Lightweight Brake Pedal Arms via Metal Additive Manufacturing
Obsah
Introduction: Revolutionizing Automotive Components with Metal AM
In the intricate system that constitutes a modern vehicle, few components are as fundamentally critical to safety and driver control as the brake pedal arms. It’s the direct interface between the driver’s input and the vehicle’s braking system, translating foot pressure into hydraulic force that brings tonnes of metal safely to a halt. For decades, these components have been reliably manufactured using traditional methods like casting or forging, often resulting in robust but relatively heavy parts. However, the automotive industry is undergoing a profound transformation, driven by the dual imperatives of electrification and relentless performance enhancement. In this new era, every gram matters. Lightweighting is no longer a niche pursuit for high-end racing teams; it’s a core engineering principle essential for extending electric vehicle (EV) range, improving handling dynamics, reducing emissions, and boosting overall efficiency.
This industry-wide push towards mass reduction has opened the door for disruptive technologies capable of reimagining component design and manufacturing. Enter výroba aditiv kovů (AM), commonly known as metal 3D printing. This technology is rapidly moving beyond prototyping and into the realm of functional end-use parts, offering unprecedented design freedom and the ability to create highly optimized, lightweight structures that were previously impossible to produce. For components like brake pedal arms, metal AM presents a transformative opportunity. It allows engineers to break free from the constraints of traditional manufacturing, enabling the creation of organically shaped, topologically optimized pedals that maintain or even exceed the strength and stiffness requirements while shedding significant weight. This shift has profound implications not just for vehicle performance but also for the entire automotive dodavatelský řetězec, která nabízí automotive OEMs a Tier 1 suppliers new levels of agility and customization potential. As we delve deeper, we’ll explore how leveraging advanced metal powders and printing processes can unlock the next generation of automotive brake system components.
Applications & Use Cases: Where Lightweight Brake Pedals Shine
While the concept of a 3D printed metal brake pedal might sound futuristic, its applications are grounded in tangible engineering benefits that address specific needs within the automotive landscape. The advantages of lightweighting and design optimization offered by metal AM make these components particularly valuable in several key areas:
- High-Performance Sports Cars & Supercars: In vehicles where performance is paramount, minimizing unsprung and overall mass is crucial for superior handling, acceleration, and braking. A lightweight brake pedal contributes to a more responsive feel and allows engineers to fine-tune the vehicle’s dynamics with greater precision. Manufacturers catering to this exclusive market often require highly engineered, custom solutions where the benefits of AM outweigh potentially higher per-part costs compared to mass-market vehicles.
- Electric Vehicles (EVs): Range anxiety remains a significant factor in EV adoption. Reducing the overall vehicle weight is one of the most effective ways to maximize battery range. While a single brake pedal’s weight saving might seem small in isolation, it contributes to the cumulative mass reduction efforts across hundreds of components. Furthermore, the design freedom allows for potential integration of features or sensors specific to regenerative braking systems. Automotive procurement managers working on EV platforms are constantly seeking innovative ways to shave off kilograms.
- Motorsport & Racing: In the ultra-competitive world of racing, teams operate under stringent regulations but constantly seek marginal gains. Metal AM allows for the rapid development and production of bespoke, highly optimized brake pedals tailored to specific cars or drivers. The ability to quickly iterate designs based on track data or driver feedback is invaluable, and the superior strength-to-weight ratio is a critical performance differentiator.
- Advanced Prototyping & Niche Vehicles: Before committing to expensive tooling for casting or forging, automotive manufacturers can use metal AM to produce functional prototypes of new brake pedal designs. This allows for real-world testing and validation much earlier in the development cycle, reducing risks and accelerating time-to-market. It’s also ideal for low-volume niche vehicles, bespoke builds, or classic car restorations where creating traditional tooling would be prohibitively expensive.
- Optimizing Pedal Feel and Ergonomics: Beyond pure lightweighting, the design freedom allows engineers to experiment with structures that enhance pedal stiffness and provide a more direct, confidence-inspiring feel for the driver, contributing to the perceived quality and sportiness of the vehicle.
Essentially, wherever vehicle mass is a critical design parameter, development speed is essential, or unique design requirements preclude traditional manufacturing, lightweight metal AM brake pedals offer a compelling solution for forward-thinking automotive engineers and component suppliers.
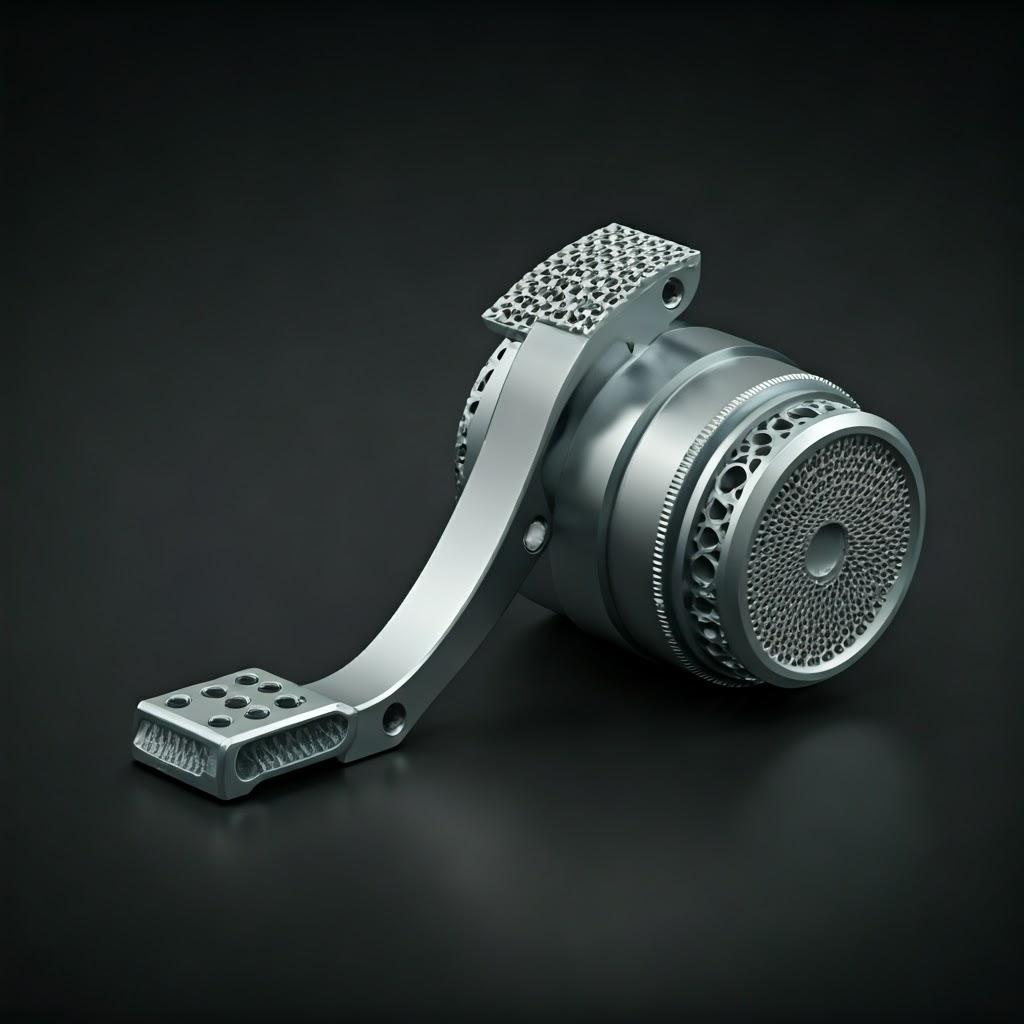
Why Metal 3D Printing for Brake Pedal Arms? Key Advantages
Opting for metal additive manufacturing over established methods like casting, forging, or machining for a safety-critical component like a brake pedal arm requires compelling justification. The advantages offered by AM, particularly Laser Powder Bed Fusion (L-PBF), are significant and address many modern automotive challenges:
- Unmatched Design Freedom & Topology Optimization: This is arguably the most transformative benefit. Traditional methods are inherently limited by tooling requirements (draft angles for casting, die shapes for forging, tool access for machining). AM builds parts layer by layer, freeing designers to:
- Implement Topology Optimization: Use software to analyze load paths and remove material from non-critical areas, resulting in organic, skeletal structures that are optimally strong only where needed. This can lead to weight reductions of 30-60% or more compared to traditional designs, while maintaining or even increasing stiffness.
- Create Complex Internal Geometries: Incorporate internal lattice structures for further weight reduction and stiffness tuning, or design internal channels if needed for sensors or other functionalities – features impossible to achieve with conventional techniques.
- Realize Biomimetic Designs: Draw inspiration from natural structures (like bone) that have evolved for maximum efficiency.
- Significant Weight Reduction: As highlighted, the ability to optimize geometry directly translates into substantial mass savings. This impacts:
- Vehicle Performance: Lower inertia, improved acceleration/braking response.
- Fuel/Energy Efficiency: Reduced overall vehicle mass contributes directly to lower fuel consumption or extended EV range.
- Material Savings: Using only the necessary material reduces waste and raw material costs over the long term, especially with valuable alloys.
- Konsolidace částí: Complex assemblies often required with traditional manufacturing (e.g., a pedal arm welded or bolted to a mounting bracket) can potentially be redesigned and printed as a single, monolithic part. This offers several benefits:
- Reduced Assembly Time & Cost: Fewer components mean simpler, faster assembly lines.
- Improved Structural Integrity: Eliminates potential failure points at joints or welds.
- Simplified Supply Chain: Fewer individual part numbers to manage, track, and source for automotive wholesale buyers and logistics teams.
- Rapid Prototyping, Iteration & Development Speed: The journey from CAD model to functional metal prototype can be drastically shortened with AM.
- Tooling Elimination: AM requires no expensive, time-consuming tooling (moulds, dies). Design changes can be implemented digitally and reprinted quickly.
- Faster Validation: Functional prototypes can be produced in days or weeks, allowing for quicker testing cycles (fit, form, function, even limited rig testing). This accelerates the overall vehicle development timeline.
- Supply Chain Flexibility & On-Demand Production:
- Snížené zásoby: Parts can be printed as needed, minimizing warehousing costs and obsolescence risks, a key benefit for managing dodavatel relationships and inventory levels.
- Distributed Manufacturing: Enables production closer to the point of assembly, potentially reducing shipping costs and lead times.
- Přizpůsobení: Facilitates easier production of variants or custom designs without major retooling investments.
While traditional methods remain suitable for high-volume, cost-sensitive standard components, metal AM provides a powerful alternative for applications demanding lightweighting, complex geometries, speed, and customization, reshaping how engineers and specialisté na veřejné zakázky approach component design and sourcing.
Material Focus: AlSi10Mg and A7075 for Automotive Performance
The success of any metal AM component hinges critically on selecting the right material. For lightweight, high-performance automotive applications like brake pedal arms, aluminum alloys are often the primary candidates due to their inherent low density and good mechanical properties. Two alloys stand out for L-PBF processing in this context: AlSi 10Mg a A7075. Choosing between them depends heavily on the specific performance requirements, operating environment, and cost targets of the application.
AlSi10Mg: This is one of the most common and well-understood aluminum alloys used in additive manufacturing. It’s essentially a casting alloy adapted for L-PBF processes.
- Klíčové vlastnosti:
- Dobrý poměr pevnosti a hmotnosti: Offers respectable mechanical properties suitable for many structural applications.
- Vynikající tisknutelnost: Relatively easy to process via L-PBF, with well-established parameters leading to dense parts.
- Dobré tepelné vlastnosti: Efficiently dissipates heat.
- Dobrá odolnost proti korozi: Performs well in typical automotive environments.
- Svařitelnost: Can be welded if necessary, although part consolidation often minimizes this need.
- Suitability for Brake Pedals: AlSi10Mg provides a robust and cost-effective solution for many lightweight brake pedal designs, especially where extreme load cases are not the primary driver or where it replaces a standard cast aluminum part. It allows significant weight savings through topology optimization compared to traditional designs.
- Met3dp Powder Quality: Achieving the desired mechanical properties consistently requires high-quality input material. Companies like Met3dp se specializují na výrobu kovové prášky using advanced gas atomization techniques. This ensures their AlSi10Mg powder exhibits high sphericity, good flowability, and controlled particle size distribution – all crucial factors for achieving dense, reliable parts with predictable properties in demanding 3D tisk z kovu procesy.
A7075 (Aluminum Alloy 7075): This is a high-strength, zinc-alloyed aluminum widely used in aerospace and high-performance applications. Traditionally available as wrought products (sheets, plates, extrusions), adapting it for AM has presented challenges but offers significant performance benefits.
- Klíčové vlastnosti:
- Very High Strength: One of the highest-strength aluminum alloys available, approaching the strength of some steels but at roughly one-third the density.
- Excellent Fatigue Strength: Crucial for components subjected to cyclic loading, like a brake pedal.
- Good Fracture Toughness (when properly heat treated).
- Challenges & Solutions in AM: A7075 can be prone to hot cracking during the rapid melting and solidification cycles of L-PBF. Successful printing requires careful parameter optimization (laser power, scan speed, hatching patterns), potentially modified alloy compositions specifically for AM, and meticulous post-process heat treatment (typically a T6 temper) to achieve optimal properties. Partnering with an AM provider experienced with high-strength aluminum alloys is essential.
- Suitability for Brake Pedals: A7075 is the preferred choice when maximum strength and fatigue resistance are critical, such as in high-performance racing applications or vehicles subjected to extreme durability requirements. The higher material and processing costs are justified by the superior performance and potential for even greater weight savings due to the material’s inherent strength.
Material Selection Comparison:
Vlastnosti | AlSi 10Mg | A7075 (AM Processed) | Importance for Brake Pedal Arm |
---|---|---|---|
Primary Alloying | Silicon (Si), Magnesium (Mg) | Zinek (Zn), Hořčík (Mg), Měď (Cu) | Influences core mechanical properties. |
Typical Yield Strength (Heat Treated) | ~230-280 MPa | ~450-520 MPa | Resistance to permanent deformation under load. Critical. |
Typical Tensile Strength (Heat Treated) | ~330-430 MPa | ~500-580 MPa | Maximum stress before fracture. Critical for safety margin. |
Hustota | ~2.67 g/cm³ | ~2.81 g/cm³ | Key for lightweighting. Both are excellent. |
Printability (L-PBF) | Generally Good | More Challenging (requires expertise) | Affects process reliability, defect potential, and cost. |
Únavová pevnost | Mírný | Velmi dobře | Resistance to failure under cyclic loading. Highly important. |
Odolnost proti korozi | Dobrý | Moderate (can require surface protection) | Important for durability in automotive environments. |
Relativní náklady | Dolní | Vyšší | Significant factor for procurement and overall project budget. |
Ideal Use Case | General lightweighting, EV, standard performance | High-performance, racing, maximum strength needs | Matching material to application requirements. |
Export do archů
Ultimately, the choice between AlSi10Mg and A7075 for a metal AM brake pedal involves a trade-off between performance requirements, manufacturing complexity, and budget. Both materials, when processed correctly using high-quality powders and optimized L-PBF parameters, enable the creation of lightweight, high-performance components that significantly advance automotive design possibilities. Consulting with material experts and experienced AM service providers is crucial for making the optimal selection.
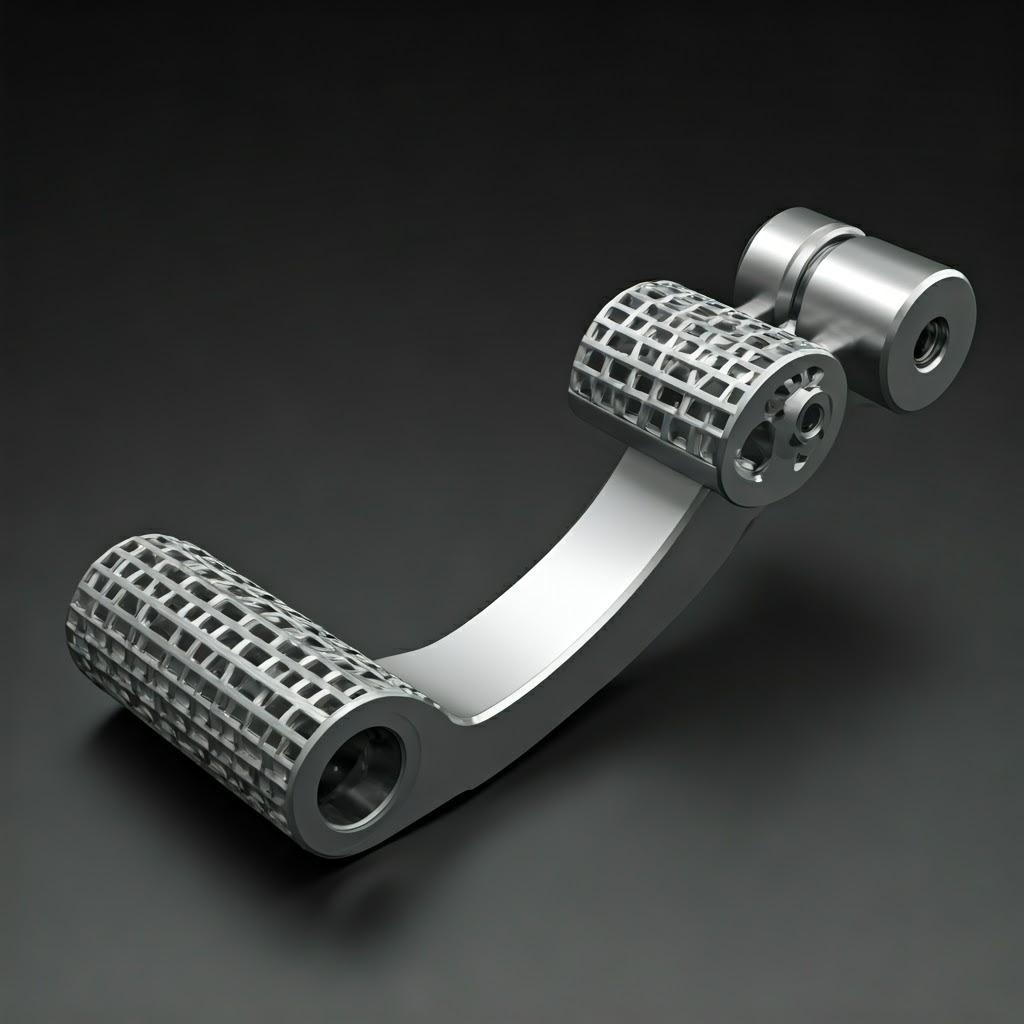
Design for Additive Manufacturing (DfAM): Optimizing Brake Pedal Geometry
Simply taking an existing brake pedal design intended for casting or forging and sending it to a metal 3D printer will rarely unlock the full potential of additive manufacturing. To truly leverage the benefits described earlier – particularly significant lightweighting and performance enhancement – engineers must embrace Design pro aditivní výrobu (DfAM). DfAM is a paradigm shift that involves designing components specifically with the possibilities and constraints of the layer-by-layer AM process in mind. For a critical component like a brake pedal arm, applying DfAM principles is essential for success.
Key DfAM considerations for metal AM brake pedals include:
- Topology Optimization & Load Path Analysis: This is often the starting point for lightweighting. Finite Element Analysis (FEA) software can identify the primary load paths through the pedal during braking scenarios. Topology optimization algorithms then systematically remove material from areas experiencing low stress, leaving behind an organic, efficient structure that directs forces optimally. The resulting designs often look radically different from their traditional counterparts, featuring smooth curves and skeletal forms.
- Lattice Structures & Infill Strategies: For areas requiring stiffness or specific energy absorption characteristics, internal lattice structures can be incorporated. These complex, repeating geometric patterns (like honeycomb, cubic, or gyroid structures) offer:
- High stiffness-to-weight ratios.
- Enhanced energy absorption potential (relevant for crashworthiness considerations).
- Improved thermal dissipation if needed.
- Design flexibility to tune local stiffness. However, designing effective lattices also requires considering powder removal after printing.
- Minimum Feature Sizes & Wall Thickness: AM processes have limitations on the minimum size of features (holes, pins) and the thinnest walls they can reliably produce. For L-PBF with aluminum alloys, minimum wall thickness is typically around 0.4-0.8 mm, depending on the machine and geometry. Designs must respect these limits to ensure printability and structural integrity. Overly thin sections can warp or fail during printing or use.
- Build Orientation Strategy: How the part is oriented on the build platform significantly impacts:
- Podpůrné struktury: Affects the quantity, location, and removability of supports. Steeper overhangs (typically >45 degrees from horizontal) require support.
- Povrchová úprava: Upward-facing and vertical surfaces generally have better finish than downward-facing supported surfaces.
- Mechanické vlastnosti: Anisotropy (directional variation in properties) can occur in AM parts, although it’s generally less pronounced in metals compared to polymers. Orientation might be chosen to align the strongest direction with the primary load axis.
- Build Time & Cost: Taller builds generally take longer. Fitting more parts onto a single build plate reduces per-part cost, influencing decisions for volume production.
- Support Structure Design & Minimization: Supports are often necessary evils in metal AM. They anchor the part to the build plate, counteract warping, and support overhanging features. However, they consume extra material, add print time, require removal (a significant post-processing step), and can leave witness marks on the surface. Good DfAM practice involves:
- Minimizing overhangs and designing self-supporting angles where possible (typically up to 45 degrees).
- Designing supports that are strong enough but easy to access and remove (e.g., using conical or perforated structures).
- Considering where support witness marks are acceptable and orienting the part accordingly.
- Designing for Post-Processing & Inspection: Critical features requiring tight tolerances or specific surface finishes (like pivot bores or sensor mounting points) should be designed with extra material (“machining stock”) to allow for precise finishing via CNC machining after printing. Access for inspection tools (like CMM probes or NDT scanners) should also be considered.
Leveraging DfAM effectively requires a deep understanding of the chosen AM process (like L-PBF) and the material behavior. Collaborating with experienced AM service providers who offer DfAM expertise can significantly shorten the learning curve and lead to more optimized and manufacturable designs, ensuring components meet rigorous manufacturing standards.
Achievable Tolerances, Surface Finish, and Dimensional Accuracy
Engineers and procurement specialists accustomed to the tight tolerances and smooth finishes of traditional machining need to understand the capabilities and nuances of metal additive manufacturing regarding dimensional accuracy and surface quality. While metal AM can produce highly complex parts, it typically requires post-processing to achieve the very high precision needed for certain features on a brake pedal arm.
Here’s a breakdown of what to expect:
- Typical Tolerances: For L-PBF processes with aluminum alloys like AlSi10Mg or A7075, as-printed dimensional accuracy often falls within the range of +/- 0.1 mm to +/- 0.3 mm or +/- 0.1% to 0.3% of the dimension, whichever is greater. General tolerances can often be related to standards like ISO 2768-m (medium) or -f (fine) for linear and angular dimensions, but this is highly dependent on the part geometry, size, orientation, and process control. Achieving tighter tolerances usually requires secondary machining operations.
- Faktory ovlivňující přesnost: Several factors contribute to the final dimensional accuracy of an AM part:
- Kalibrace stroje: Regular calibration of the laser scanner system and build platform is crucial.
- Parametry procesu: Laser power, scan speed, layer thickness, and hatch strategy all influence melt pool dynamics and solidification, affecting dimensional stability.
- Thermal Stresses: The rapid heating and cooling cycles inherent in L-PBF induce internal stresses that can cause warping or distortion, particularly in large or complex parts. Effective thermal management and stress relief post-processing are critical.
- Part Geometry & Size: Larger parts and complex geometries with varying cross-sections are more prone to deviation.
- Podpůrné struktury: How the part is supported affects its stability during the build and potential distortion upon removal.
- Následné zpracování: Stress relief heat treatments can cause minor dimensional changes, which need to be accounted for. Machining obviously improves accuracy but only on the machined features.
- As-Built Surface Finish: The layer-by-layer nature of AM results in a characteristic surface texture. The as-built surface roughness (Ra) for L-PBF aluminum parts typically ranges from 6 µm to 20 µm (around 240-800 µin).
- Vertical Walls: Generally offer the smoothest finish.
- Upward-Facing Surfaces: Slightly rougher due to layer stepping.
- Downward-Facing Surfaces: Tend to be the roughest, especially those requiring supports, as the interface between the support and the part can be irregular.
- Implications: This as-built finish is often acceptable for non-critical surfaces but is usually insufficient for bearing interfaces, sealing surfaces, or areas requiring smooth tactile feedback.
- Secondary Machining Requirements: For a brake pedal arm, certain features almost always require post-process CNC machining to meet functional requirements:
- Pivot Bore(s): Need precise diameter, roundness, and surface finish for smooth operation and interaction with bushings or bearings.
- Mounting Holes/Interfaces: Require accurate positioning and dimensions for assembly to the bulkhead or pedal box.
- Pedal Pad Interface: May require specific flatness or features for attaching the pedal pad securely.
- Sensor Mounting Points: If applicable, these often need precise location and geometry.
- Quality Control & Inspection: Ensuring dimensional integrity requires robust kontrola kvality measures. This typically involves 3D scanning or Coordinate Measuring Machine (CMM) inspection to compare the final part against the original CAD model and technical drawings. Non-destructive testing (NDT) methods may also be used to check for internal defects that could affect structural integrity, crucial for component distributors guaranteeing part quality.
Understanding these factors allows engineers to design parts appropriately (e.g., adding machining stock) and set realistic expectations for tolerances and finishes achievable directly from the AM process versus those requiring secondary operations.
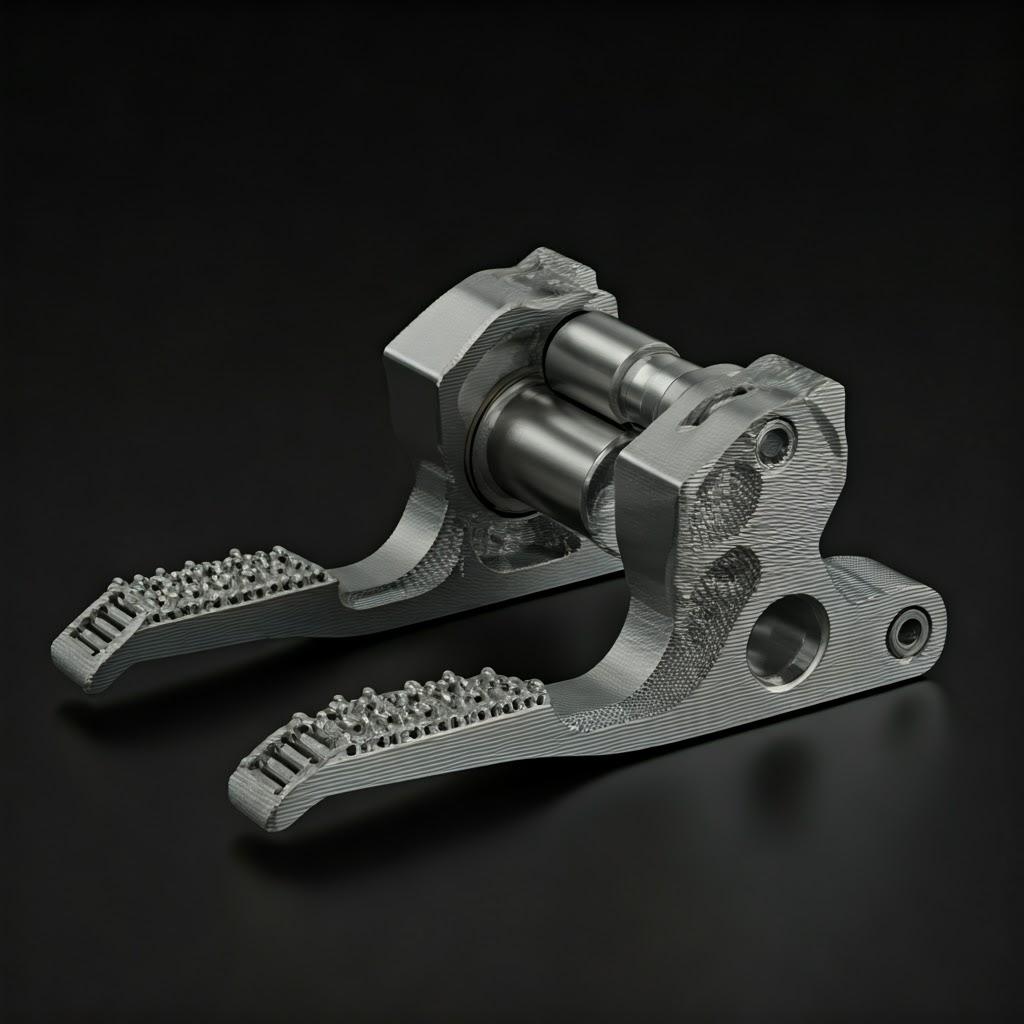
Essential Post-Processing Steps for Metal AM Brake Pedals
A metal 3D printed part, upon removal from the printer, is rarely ready for end-use, especially for a demanding automotive application like a brake pedal. A series of crucial post-processing steps are required to achieve the necessary material properties, dimensional accuracy, surface finish, and overall quality. Neglecting these steps can compromise the performance and safety of the component.
The typical post-processing workflow for an L-PBF aluminum alloy brake pedal arm includes:
- Úleva od stresu / tepelné ošetření: This is arguably the most critical step for aluminum AM parts.
- Účel: The rapid heating/cooling cycles during L-PBF create significant residual stresses within the part. These stresses can cause distortion after removal from the build plate or even lead to premature failure under load. Heat treatment relieves these stresses and homogenizes the material’s microstructure. For alloys like AlSi10Mg and especially A7075, specific heat treatment cycles (like the T6 temper involving solutionizing, quenching, and artificial aging) are essential to develop the required high strength and hardness.
- Proces: Parts are typically heat-treated while still attached to the build plate to minimize distortion. The exact temperature profile and duration depend heavily on the alloy and desired properties. Expertise in metallurgy and heat treatment protocols for AM materials is vital.
- Part Removal from Build Plate: Once heat treatment is complete (or sometimes before, depending on the workflow), the part needs to be separated from the build plate.
- Metody: This is commonly done using wire Electrical Discharge Machining (EDM) or a bandsaw. Care must be taken not to damage the part itself.
- Odstranění podpůrné konstrukce: The supports used during the printing process must now be removed.
- Metody: This can be a labor-intensive process, often involving manual breaking or cutting (using pliers, grinders, or hand tools), CNC machining, or sometimes wire EDM for difficult-to-reach areas.
- Úvahy: The ease of support removal is heavily influenced by the DfAM choices made earlier. Poorly designed supports can be difficult to remove cleanly, potentially damaging the part surface and increasing costs.
- Cleaning & Powder Removal: Any unfused powder trapped in internal channels, lattice structures, or complex geometries must be thoroughly removed.
- Metody: This typically involves compressed air blasting, bead blasting, or ultrasonic cleaning. Access for cleaning tools needs to be considered during the design phase. Incomplete powder removal can add weight and potentially compromise performance or interfere with assembly.
- Povrchová úprava: Depending on the requirements, various surface treatments can be applied:
- Tryskání kuličkami / kuličkování: Creates a uniform matte finish, removes loose particles, and can sometimes improve fatigue life through compressive residual stresses (shot peening).
- Obrábění / vibrační úprava: Smooths surfaces and edges by tumbling parts with media. Effective for batch processing but less controlled for specific features.
- Leštění: For achieving very smooth, reflective surfaces, often done manually or with automated polishing tools for specific areas.
- Anodizing: An electrochemical process for aluminum that creates a hard, corrosion-resistant oxide layer. Can also be used for coloring. Essential for A7075 in many applications due to its moderate corrosion resistance.
- Painting / Coating: Applying specialized automotive paints or coatings for aesthetics and enhanced environmental protection.
- CNC obrábění: As discussed previously, critical features requiring tight tolerances (+/- 0.01 to 0.05 mm) and specific surface finishes (Ra < 1.6 µm or lower) are typically machined. This ensures precise fit and function for pivots, mounting points, and interfaces.
- Inspection and Validation: The final step involves rigorous quality checks:
- Rozměrová kontrola: Using CMM or 3D scanning.
- Nedestruktivní zkoušení (NDT): Methods like CT scanning (Computed Tomography) can inspect internal structures for defects (porosity, cracks) without damaging the part. Dye penetrant or ultrasonic testing might also be used.
- Material Property Verification: Testing sample coupons printed alongside the main part to confirm tensile strength, hardness, etc.
Successfully navigating these post-processing steps requires specialized equipment, skilled technicians, and well-defined procedures. Partnering with an AM service provider like Met3dp, which understands the full workflow from powder to finished part, including various tiskových metod and necessary secondary operations, is crucial for achieving reliable and high-quality results.
Common Challenges in Printing Brake Pedals & Mitigation Strategies
While metal AM offers tremendous potential for automotive components like brake pedals, it’s not without its challenges. Awareness of these potential issues and implementing effective mitigation strategies are key to successful adoption, particularly when scaling towards volume production.
Here are some common challenges encountered when printing aluminum alloy brake pedals using L-PBF:
- Warping and Distortion:
- Cause: Significant thermal gradients between the molten material and surrounding powder/solidified layers induce stress, causing parts (especially large, flat, or asymmetric ones) to warp or pull away from the build plate.
- Mitigation:
- Optimalizovaná orientace: Orienting the part to minimize large flat surfaces parallel to the build plate and reduce thermal mass concentration.
- Effective Support Strategy: Robust supports anchor the part securely and help dissipate heat.
- Build Plate Heating: Pre-heating the build plate reduces the thermal gradient.
- Process Parameter Control: Fine-tuning laser parameters to manage heat input.
- Stress Relief Heat Treatment: Essential for relieving built-up stresses post-print.
- Residual Stress Management:
- Cause: Even if warping is controlled during the build, significant residual stress remains locked within the part. This can lead to distortion upon removal from the build plate or support structures, or even premature failure in service.
- Mitigation:
- Mandatory Heat Treatment: Implementing appropriate stress relief and/or solution treatment and aging cycles tailored to the specific alloy (AlSi10Mg vs. A7075) is non-negotiable.
- Simulation: Using process simulation software to predict stress accumulation and optimize build strategy.
- Support Removal Complexity & Surface Quality:
- Cause: Supports needed for overhangs or stability can be difficult to remove cleanly, especially from complex internal geometries or delicate features. Removal processes can leave witness marks or scratches on the part surface.
- Mitigation:
- DfAM for Support Minimization: Designing parts with self-supporting angles where possible.
- Smart Support Design: Using support structures (e.g., thin tips, perforations) designed for easier detachment.
- Strategic Orientation: Placing supports on non-critical surfaces where possible.
- Vhodné techniky odstraňování: Using careful manual methods or precise machining/EDM. Planning for finishing steps (blasting, polishing) to remove marks.
- Powder Removal from Internal Features:
- Cause: Complex internal channels or lattice structures designed for lightweighting can trap unfused metal powder.
- Mitigation:
- DfAM for Powder Escape: Designing internal features with drain holes or access points for powder removal.
- Thorough Cleaning Procedures: Utilizing effective methods like high-pressure air/gas jets, vibration tables, and potentially solvent cleaning.
- Inspection: Using methods like CT scanning to verify complete powder removal if critical.
- Kontrola pórovitosti:
- Cause: Small voids or pores can form within the printed material due to trapped gas, keyholing (vapor depression instability), or lack of fusion between layers. Porosity can significantly degrade mechanical properties, particularly fatigue life.
- Mitigation:
- Vysoce kvalitní prášek: Using powder with low internal gas content, controlled particle size distribution, and high sphericity (like those produced by Met3dp using advanced atomization).
- Optimized Print Parameters: Precisely controlling laser power, scan speed, layer thickness, and hatching strategy to ensure complete melting and fusion.
- Inert Atmosphere Control: Maintaining a high-purity inert gas environment (Argon or Nitrogen) in the build chamber to prevent oxidation.
- Monitorování procesů: Utilizing real-time monitoring systems (melt pool monitoring) to detect potential inconsistencies.
- Izostatické lisování za tepla (HIP): A post-processing step that uses high temperature and pressure to close internal pores (adds cost but can significantly improve properties for critical parts).
Overcoming these challenges consistently requires robust process control, high-quality materials, advanced equipment, and significant technical expertise. Working with experienced partners like Met3dp, known for their reliable printing systems and deep understanding of materials science and process optimization, provides assurance that these potential issues are proactively managed, leading to consistent, high-quality brake pedal arms ready for demanding automotive applications.
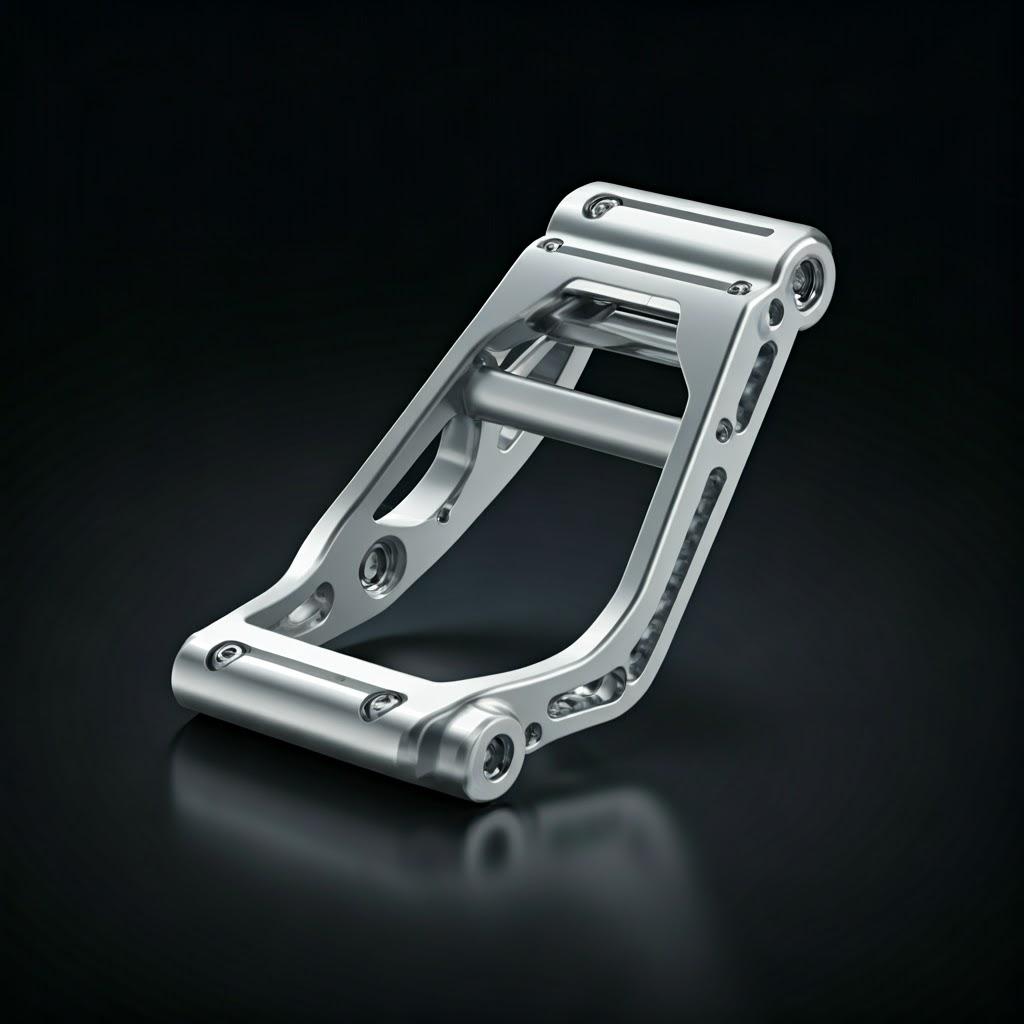
Selecting the Right Metal 3D Printing Service Provider for Automotive Parts
Choosing a partner for producing safety-critical components like brake pedal arms using additive manufacturing is a decision that requires careful consideration. Not all AM service providers possess the necessary expertise, equipment, or quality systems to meet the demanding requirements of the automotive industry. Engineers and procurement managers need a robust supplier evaluation process focused on several key criteria:
- Proven Automotive & Materials Expertise: Does the provider have demonstrable experience with automotive applications and specifically with the chosen materials (AlSi10Mg, A7075)? They should understand the performance requirements, testing protocols (like fatigue testing), and traceability needs common in the sector. Look for case studies or references related to structural automotive components.
- Relevant Quality Certifications: At a minimum, look for ISO 9001 certification, indicating a robust quality management system. For suppliers aiming for series production in automotive, certifications like IATF 16949 (or demonstrated progress towards it) are highly desirable, signifying adherence to stringent automotive quality standards.
- Material Quality Control: How does the provider ensure the quality and consistency of the metal powders used? Do they have controls for powder sourcing, handling, storage, and recycling (if applicable)? Consistent powder quality is fundamental to achieving repeatable mechanical properties. Providers who also manufacture their own powders, like Met3dp, often have an advantage here due to direct control over powder characteristics.
- Technology & Capacity: Does the provider have the right equipment (e.g., industrial-grade L-PBF machines suitable for aluminum alloys) and sufficient capacity? Consider their machine park size, build envelope capabilities, and ability to scale production if needed for low-to-mid volume production runs. Does their technology portfolio include complementary processes if needed? Met3dp, for instance, offers industry-leading capabilities in both L-PBF and SEBM (Selective Electron Beam Melting) printers, showcasing a breadth of technological expertise.
- Engineering & DfAM Support: Can the provider offer valuable input on Design for Additive Manufacturing (DfAM)? Collaborative design optimization can significantly improve part performance, reduce costs, and streamline production. Look for partners with skilled application engineers who can guide your team.
- Post-Processing Capabilities: Does the provider have in-house or tightly managed external capabilities for all necessary post-processing steps (heat treatment specific to AM aluminum alloys, precision machining, surface finishing, NDT inspection)? A vertically integrated approach often simplifies logistics and ensures accountability.
- Location, Logistics & Communication: Consider the provider’s location relative to your needs, typical shipping dodací lhůty, and their communication responsiveness. Clear communication channels are vital for managing complex projects.
Evaluating potential partners against these criteria helps ensure you select a supplier capable of delivering high-quality, reliable metal AM brake pedal arms that meet your specifications. Companies like Met3dp, headquartered in Qingdao, China, position themselves as a leading provider of comprehensive additive manufacturing solutions. With decades of collective expertise, advanced powder manufacturing systems (utilizing gas atomization and PREP technologies), industry-leading print accuracy, and a focus on mission-critical parts for aerospace, medical, and automotive sectors, they represent the type of capable partner required for such demanding applications. You can learn more O společnosti Met3dp and their commitment to quality and innovation.
Understanding Cost Drivers and Lead Times for AM Brake Pedals
One of the primary considerations for any engineering or procurement team evaluating a new manufacturing process is cost and lead time. Metal additive manufacturing has a different cost structure compared to traditional methods like casting or forging.
Key Cost Drivers for Metal AM Brake Pedals:
- Material Type & Consumption: The cost of the metal powder itself is a significant factor. High-strength alloys like A7075 are typically more expensive than standard AlSi10Mg. The total volume of material used (including support structures) directly impacts cost. Efficient DfAM and topology optimization help minimize material consumption.
- Machine Time (Build Time): L-PBF machines are expensive assets, and their operating time is a major cost component. Build time depends on:
- Part Height: Taller parts take longer.
- Part Volume & Complexity: More material to melt takes more time. Complex geometries might require slower scan speeds for accuracy.
- Number of Parts per Build: Maximizing the parts printed simultaneously on one build plate reduces the machine time allocated per part.
- Support Structure Volume & Removal: Material used for supports adds cost, and the labor/time required for their removal is a significant post-processing expense. Optimized design minimizes support needs.
- Post-Processing Intensity: Each step adds cost:
- Heat Treatment: Furnace time and energy consumption.
- Machining: Setup and machining time for critical features.
- Surface Finishing: Labor and consumables for blasting, polishing, anodizing, etc.
- Inspection: NDT and CMM inspection require specialized equipment and skilled operators.
- Objednávkové množství: While AM avoids tooling costs, the per-part cost is less sensitive to quantity than mass production methods. However, množstevní slevy are often available for larger B2B orders due to efficiencies in setup, batch processing, and optimized build plate utilization. Discuss potential wholesale inquiries directly with the provider.
- Engineering & Setup: Initial design consultation, DfAM optimization, and print file preparation may involve upfront engineering charges, especially for complex projects.
Typical Lead Times:
Lead times for AM parts are significantly faster than traditional methods requiring tooling, especially for prototypes and low volumes. However, multiple steps are involved:
- Citace & Design Review: 1-5 pracovních dnů (v závislosti na složitosti a rychlosti reakce poskytovatele).
- Print Preparation (File Setup): 0.5-2 business days.
- Tisk: 1-4 days (highly dependent on part size, complexity, and number of parts per build).
- Chlazení: Several hours to a full day within the machine or build removal station.
- Následné zpracování: 2-10 business days (this is often the longest stage, involving heat treatment cycles, support removal, machining setups, finishing, and inspection queues).
- Doprava: Dependent on location and shipping method.
Overall, expect lead times ranging from 1 to 4 weeks for a typical metal AM brake pedal project, depending heavily on the factors above. This is considerably faster than the months often required for traditional tooling development and initial part production. Accurate cost analysis and lead time estimates require submitting a specific design (CAD model and technical drawing) to potential suppliers for a detailed quote.
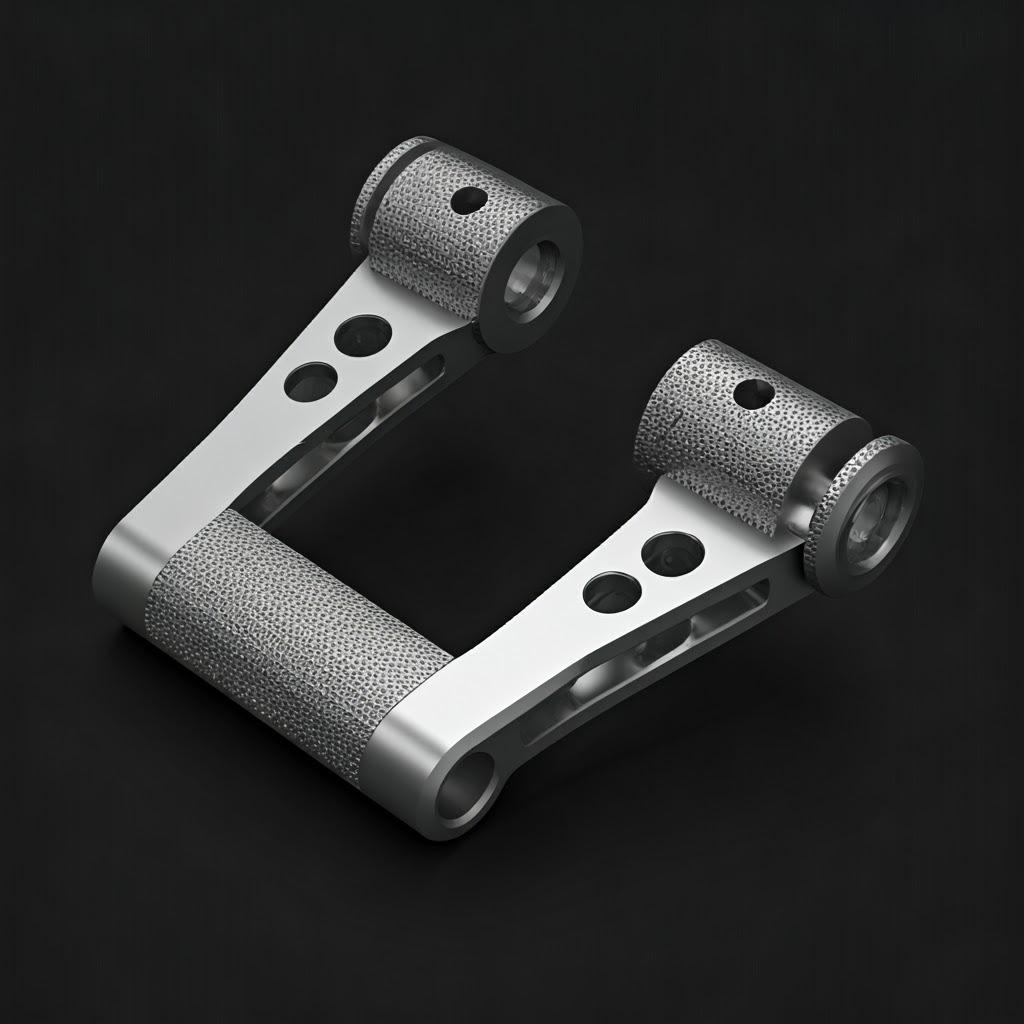
Často kladené otázky (FAQ)
- Q1: Are 3D printed metal brake pedals strong and safe enough for automotive use?
- A: Absolutely, provided they are designed, manufactured, and validated correctly. Using high-strength alloys like AlSi10Mg or A7075, combined with topology optimization and proper DfAM principles, results in parts that can meet or exceed the strength and stiffness requirements of traditional components. Crucially, rigorous process control during printing, appropriate heat treatment, and thorough NDT inspection (like CT scanning) are essential to ensure material integrity and absence of critical defects. Extensive simulation (FEA) and physical testing (static load tests, fatigue tests) are standard validation steps before implementation in any production vehicle.
- Q2: How does the cost of an AM brake pedal compare to a traditionally manufactured one?
- A: It depends heavily on the production volume and complexity. For prototypes and low-volume production (dozens to hundreds of parts), AM is often more cost-effective because it eliminates the high upfront cost of tooling (casting moulds, forging dies). For mass production (thousands or millions of parts), traditional methods typically achieve a lower per-part cost due to economies of scale. However, the AM part might enable system-level savings through lightweighting (improved fuel/energy efficiency) or part consolidation (reduced assembly costs) that offset a higher per-part price. A total cost of ownership analysis is often required.
- Q3: What kind of fatigue life can be expected from an aluminum AM brake pedal arm?
- A: Fatigue life is excellent, particularly for parts printed with high-quality powder, optimized parameters, and appropriate post-processing. High-strength alloys like A7075 offer inherently good fatigue resistance. Process control to minimize porosity is critical, as internal defects can act as fatigue initiation sites. Post-processing steps like Hot Isostatic Pressing (HIP), while adding cost, can further enhance fatigue life by closing internal pores. Surface finish and residual stresses also play a role. With proper engineering and manufacturing, AM brake pedals can be designed to meet or exceed the demanding fatigue life requirements of automotive applications.
- Q4: Can you achieve the same surface finish as a cast or forged part?
- A: The as-printed surface finish of an L-PBF part is typically rougher (Ra 6-20 µm) than a machined or even a smooth cast/forged surface. However, through post-processing techniques like bead blasting, tumbling, polishing, or machining, specific surfaces can achieve finishes that are comparable or even superior to traditional methods. Critical areas like pivot bores will typically be machined to achieve the required smooth finish (e.g., Ra < 1.6 µm or lower). Non-critical surfaces might retain the as-blasted finish or be painted/anodized. The required finish for each surface should be specified in the technical drawing for the product.
Conclusion: Accelerating Automotive Innovation with Optimized AM Brake Pedals
The automotive industry’s relentless pursuit of lightweighting, performance, and efficiency demands innovative manufacturing solutions. Metal additive manufacturing, specifically L-PBF using advanced aluminum alloys like AlSi10Mg and A7075, offers a powerful pathway to producing next-generation brake pedal arms. By unshackling designers from traditional constraints, AM enables the creation of topologically optimized, complex, and significantly lighter components without compromising strength or safety.
We’ve explored the compelling applications, the critical importance of Design for Additive Manufacturing (DfAM), the nuances of material selection, and the essential post-processing steps required to achieve production-ready parts. Understanding achievable tolerances, managing potential challenges, and carefully selecting the right manufacturing partner are all crucial elements for success.
The journey involves leveraging advanced materials and processes, implementing rigorous quality control, and often collaborating closely with AM experts. Companies like Met3dp stand at the forefront of this technological wave, offering not just state-of-the-art printing equipment (including SEBM and L-PBF systems) and high-performance metal powders produced via cutting-edge atomization techniques, but also the comprehensive expertise needed to navigate the complexities of industrial additive manufacturing. Their commitment to enabling next-generation manufacturing makes them an ideal partner for automotive companies looking to harness the power of AM.
For engineers and procurement managers aiming to push the boundaries of vehicle design and performance, metal AM presents a tangible opportunity. Lightweighting critical components like brake pedal arms contributes directly to enhanced vehicle dynamics, extended EV range, and overall system efficiency.
Ready to explore how metal additive manufacturing can revolutionize your automotive components? Návštěva Met3dp.com or contact their team today to discuss your project requirements and discover how their advanced powders, printing systems, and application development services can accelerate your organization’s additive manufacturing goals.
Sdílet na
Facebook
Cvrlikání
LinkedIn
WhatsApp
E-mailem
MET3DP Technology Co., LTD je předním poskytovatelem řešení aditivní výroby se sídlem v Qingdao v Číně. Naše společnost se specializuje na zařízení pro 3D tisk a vysoce výkonné kovové prášky pro průmyslové aplikace.
Dotaz k získání nejlepší ceny a přizpůsobeného řešení pro vaše podnikání!
Související články
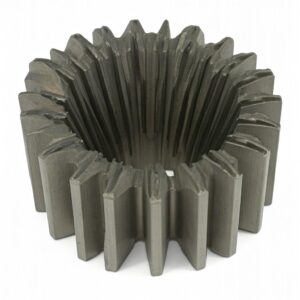
Vysoce výkonné segmenty lopatek trysek: Revoluce v účinnosti turbín díky 3D tisku z kovu
Přečtěte si více "
13. května 2025
Žádné komentáře
O Met3DP
Nedávná aktualizace
Náš produkt
KONTAKTUJTE NÁS
Nějaké otázky? Pošlete nám zprávu hned teď! Po obdržení vaší zprávy obsloužíme vaši žádost s celým týmem.
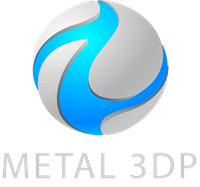
Kovové prášky pro 3D tisk a aditivní výrobu
SPOLEČNOST
PRODUKT
kontaktní informace
- Město Qingdao, Shandong, Čína
- [email protected]
- [email protected]
- +86 19116340731