utilize vibration, compressed air, and potentially specialized powder removal stations post-build.
- Process Choice: Some processes might be better suited for internal channels (e.g., EBM often produces less tightly packed powder).
- Inspection: CT scanning may be necessary to verify complete powder removal and internal integrity.
- Ensuring Consistency and Quality Assurance:
- Výzva: Maintaining consistent part quality (dimensional accuracy, material properties, defect levels) across different builds and different machines requires robust quality assurance additive manufacturing protocols.
- Mitigation:
- Standardized Procedures: Implement strict process controls, standardized build parameters, and rigorous machine maintenance schedules.
- Material Traceability: Maintain full traceability of powder batches used for each build. Regularly test powder quality.
- Monitorování během procesu: Utilize available melt pool monitoring or layer imaging tools to detect potential anomalies during the build.
- Post-Build Qualification: Implement consistent inspection protocols (CMM, scanning, NDT) and mechanical property testing (using witness coupons printed alongside the parts) to verify quality.
Addressing these challenges requires a combination of smart design (DfAM), careful process control, high-quality materials, appropriate post-processing, and rigorous quality assurance. Partnering with a vertically integrated and experienced provider like Met3dp, which controls everything from powder production to final part qualification, significantly minimizes these risks. Their deep understanding of materials science, printing processes, and post-processing intricacies ensures that potential issues are anticipated and addressed proactively. For companies seeking reliable custom 3D printed heat shields, choosing a partner with proven expertise is paramount to navigating the complexities of metal AM. You can learn more about Met3dp’s comprehensive capabilities and commitment to quality across their full range of services by visiting their website https://met3dp.com/.
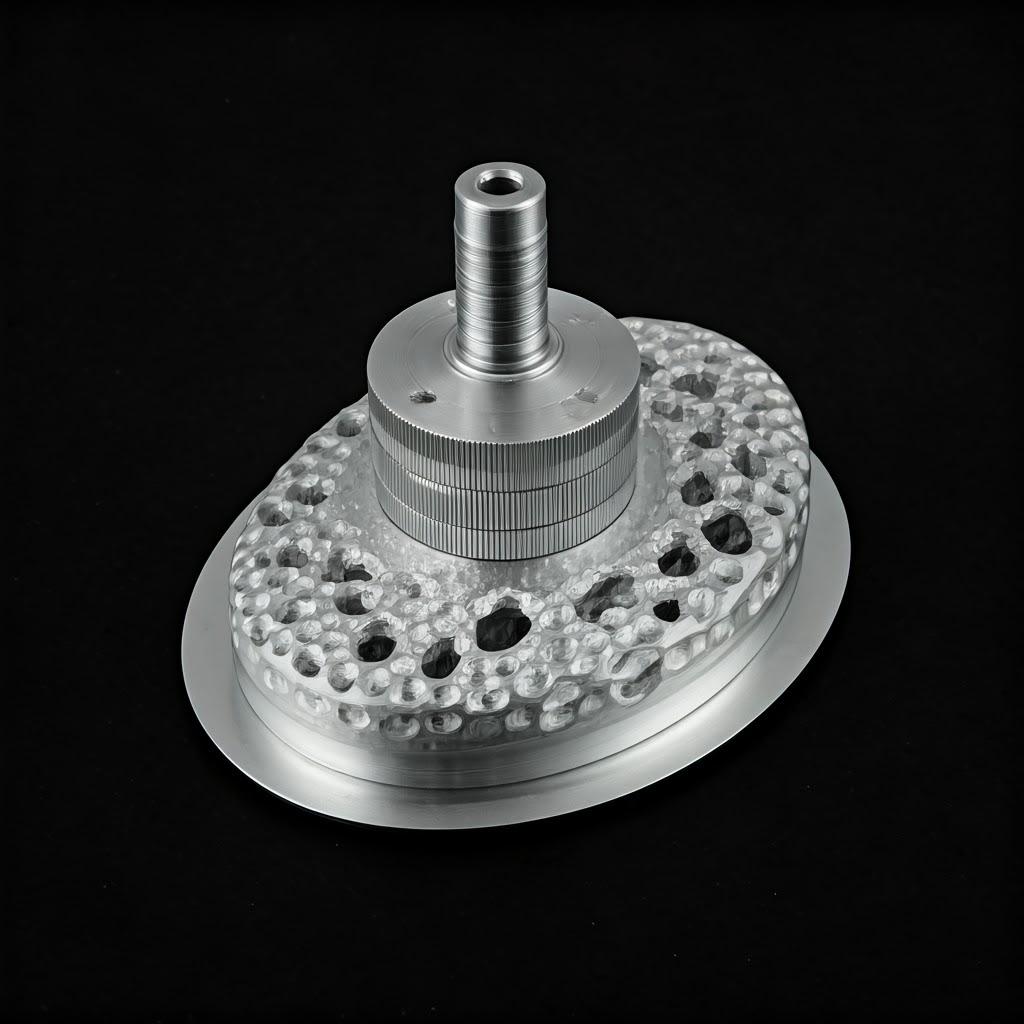
How to Choose the Right Metal 3D Printing Service Provider for Automotive Heat Shields
Selecting the right manufacturing partner is as critical as perfecting the design itself, especially when venturing into advanced technologies like metal additive manufacturing for demanding automotive applications such as heat shields. The quality, reliability, and performance of your final component depend heavily on the capabilities, expertise, and quality systems of your chosen metal 3D printing service bureau automotive provider. For engineers and procurement managers navigating this landscape, evaluating potential suppliers requires looking beyond just price and considering a range of technical and operational factors. Finding a true heat shield manufacturing expert who understands both AM and the nuances of automotive requirements is key.
Here’s a comprehensive guide on what to look for when choosing a metal AM partner for your custom heat shield project:
- Technical Expertise and Material Capabilities:
- Relevant Materials: Ensure the provider has proven experience printing with the specific materials required for your heat shield – primarily AlSi 10Mg a IN625. Ask for evidence of successful prints, material datasheets based on their process, and potentially sample parts. They should understand the unique challenges and optimal parameters for each alloy.
- Process Proficiency: Verify their expertise in the appropriate AM technology, most commonly Laser Powder Bed Fusion (L-PBF, including SLM/DMLS) for heat shields due to its ability to produce fine features and complex geometries. If Electron Beam Melting (EBM) is considered (perhaps for specific IN625 applications), ensure they possess that capability.
- Engineering Support (DfAM): Does the provider offer Design for Additive Manufacturing (DfAM) consultation? A good partner will work with your engineering team to optimize the heat shield design for printability, functionality, cost-effectiveness, and minimized post-processing. This collaborative approach is invaluable.
- Quality Management System (QMS) and Certifications:
- ISO 9001: This is a fundamental requirement, indicating a robust QMS for general manufacturing quality.
- Automotive Standards (IATF 16949): While not all AM service bureaus hold the automotive-specific IATF 16949 certification (it’s rigorous and often geared towards higher volume production), inquire about their familiarity with automotive requirements and whether their QMS incorporates relevant principles (e.g., APQP, PPAP, traceability). Providers serving the aerospace industry (e.g., holding AS9100) often have highly stringent quality systems applicable to demanding automotive needs.
- Traceability: The provider must demonstrate full material traceability – from the raw powder batch (AlSi10Mg powder supplier, IN625 powder distributor) through printing, post-processing, and final inspection. This is non-negotiable for critical automotive parts. Met3dp, manufacturing its own powders, offers exceptional control over this aspect.
- Zkušenosti v oboru:
- Automotive Track Record: Has the provider worked on automotive projects before, specifically thermal management components? Experience with the demanding requirements, validation processes, and common challenges of the automotive sector is a significant advantage. Ask for relevant case studies or references (respecting confidentiality agreements).
- Understanding Applications: Do they understand the functional requirements of a heat shield – thermal insulation/reflection, vibration resistance, durability, mounting constraints?
- Equipment and Facilities:
- Machine Park: Assess their range and number of industrial-grade metal AM printers. Do they have sufficient AM production capacity to handle your prototyping needs and potentially scale to low-to-mid volume production? Are the machines well-maintained and calibrated?
- Manipulace s práškem: Evaluate their procedures for powder storage, handling, recycling, and quality testing to prevent contamination and ensure material consistency. Providers like Met3dp, who manufacture their own powders using advanced methods like gas atomization and PREP, demonstrate superior control over this critical input.
- Post-Processing Capabilities: Do they offer essential post-processing steps in-house (heat treatment, support removal, basic finishing)? Or do they rely on outsourcing? In-house capabilities generally allow for better control over quality, lead times, and communication. Verify their expertise in heat treating AlSi10Mg and IN625 correctly and safely removing supports from complex geometries. Assess their capabilities for CNC machining or specialized surface finishing if required.
- Řízení projektů a komunikace:
- Clear Communication: Is the provider responsive, transparent, and easy to communicate with? Establish clear points of contact and reporting structures.
- Project Management: Do they have a structured process for managing projects, from quotation and design review to production scheduling, quality checks, and delivery?
- Quotation Detail: Review their quotation process. Does it clearly break down costs (material, machine time, labor, post-processing)? Does it specify lead times and delivery terms?
- Cost and Lead Time:
- Konkurenční ceny: While cost shouldn’t be the pouze factor, obtain quotes from multiple qualified suppliers. Ensure you are comparing like-for-like in terms of material, quality standards, and included post-processing.
- Realistic Lead Times: Discuss lead times for prototypes versus production runs. Ensure the provider has a track record of meeting delivery commitments. Understand factors that influence lead time (machine availability, post-processing complexity).
Evaluating Potential Suppliers: Key Questions to Ask
- Can you provide examples of similar heat shields or complex automotive components you have printed using AlSi10Mg / IN625?
- What is your process for ensuring material quality and traceability? Where do you source your powders? (Met3dp’s answer here highlights their vertical integration).
- What DfAM support can you offer for optimizing our heat shield design?
- What are your standard dimensional tolerances and achievable surface finishes for these materials as-built?
- Describe your in-house post-processing capabilities (heat treatment, machining, finishing).
- What quality certifications do you hold (ISO 9001, IATF 16949, AS9100)? Can you accommodate PPAP requirements if needed?
- What is your typical lead time for a prototype heat shield of this complexity? For a batch of X units?
- How do you manage quality control throughout the production process? What inspection methods do you use?
Why Consider Met3dp?
Met3dp stands out as a potential partner by addressing many of these critical selection criteria:
- Vertical Integration: As a manufacturer of both advanced metal AM printers and high-quality spherical metal powders (including AlSi10Mg, IN625, and innovative alloys), Met3dp offers unparalleled control over the entire process chain.
- Materiálové znalosti: Deep understanding of powder metallurgy and material behavior during AM ensures optimal results.
- Pokročilé technologie: Utilization of industry-leading printing equipment and powder production techniques (Gas Atomization, PREP).
- Komplexní řešení: Offering printers, powders, and application development services provides a holistic approach.
- Industry Focus: Experience serving demanding industries like aerospace, medical, and automotive.
Výběr správného AM supplier is a strategic decision. By carefully evaluating potential partners against these criteria, automotive companies can forge strong relationships that enable them to successfully leverage the power of metal 3D printing for innovative and high-performance heat shield solutions.
Cost Factors and Lead Time for Custom 3D Printed Heat Shields
While the technical benefits of metal 3D printing for automotive heat shields are compelling, understanding the associated metal 3D printing cost automotive factors and typical production timelines is crucial for project planning, budgeting, and comparing AM against traditional methods. The final price and delivery schedule for a custom 3D printed heat shield depend on a complex interplay of design choices, material selection, processing steps, and order volume.
Key Cost Drivers:
- Material Type and Volume:
- Material Choice: This is often a primary cost driver. High-performance nickel superalloys like IN625 are significantly more expensive per kilogram than aluminum alloys like AlSi 10Mg, due to raw material costs and processing difficulty.
- Part Volume/Mass: The sheer amount of metal powder consumed directly impacts cost. Larger, denser parts naturally cost more. DfAM techniques like topology optimization and lattice structures are key to minimizing material usage while maintaining function.
- Machine Time (Build Time):
- Part Size (Bounding Box): Larger parts occupy the build chamber for longer periods. The overall height of the part is particularly influential, as printing is layer by layer.
- Část Složitost: Complex geometries might require slower print speeds or more intricate scanning strategies, increasing machine time.
- Podpůrné struktury: The volume of support material needed adds to both material consumption and build time, as these structures also need to be printed. Optimized orientation and DfAM help reduce support needs.
- Nesting/Batching: Printing multiple parts simultaneously in one build (nesting) can significantly reduce the effective machine time cost per part by maximizing machine utilization. This is key for achieving better economics in velkoobchodní služby 3D tisku or small series production.
- Labor and Setup:
- File Preparation: Preparing the CAD file for printing (orientation, support generation, slicing) requires skilled labor.
- Machine Setup/Teardown: Loading powder, setting up the build, and then removing parts and cleaning the machine involve labor time. These setup costs are amortized over the number of parts in a build; hence, single prototypes have a higher per-part setup cost than batch production.
- Práce po zpracování: This can be a significant cost component. Manual support removal, machining setup and operation, finishing, and inspection all require skilled technician time. The complexity of the part and its post-processing requirements directly influence this cost.
- Post-Processing Intensity:
- Tepelné zpracování: Required for virtually all metal AM parts, the cost depends on the furnace cycle time, atmosphere requirements (vacuum/inert gas is more expensive), and batch size.
- Odstranění podpory: Complex or inaccessible supports drive up labor costs.
- CNC obrábění: If tight tolerances or specific finishes are needed on certain features, machining adds significant cost due to machine time, tooling, programming, and labor. Machining IN625 is notably more expensive than machining AlSi10Mg.
- Povrchová úprava: Costs vary widely depending on the method (simple bead blast vs. multi-step polishing or electropolishing) and the required quality level.
- Povlaky: Applying TBCs or other coatings adds material and process costs.
- Quality Assurance and Inspection:
- Basic QA: Standard dimensional checks and visual inspection are typically included.
- Advanced QA: Requiring CMM reports, 3D scanning comparisons, NDT (e.g., CT scanning for internal porosity), or material property testing (tensile tests on witness coupons) adds cost but may be necessary for critical applications. PPAP documentation also incurs costs.
- Objednávkové množství:
- Prototypy: Single parts or very small batches have the highest per-part cost due to setup costs not being amortized.
- Low-to-Mid Volume: As quantities increase (e.g., dozens to hundreds), per-part costs decrease due to economies of scale in setup, batch processing (printing, heat treatment, finishing), and potential for negotiated velkoobchodní služby 3D tisku rates.
- High Volume: For very high volumes (thousands or tens of thousands), traditional methods like stamping usually become more cost-effective for simpler heat shield designs. However, for highly complex or customized shields, AM might remain competitive even at higher volumes than initially expected.
Lead Time Factors:
Lead time refers to the total time from order placement to part delivery. For heat shield production time using metal AM, typical factors include:
- Quotation and Design Review: Initial communication, file checks, DfAM consultation (if needed), and quote generation take time (typically days).
- Queue Time / Machine Availability: Service bureaus operate on schedules. Your job must wait for an available machine appropriately configured for your material. This can range from days to weeks, depending on demand.
- Print Time: The actual time the part spends printing in the machine. Can range from hours for small parts to several days for large, complex parts or full build plates.
- Cooling Time: Parts need to cool down within the machine or after removal before handling.
- Následné zpracování: This often constitutes a significant portion of the total lead time.
- Heat treatment cycles can take 8-24 hours or more (including ramp-up/cool-down).
- Support removal and basic finishing might take hours to days depending on complexity.
- CNC machining adds significant time for programming, setup, and machining cycles (hours/days).
- Outsourced steps (e.g., specialized coatings, HIP) add transit time and the external vendor’s lead time.
- Kontrola kvality: Time required depends on the level of inspection needed.
- Doprava: Transit time to your facility.
Typical Lead Time Estimates:
- Prototypy: Often 5-15 business days, depending heavily on complexity, post-processing, and current machine queues. Expedited services may be available at a premium.
- Low-Volume Production (e.g., 10-100 units): Typically 3-6 weeks, allowing for batch processing and scheduling efficiencies.
- Higher Volumes: Lead times scale with quantity and complexity, requiring careful planning and potentially dedicated machine time.
Lead Time Reduction Strategies:
- DfAM: Simplifying design and minimizing supports reduces print and post-processing time.
- Clear Specifications: Providing complete and accurate requirements upfront avoids delays.
- Partner Communication: Working closely with a responsive service provider like Met3dp helps streamline the process.
- Realistic Expectations: Understand that metal AM, especially with extensive post-processing, is not typically an overnight process for complex parts.
By understanding these cost and lead time dynamics, engineers and procurement managers can make informed decisions about utilizing metal AM for automotive heat shields, balancing the technical advantages against budget and schedule constraints.
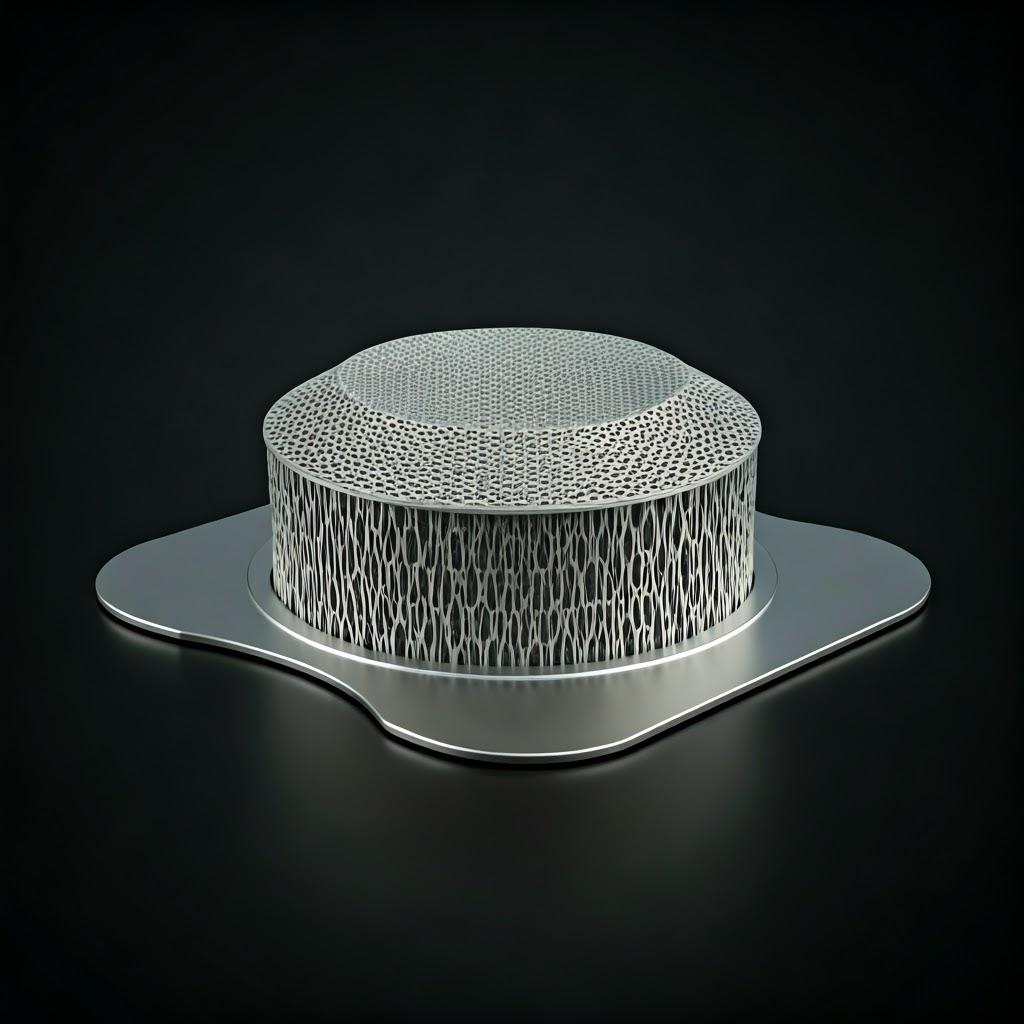
Frequently Asked Questions (FAQ) about 3D Printed Automotive Heat Shields
As metal additive manufacturing becomes more prevalent in the automotive industry, engineers and designers often have specific questions about its application for components like heat shields. Here are answers to some common queries:
1. How durable are 3D printed metal heat shields compared to traditional stamped ones?
- Odpověď: The durability of a 3D printed heat shield is excellent and often superior to traditional stamped parts, provided the correct material is chosen and the part is designed and processed correctly.
- Vlastnosti materiálu: Metal AM utilizes robust engineering materials like AlSi10Mg and high-performance alloys like IN625. When printed to high density (>99.5%) and properly heat-treated, these materials exhibit mechanical properties (strength, fatigue resistance, creep resistance) comparable or superior to their wrought or cast counterparts, especially at elevated temperatures (in the case of IN625). IN625 heat resistance is exceptional.
- Optimalizace designu: AM allows for designs optimized for durability. Features like integrated stiffening ribs, optimized wall thicknesses, and the elimination of welds or fasteners (potential failure points in stamped assemblies) can enhance structural integrity and fatigue life.
- Potential Issues: Like any manufacturing process, potential defects (e.g., porosity, residual stress if not properly managed) could impact durability. This underscores the importance of choosing a quality provider with robust process control and post-processing expertise, like Met3dp.
- Srovnání: A well-designed and manufactured 3D printed IN625 heat shield operating in a high-temperature environment (e.g., near a turbocharger) will typically offer significantly better long-term durability and resistance to thermal fatigue than a comparable shield stamped from conventional steel or aluminum alloys. An AlSi10Mg shield, used within its temperature limits, will offer excellent durability comparable to traditional aluminum shields but with added design flexibility and potentially lower weight.
2. Is metal 3D printing cost-effective for high-volume heat shield production?
- Odpověď: Generally, for very high-volume production (tens or hundreds of thousands per year) of simple heat shield designs, traditional stamping remains more cost-effective due to lower per-part costs once tooling is amortized. However, the cost comparison heat shield AM vs traditional is nuanced:
- AM Strengths: Metal AM is highly cost-effective for:
- Prototyping & Development: Eliminates tooling costs, enabling rapid iteration at lower overall development expense.
- Low-to-Mid Volume Production: For volumes where tooling costs for stamping are prohibitive (e.g., niche vehicles, aftermarket parts, initial production ramps).
- High Complexity: When the heat shield design is very complex (conformal shapes, internal features, integrated elements), stamping may require multiple tools and assembly steps, potentially making AM cost-competitive even at moderate volumes.
- Přizpůsobení: For bespoke or highly customized shields (e.g., motorsports), AM is often the only viable option.
- On-Demand / Spare Parts: Printing parts as needed avoids warehousing costs and is ideal for legacy component replacement.
- Breakeven Point: The exact volume at which stamping becomes cheaper depends heavily on part complexity, material, and tooling cost. For complex IN625 shields, AM might remain competitive at surprisingly high volumes compared to multi-stage forming and fabrication of the same design.
- Total Cost of Ownership: Consider factors beyond per-part price: reduced assembly time (part consolidation), lighter weight (fuel savings), potentially longer service life (better materials/design).
- AM Strengths: Metal AM is highly cost-effective for:
3. What is the typical temperature resistance of 3D printed heat shields made from AlSi10Mg and IN625?
- Odpověď: Temperature resistance is a key differentiator:
- AlSi10Mg: This aluminum alloy has moderate temperature resistance. Its mechanical properties significantly degrade above approximately 200-250°C (392-482°F). It is suitable for heat shields located further from extreme heat sources, such as lower sections of the exhaust, underbody components away from the catalytic converter, or shielding electronics from moderate engine bay heat.
- IN625 (Inconel® 625): This nickel superalloy offers excellent high-temperature strength and oxidation resistance. It maintains useful strength up to 800-900°C (1472-1652°F) or even higher for short durations, depending on the load conditions. This makes it the ideal best material for exhaust heat shield applications near manifolds, catalytic converters, turbochargers, and high-performance exhaust systems where temperatures are extreme.
4. Can complex, integrated mounting features be printed directly onto the heat shield?
- Odpověď: Absolutely. This is one of the key advantages of metal 3D printing for heat shields. Features like mounting bosses, threaded holes (often printed slightly undersized then tapped), locating pins, brackets, and clips can be designed directly into the heat shield model and printed as a single, monolithic part.
- Výhody:
- Konsolidace částí: Reduces the number of individual components, simplifying assembly and inventory.
- Reduced Assembly Time/Cost: Eliminates welding, riveting, or fastening operations.
- Improved Strength/Reliability: Integrated features can be stronger than attached ones, eliminating potential failure points at joints.
- Flexibilita designu: Allows for optimized placement and geometry of mounting features without the constraints of traditional manufacturing.
- Úvahy: DfAM principles should be applied to ensure these features are printable (e.g., supported if necessary, appropriate minimum feature sizes) and functional (e.g., designing sufficient material around threaded holes). Post-processing like tapping or reaming may be required for precise interfaces.
- Výhody:
Conclusion: The Future of Automotive Thermal Protection is Additive
The journey through the intricacies of custom 3D printed heat shields for vehicles reveals a compelling narrative: metal additive manufacturing is not merely an alternative; it’s a transformative force reshaping how we approach automotive thermal management. By breaking free from the geometric constraints of traditional stamping and forming, metal AM empowers engineers to design and produce advanced heat shield solutions that are lighter, more complex, highly customized, and often exhibit superior performance, particularly in demanding high-temperature environments.
We’ve explored the critical functions of heat shields across various automotive applications, from mitigating exhaust heat to protecting sensitive EV batteries. The distinct advantages of using metal AM – unparalleled design freedom enabling conformal shapes and integrated features, significant lightweighting potential through topology optimization and lattice structures, rapid prototyping accelerating development cycles, and the capability for on-demand production and customization – present a clear value proposition for OEM automotive parts suppliers, aftermarket specialists, and motorsports teams alike.
The strategic selection of materials like the lightweight AlSi 10Mg for moderate temperature zones and the robust, heat-resistant IN625 for extreme environments, coupled with high-quality powders like those produced by Met3dp, forms the foundation for reliable performance. Understanding and applying DfAM principles, recognizing the achievable tolerances and surface finishes, and implementing necessary post-processing steps are all crucial elements in harnessing the full potential of this technology. While challenges exist, partnering with an experienced and vertically integrated poskytovatel služeb 3D tisku kovů like Met3dp, who possesses deep expertise from powder to finished part, effectively mitigates risks and ensures successful outcomes.
The ability to create optimized, application-specific thermal management components quickly and efficiently aligns perfectly with the trajectory of the automotive industry towards more complex powertrains, electrification, higher performance demands, and increasing personalization. Metal 3D printing is a key enabler of digital manufacturing automotive strategies, offering agility and innovation previously unattainable.
As we look to the future, the role of additive manufacturing in producing critical components like heat shields will only continue to grow. It offers a pathway to enhanced vehicle efficiency, improved safety, faster innovation, and more sustainable manufacturing practices through material optimization and reduced waste.
Ready to explore how metal 3D printing can revolutionize your automotive thermal management challenges?
Met3dp Technology Co., LTD, with its industry-leading SEBM printers, advanced gas atomization and PREP powder manufacturing systems, and extensive portfolio of high-performance metal powders including AlSi10Mg and IN625, is your ideal Met3dp additive manufacturing partner. Our team possesses decades of collective expertise to support you from design consultation to final part production.
Contact Met3dp today to discuss your specific heat shield requirements or other custom automotive components supplier needs. Let us help you leverage the power of additive manufacturing to accelerate your innovation and drive the future of vehicle performance and efficiency. Visit our website or reach out to our expert team to begin the conversation.
Sdílet na
Facebook
Cvrlikání
LinkedIn
WhatsApp
E-mailem
MET3DP Technology Co., LTD je předním poskytovatelem řešení aditivní výroby se sídlem v Qingdao v Číně. Naše společnost se specializuje na zařízení pro 3D tisk a vysoce výkonné kovové prášky pro průmyslové aplikace.
Dotaz k získání nejlepší ceny a přizpůsobeného řešení pro vaše podnikání!
Související články
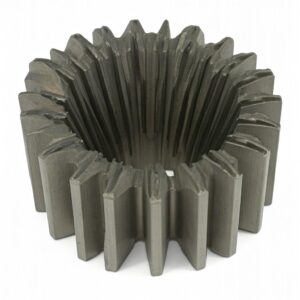
Vysoce výkonné segmenty lopatek trysek: Revoluce v účinnosti turbín díky 3D tisku z kovu
Přečtěte si více "
13. května 2025
Žádné komentáře
O Met3DP
Nedávná aktualizace
Náš produkt
KONTAKTUJTE NÁS
Nějaké otázky? Pošlete nám zprávu hned teď! Po obdržení vaší zprávy obsloužíme vaši žádost s celým týmem.
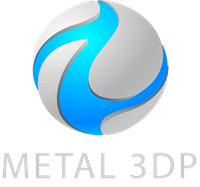
Kovové prášky pro 3D tisk a aditivní výrobu
SPOLEČNOST
PRODUKT
kontaktní informace
- Město Qingdao, Shandong, Čína
- [email protected]
- [email protected]
- +86 19116340731