3D Printed Heat Sinks for Electric Motor Cooling
Obsah
Introduction: Revolutionizing Electric Motor Thermal Management with Metal 3D Printing
Electric motors are the workhorses of modern industry, powering everything from electric vehicles (EVs) and aerospace actuators to industrial robots and medical devices. However, as performance demands increase – higher power density, faster speeds, and more compact designs – effective thermal management becomes paramount. Overheating is a primary cause of reduced efficiency, decreased lifespan, and catastrophic failure in electric motors. Traditional heat sinks, often manufactured through casting or extrusion, face limitations in handling the complex cooling requirements of advanced motor designs. This is where metal additive manufacturing (AM), or 3D tisk, emerges as a transformative technology, enabling the creation of highly optimized, complex electric motor cooling solutions previously impossible to produce.
Metal 3D printing allows engineers to break free from the constraints of conventional manufacturing. Instead of subtracting material from a block, AM builds parts layer by layer directly from a digital model using high-performance metal powders. This additive approach unlocks unprecedented design freedom, making it possible to create intricate internal cooling channels, topology-optimized geometries, and lightweight structures tailored precisely to the thermal profile of a specific electric motor. For procurement managers and engineers seeking cutting-edge řešení tepelného managementu, understanding the potential of additive manufacturing heat sinks is crucial for staying competitive. These advanced components offer superior heat dissipation, enabling motors to run cooler, longer, and more efficiently, particularly in demanding průmyslové aplikace. Companies like Met3dp, specializing in both advanced 3D tisk z kovu equipment and high-quality metal powders, are at the forefront of this revolution, providing the tools and materials necessary to realize these next-generation thermal solutions. This shift towards AM represents not just an incremental improvement but a fundamental change in how we approach the design and production of critical components like electric motor heat sinks.
The challenge of effectively dissipating heat generated by stator windings and rotor assemblies intensifies as motor designs become more power-dense and integrated into confined spaces. Conventional heat sinks, typically featuring straight fins attached to a base plate, rely primarily on maximizing surface area exposed to a cooling medium (air or liquid). While effective to a point, their performance is often limited by the manufacturing process itself. Extrusion limits fin shapes and designs, while casting can introduce porosity and limit geometric complexity and minimum feature sizes. Machining complex shapes from solid blocks is possible but often prohibitively expensive and wasteful.
Metal 3D printing overcomes these hurdles by enabling:
- Unparalleled Geometric Complexity: AM can produce organic shapes, intricate lattice structures, and conformal cooling channels that follow the exact contours of motor components. This allows heat to be extracted more directly and efficiently from hotspots.
- Topology Optimization: Using computational analysis, designs can be optimized to place material only where it’s structurally or thermally necessary, resulting in significantly lighter components with equivalent or superior cooling performance.
- Konsolidace částí: Multiple components of a thermal management assembly (e.g., heat sink, mounting brackets, flow directors) can potentially be consolidated into a single 3D printed part, reducing assembly time, potential leak points, and overall system complexity.
- Rychlé prototypování a iterace: Design changes can be implemented and tested quickly without the need for expensive tooling modifications, accelerating development cycles for new motor cooling solutions.
- Use of Advanced Materials: AM processes can effectively work with materials optimized for thermal conductivity and strength, such as specific copper and aluminum alloys, which might be difficult or costly to process using traditional methods.
For businesses involved in the supply chain for electric motors – from component suppliers to original equipment manufacturers (OEMs) – embracing metal AM for heat sinks offers a distinct competitive advantage. It facilitates the development of higher-performance, more reliable, and potentially more cost-effective electric motors, meeting the stringent demands of industries like automotive (especially EVs), aerospace, robotics, and high-performance computing. Procurement professionals looking for reliable metal AM suppliers capable of producing high-quality, intricate heat sinks in materials like Copper Chromium Zirconium (CuCrZr) or Aluminum Silicon Magnesium (AlSi10Mg) are increasingly turning to specialized providers who understand both the printing process and the critical application requirements. The integration of simulation-driven design with advanced manufacturing capabilities promises a future where thermal bottlenecks are systematically engineered out of electric motor systems.
What are 3D Printed Electric Motor Heat Sinks Used For?
The applications for 3D printed electric motor heat sinks span a wide range of industries where high performance, efficiency, and reliability are critical. The ability to create custom, highly efficient cooling solutions makes metal AM particularly valuable in scenarios where conventional heat sinks fall short due to space constraints, weight limitations, or extreme thermal loads. Key application areas include:
- Electric Vehicles (EVs) and Automotive:
- Traction Motors: The main propulsion motors in EVs generate significant heat, especially during high acceleration or regenerative braking. EV thermal management is critical for range, performance, and battery life. 3D printed heat sinks with complex internal channels for liquid cooling or optimized fin structures for air cooling can significantly enhance heat dissipation in compact powertrain assemblies. They allow for more power-dense motor designs, contributing to vehicle lightweighting and efficiency.
- On-Board Chargers & Inverters: Power electronics components also require robust cooling. Custom 3D printed cold plates or heat sinks can be integrated directly into these units for optimal thermal performance.
- Motorsport: In racing applications, every gram counts, and maximum performance is essential. Topology-optimized, lightweight 3D printed heat sinks provide a competitive edge by ensuring motors operate at peak efficiency under extreme conditions.
- Letectví a obrana:
- Actuation Systems: Electric motors drive flight control surfaces, landing gear, and other critical systems. Reliability is paramount, and components must withstand harsh operating conditions (temperature fluctuations, vibrations). Aerospace motor components often require lightweight, high-strength materials and optimized thermal management. 3D printed heat sinks, often made from specialized aluminum or copper alloys, meet these demands, offering tailored cooling in tightly packaged avionics bays.
- Drone Propulsion: High-power-density motors in drones and UAVs benefit significantly from lightweight, efficient cooling solutions. 3D printed heat sinks enable longer flight times and higher payload capacities.
- Radar and Electronics Cooling: Ground-based and airborne electronic systems generate substantial heat. Custom AM heat sinks provide efficient thermal pathways.
- Industrial Automation and Robotics:
- Servo Motors and Drives: Precision movements in robotics and automated assembly lines rely on motors that operate consistently. Overheating can lead to positioning errors and reduced component life. Industrial automation cooling solutions using 3D printed heat sinks ensure thermal stability, enhancing accuracy and uptime.
- High-Torque Motors: Motors used in heavy lifting or continuous operation machinery generate considerable heat. AM allows for robust heat sinks integrated directly into the motor housing or cooling system.
- Manufacturing Equipment: Motors within CNC machines, conveyors, and other factory equipment benefit from improved thermal management provided by custom 3D printed solutions.
- Lékařské přístroje:
- Surgical Robots and Tools: Motors in medical robotics require precise control and high reliability. Compact, efficient cooling is often necessary, making 3D printed heat sinks an ideal solution.
- Medical Imaging Equipment (MRI/CT Scanners): Components within these systems may utilize motors that require specific thermal management profiles, achievable through custom AM heat sinks.
- High-Performance Computing and Electronics:
- Liquid Cooling Systems: While not electric motors, the principles apply. 3D printing enables complex cold plates and manifolds for direct liquid cooling of high-power processors and electronics, pushing the boundaries of performance.
Why Customization Matters:
The key advantage across these applications is the ability to create custom heat sink solutions. Unlike off-the-shelf heat sinks, 3D printed versions can be:
- Perfectly Conformal: Designed to match the exact shape of the motor housing or specific hot spots.
- Integrated: Combined with other functional elements like mounts or fluid connectors.
- Optimized: Tailored for specific flow rates (liquid cooling) or airflow patterns (air cooling).
- Lightweighted: Using topology optimization and lattice structures to reduce mass without compromising thermal performance.
Procurement managers sourcing high-performance motor cooling components should recognize that metal 3D printing offers a pathway to solutions that are not just replacements for traditional parts but significant upgrades, enabling higher performance and greater design flexibility across numerous demanding sectors. Identifying heat sink manufacturers with proven AM expertise is key to leveraging this technology effectively.
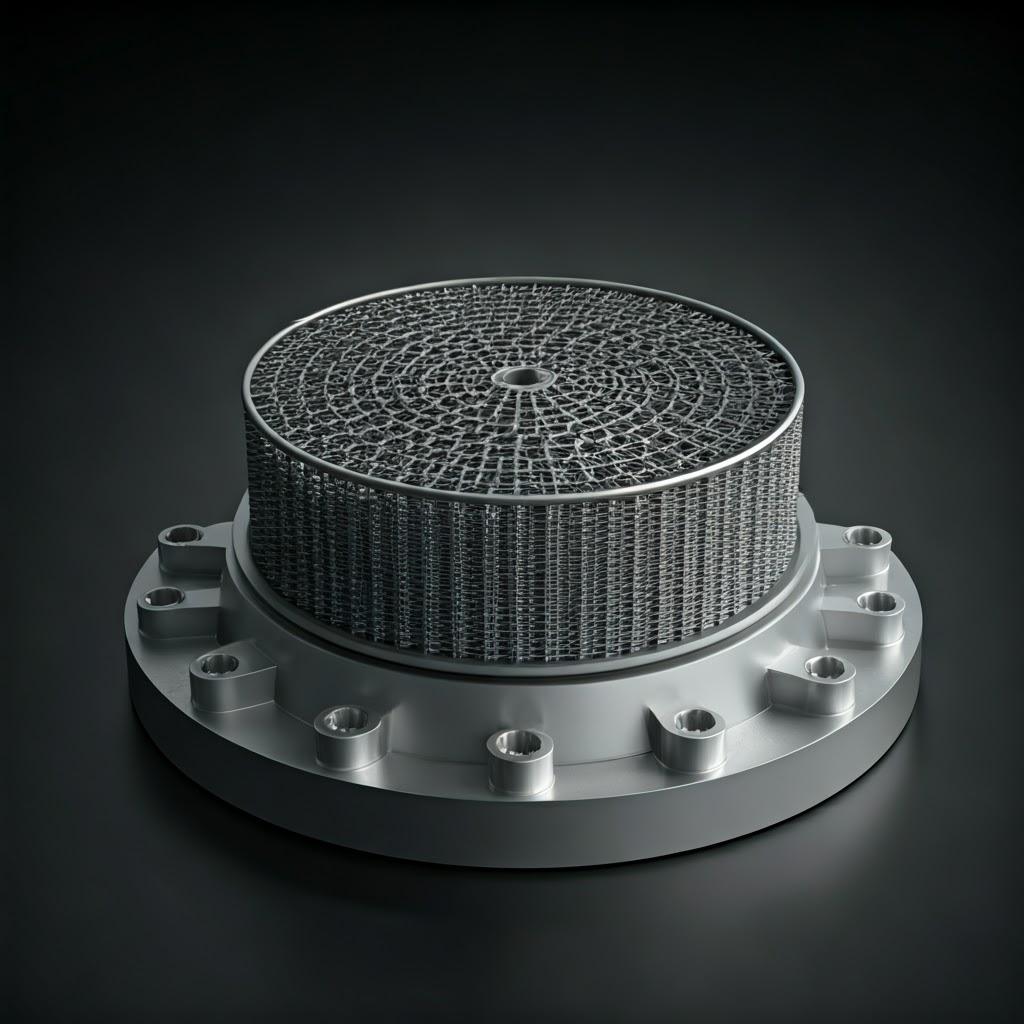
Why Use Metal 3D Printing for Electric Motor Heat Sinks? The Additive Advantage
Choosing metal additive manufacturing (AM) for producing electric motor heat sinks isn’t just about adopting a novel technology; it’s about leveraging distinct advantages that directly address the limitations of traditional manufacturing methods (like casting, extrusion, and machining) in the context of advanced thermal management. For engineers designing next-generation electric motors and procurement specialists sourcing high-performance components, understanding these benefits is crucial for making informed decisions. The additive advantage translates into superior performance, reduced weight, faster development cycles, and enhanced design possibilities.
Here’s a breakdown of why metal 3D printing is increasingly the preferred method for demanding heat sink applications:
- Unmatched Design Freedom for Complex Geometries:
- Challenge with Traditional Methods: Extrusion limits designs to 2.5D shapes with straight fins. Casting allows more complexity but struggles with thin walls, intricate internal channels, and undercuts without complex (and costly) tooling. Machining complex internal features is often impossible or requires assembling multiple parts.
- AM Advantage: 3D printing builds parts layer-by-layer, enabling the creation of highly complex heat sink geometries. This includes:
- Internal Cooling Channels: Sophisticated, smoothly curved channels for liquid cooling can be integrated directly within the heat sink body, following the contours of the motor’s heat sources for maximum efficiency.
- Triply Periodic Minimal Surfaces (TPMS) & Lattice Structures: These complex, porous structures offer enormous surface area-to-volume ratios, ideal for enhancing convective heat transfer in both air and liquid-cooled applications. They are virtually impossible to make traditionally.
- Conformal Designs: Heat sinks can be shaped to perfectly hug the motor casing or specific components, minimizing thermal resistance and improving heat extraction.
- Optimized Fin Shapes: Fins can be designed with variable thickness, curvature, and density, precisely tuned through simulation for optimal airflow or fluid flow interaction.
- Topology Optimization and Lightweighting:
- Challenge with Traditional Methods: Designs are often over-engineered with uniform material distribution because removing material precisely where it isn’t needed is difficult or costly.
- AM Advantage:Optimalizace topologie software can analyze the thermal and structural loads on a heat sink and algorithmically determine the most efficient material distribution. AM can then fabricate these optimized, often organic-looking designs. This leads to:
- Significant Weight Reduction: Removing unnecessary material drastically reduces component weight, which is critical in aerospace, automotive (especially EVs), and robotics applications.
- Material Savings: Less material used translates to lower raw material costs, especially important when using more expensive high-conductivity alloys like copper.
- Improved System Performance: Lighter components contribute to better overall system dynamics and efficiency (e.g., increased vehicle range, faster robotic movements).
- Enhanced Thermal Efficiency:
- Challenge with Traditional Methods: Design limitations often result in sub-optimal thermal pathways and lower overall heat dissipation capacity for a given volume or weight. Joining multiple parts (e.g., base plate and fins) introduces thermal contact resistance.
- AM Advantage: The ability to create complex internal structures, conformal designs, and integrate features like turbulators or optimized fin arrays leads to improved thermal efficiency.
- Targeted Cooling: Heat can be drawn away more effectively from specific hotspots.
- Increased Surface Area: Lattice structures and complex fin designs maximize the heat transfer surface within a given volume.
- Reduced Thermal Resistance: Single-piece construction eliminates contact resistance between joined parts. Performance gains of 20-50% or more compared to traditionally manufactured heat sinks of similar size are often reported.
- Rychlá tvorba prototypů a opakování návrhu:
- Challenge with Traditional Methods: Creating prototypes often requires dedicated tooling (molds, dies) or extensive machining setup. Iterating on a design is slow and expensive, hindering optimization.
- AM Advantage: AM allows for rapid prototyping heat sinks directly from CAD models.
- Rychlost: Functional metal prototypes can often be produced in days rather than weeks or months.
- Efektivita nákladů: No tooling investment is needed for prototypes or low-volume production runs.
- Flexibilita: Designs can be easily modified and reprinted, allowing engineers to quickly test and validate different cooling strategies (e.g., varying channel designs, fin patterns, or lattice densities). This accelerates the product development cycle significantly.
- Konsolidace částí:
- Challenge with Traditional Methods: Complex cooling assemblies often require multiple components (heat sink base, fins, brackets, manifolds, connectors) that need to be manufactured separately and then assembled.
- AM Advantage: 3D printing enables the consolidation of multiple functions into a single, monolithic part. For instance, mounting features, fluid inlet/outlet ports, and the heat exchange structure can all be printed as one piece. This reduces:
- Assembly time and labor costs.
- Potential leak points (in liquid-cooled systems).
- Part count and inventory management complexity.
- Overall system weight and volume.
- Enabling Advanced Materials:
- Challenge with Traditional Methods: Some high-performance materials, particularly certain copper alloys optimized for thermal conductivity and strength, can be difficult or expensive to cast or machine accurately into complex shapes.
- AM Advantage: Powder Bed Fusion (PBF) processes, like Selective Laser Melting (SLM) or Electron Beam Melting (EBM), are well-suited for processing a range of advanced metal powders, including specialized copper alloys (like CuCrZr) a aluminum alloys (like AlSi10Mg), tailored for thermal applications. Companies like Met3dp utilize advanced powder manufacturing techniques (e.g., gas atomization) to produce spherical, high-flowability powders ideal for achieving dense, high-performance AM parts.
Traditional vs. Additive Manufacturing for Heat Sinks: A Comparative Overview
Vlastnosti | Traditional Manufacturing (Casting, Extrusion, Machining) | Aditivní výroba kovů (3D tisk) | Advantage of AM |
---|---|---|---|
Geometric Complexity | Limited (especially internal features) | Very High (internal channels, lattices, organic shapes) | Enables optimized, conformal designs for superior thermal performance. |
Optimalizace topologie | Difficult / Impractical | Readily Applicable | Significant lightweighting potential, material savings. |
Konsolidace částí | Difficult / Requires Assembly | Possible to integrate multiple functions into one part | Reduced assembly cost, fewer leak points, lower part count. |
Prototyping Speed | Slow (requires tooling/setup) | Fast (direct from CAD) | Accelerates design iteration and validation. |
Náklady na nástroje | High (especially for complex shapes or low volumes) | Žádný | Cost-effective for custom parts, prototypes, and low-to-medium volumes. |
Materiálový odpad | High (Machining – Subtractive) | Low (Additive – uses only needed material + recyclable powder) | More sustainable, lower effective material cost in some cases. |
Tepelný výkon | Good, but often limited by manufacturability | Potentially Excellent (optimized designs, complex features) | Higher heat dissipation capacity for a given size/weight. |
Lead Time (Production) | Can be fast for high volumes (extrusion), slow (casting) | Moderate, scalable with machine capacity | Competitive for complex parts, potentially faster for low/medium volumes. |
Možnosti materiálu | Wide range, but some alloys difficult to process | Growing range, excels with specific AM-optimized alloys | Enables use of high-performance Cu/Al alloys for thermal management. |
Export to Sheets
In summary, the decision to use metal 3D printing for electric motor heat sinks is driven by the need for performance characteristics that push beyond the limits of traditional manufacturing. For procurement managers and engineers evaluating řešení tepelného managementu, the additive advantage offers a compelling pathway to creating lighter, more efficient, and highly customized components essential for the next generation of electric motors. Partnering with a knowledgeable metal AM service provider is key to unlocking this potential.
Recommended Materials for 3D Printed Heat Sinks: CuCrZr & AlSi10Mg Deep Dive
Selecting the right material is fundamental to the performance of any heat sink, especially those produced via metal additive manufacturing. The choice directly impacts thermal conductivity, mechanical strength, weight, corrosion resistance, printability, and ultimately, cost. For 3D printed electric motor heat sinks, two materials stand out due to their excellent balance of properties: Copper Chromium Zirconium (CuCrZr) a Aluminum Silicon Magnesium (AlSi10Mg). Understanding the nuances of these alloys is critical for engineers specifying components and procurement managers sourcing materials or services.
Why Material Choice Matters in AM Heat Sinks:
- Tepelná vodivost: The primary function of a heat sink is to conduct heat away from the source. Higher thermal conductivity allows for more efficient heat transfer.
- Mechanické vlastnosti: The heat sink must withstand operational stresses, vibrations, and mounting forces. Strength, hardness, and fatigue resistance are important considerations.
- Hmotnost: Especially crucial in automotive and aerospace, lower density materials contribute to lightweighting.
- Možnost tisku: Not all alloys are easily processed using AM. Factors like laser absorption, melt pool stability, solidification behavior, and susceptibility to cracking influence the quality and feasibility of printing.
- Následné zpracování: Requirements for heat treatment, surface finishing, or machining vary between materials and impact final properties and cost.
- Náklady: Raw material powder costs and the complexity/speed of the printing process influence the final part price.
Let’s delve into the specifics of the recommended powders:
1. Copper Chromium Zirconium (CuCrZr) – The High-Conductivity Champion
CuCrZr is a precipitation-hardenable copper alloy renowned for its excellent combination of high thermal and electrical conductivity, good mechanical strength (especially at elevated temperatures), and resistance to softening.
- Key Properties & Advantages:
- Exceptional Thermal Conductivity: Typically >300 W/(m·K) after appropriate heat treatment. This is significantly higher than aluminum alloys and most steels, allowing for highly efficient heat dissipation and potentially more compact heat sink designs for a given cooling requirement.
- Vysoká elektrická vodivost: Often exceeding 80% IACS (International Annealed Copper Standard). While less critical for the heat sink function itself, it can be beneficial if the component serves a secondary electrical role or is in close proximity to conductive elements.
- Good Mechanical Strength: Achieves respectable tensile strength and hardness through precipitation hardening (heat treatment), making it suitable for applications with moderate mechanical loads. Retains strength better at elevated temperatures compared to pure copper or some aluminum alloys.
- Stress Relaxation Resistance: Important for maintaining clamping forces if the heat sink is part of a bolted assembly operating at higher temperatures.
- Výzvy a úvahy:
- Možnost tisku: Copper alloys can be challenging to print using laser-based PBF due to copper’s high reflectivity and thermal conductivity, which can lead to unstable melt pools or require high laser power. Process parameter optimization is critical. Electron Beam Melting (EBM) can sometimes be advantageous for copper.
- Náklady: Copper alloy powders are generally more expensive than aluminum alloy powders.
- Hustota: Copper is significantly denser (~8.9 g/cm³) than aluminum (~2.7 g/cm³), making it less suitable for applications where weight is the absolute primary constraint, unless the superior thermal performance allows for a much smaller design.
- Následné zpracování: Requires specific heat treatment cycles (solutionizing and aging) to achieve optimal properties. Support removal can also be challenging.
- Typické aplikace: Demanding applications requiring maximum thermal performance in a constrained volume, high-performance electronics cooling, heat exchangers where space is limited, induction coils, resistance welding electrodes (leveraging electrical conductivity too). Ideal for high-performance motor cooling where thermal loads are extreme.
- Met3dp Advantage: Sourcing high-quality CuCrZr powder is crucial for successful printing. Met3dp utilizes advanced gas atomization techniques to produce spherical CuCrZr powders with excellent flowability and consistent particle size distribution, optimized for AM processes. Their expertise ensures powders meet the stringent requirements for density and performance in demanding thermal applications.
2. Aluminum Silicon Magnesium (AlSi10Mg) – The Versatile Lightweight
AlSi10Mg is one of the most common and well-understood aluminum alloys used in metal AM. It’s essentially a casting alloy adapted for additive manufacturing, offering a good balance of thermal properties, mechanical strength, low weight, and excellent printability.
- Key Properties & Advantages:
- Good Thermal Conductivity: While significantly lower than CuCrZr (typically in the range of 120-150 W/(m·K) after stress relief/heat treatment), it is still substantially better than steels or titanium alloys and sufficient for many motor cooling applications.
- Vynikající tisknutelnost: AlSi10Mg is relatively easy to process using Laser Powder Bed Fusion (LPBF/SLM). It has good laser absorption and forms stable melt pools, allowing for fine features and complex geometries to be produced reliably.
- Nízká hustota: With a density of approximately 2.68 g/cm³, it’s ideal for odlehčení applications in automotive, aerospace, and robotics.
- Dobrý poměr pevnosti a hmotnosti: Offers decent mechanical properties (yield strength, tensile strength) after printing, which can be further tailored with heat treatments (like T6).
- Odolnost proti korozi: Exhibits good resistance to atmospheric corrosion.
- Efektivita nákladů: Aluminum alloy powders are generally less expensive than copper alloys, and the faster, more established printing processes contribute to lower overall part costs.
- Výzvy a úvahy:
- Lower Thermal Conductivity: Cannot match copper alloys where extreme heat flux or minimal temperature gradients are required. Heat sinks may need to be larger compared to a CuCrZr equivalent for the same thermal load.
- Lower High-Temperature Performance: Mechanical properties degrade more significantly at elevated temperatures compared to CuCrZr.
- Anizotropie: As-printed AlSi10Mg parts can exhibit anisotropic mechanical properties (different strength depending on the build direction), which needs consideration during design and may necessitate specific heat treatments.
- Typické aplikace: Wide range of heat sinks where a good balance of performance, weight, and cost is needed. Ideal for EV thermal management components (motor housings with integrated cooling, inverter coolers), aerospace motor components where weight is critical, drone components, and general industrial automation cooling.
- Met3dp Advantage: Met3dp provides high-quality AlSi10Mg powder suitable for demanding applications. Their focus on powder sphericity and controlled particle size distribution ensures good powder bed density and flowability, leading to robust and reliable printed parts. Their experience with various tiskových metod allows them to advise on the optimal process parameters for achieving desired density and mechanical properties with AlSi10Mg.
Material Selection Guide: CuCrZr vs. AlSi10Mg for Heat Sinks
Vlastnosti | CuCrZr | AlSi 10Mg | Kritéria výběru |
---|---|---|---|
Tepelná vodivost | Excellent (>300 W/(m·K)) | Good (120-150 W/(m·K)) | Choose CuCrZr for highest heat flux, minimal temperature rise, compact designs. |
Hustota | High (~8.9 g/cm³) | Low (~2.7 g/cm³) | Choose AlSi10Mg for critical lightweighting applications. |
Printability (Laser PBF) | Challenging (reflectivity, conductivity) | Excellent (well-established process) | AlSi10Mg is generally easier/faster to print reliably. |
Mechanická pevnost | Good (especially at high temps) | Good (excellent strength-to-weight) | Evaluate based on operating temperature and load requirements. |
Náklady na suroviny | Vysoký | Mírný | AlSi10Mg offers a lower material cost baseline. |
Post-Processing (Heat Treat) | Required (Precipitation Hardening) | Optional/Recommended (Stress Relief/T6) | Factor in heat treatment complexity and cost. |
Primary Benefit | Maximum Thermal Performance | Lightweight & Versatility | Match benefit to the most critical application requirement. |
Export to Sheets
Conclusion on Materials:
The choice between CuCrZr and AlSi10Mg depends heavily on the specific requirements of the electric motor and its operating environment.
- Choose CuCrZr when: Absolute maximum thermal performance is needed, space is highly constrained, operating temperatures are elevated, and weight is a secondary concern compared to heat dissipation.
- Choose AlSi10Mg when: Lightweighting is a primary driver, good thermal performance is sufficient, cost-effectiveness is important, and complex geometries need to be printed reliably.
Engaging with a knowledgeable additive manufacturing partner like Met3dp, who understands both the materials science and the printing processes, is essential. They can provide guidance on material selection, design optimization for the chosen material, and ensure the use of high-quality, AM-optimized kovové prášky for producing reliable and high-performance 3D printed heat sinks tailored to your specific electric motor cooling needs. Procurement managers seeking wholesale nebo hromadné supply of these powders or finished components should look for suppliers with robust quality control and material traceability.
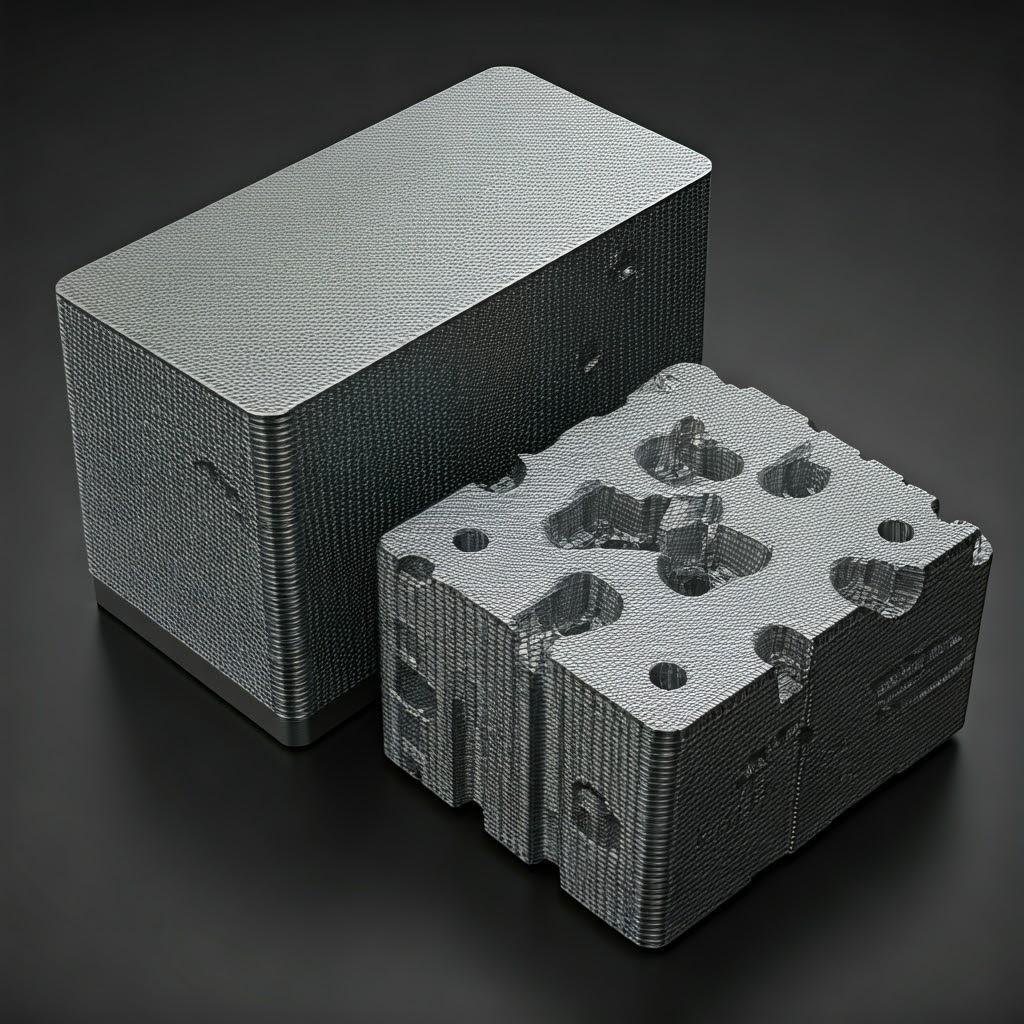
Design Considerations for Additively Manufactured Heat Sinks
The true potential of metal 3D printing for electric motor heat sinks is unlocked through intelligent design – specifically, Design for Additive Manufacturing (DfAM). Simply replicating a traditionally designed heat sink using AM often yields minimal benefits and can even be counterproductive. DfAM involves rethinking the component’s architecture from the ground up to leverage the unique capabilities of layer-by-layer fabrication, focusing on maximizing thermal performance, minimizing weight, and ensuring manufacturability. Engineers and designers creating industrial heat sink designs for AM must consider several key factors beyond those typical for conventional methods.
1. Embrace Geometric Freedom: Beyond Fins and Blocks
- Topology Optimization (TO): This is arguably the most powerful tool in the DfAM arsenal for heat sinks.
- Proces: Define the design space (maximum allowable volume), non-design regions (interfaces, mounting points), load cases (thermal loads, structural forces), objectives (minimize mass, minimize thermal resistance), and constraints (maximum stress, minimum feature size). TO software then iteratively removes material from low-stress/low-impact areas, leaving behind an optimized, often organic-looking structure.
- Výhody: Významné odlehčení (reductions of 30-70% are common compared to baseline designs) while maintaining or even improving thermal and structural performance. Reduces material consumption and print time.
- Tools: Software like Altair Inspire, nTopology, Autodesk Fusion 360, Dassault Systèmes CATIA/SOLIDWORKS offer TO modules.
- Úvahy: Optimized shapes can be complex to model cleanly for printing; requires careful mesh refinement and sometimes manual remodeling based on TO results. Ensure the optimization considers manufacturing constraints (e.g., minimum printable feature size, overhang angles).
- Lattice Structures & Triply Periodic Minimal Surfaces (TPMS):
- Concept: Instead of solid material, replace internal volumes or fin structures with porous, repeating unit cells (lattices) or mathematically defined surfaces (TPMS like Gyroid, Schwarz P, Diamond).
- Výhody: Drastically increases the surface area-to-volume ratio, enhancing convective heat transfer for both air and liquid cooling. Allows for controlled porosity and fluid flow characteristics. Excellent for lightweighting. TPMS structures offer smooth, self-supporting surfaces that can be beneficial for fluid flow and reducing stress concentrations compared to strut-based lattices.
- Design: Select unit cell type, size, and strut/wall thickness based on desired thermal performance, fluid flow (pressure drop), structural requirements, and printability. Graded lattices (varying density) can further optimize performance.
- Použití: Ideal for creating highly efficient heat exchange zones within liquid cooling cold plates or for maximizing air convection in forced or natural convection heat sinks.
- Konformní chladicí kanály:
- Concept: For liquid-cooled heat sinks, internal channels are designed to follow the exact contours of the heat-generating components (e.g., motor stator, windings).
- Výhody: Minimizes the distance heat needs to travel, reduces thermal resistance, eliminates hotspots, and allows for more uniform cooling compared to traditional drilled or milled channels. Enables cooling in previously inaccessible areas.
- Design Rules: Consider channel diameter (balancing flow rate and pressure drop), minimum wall thickness between channels and external surfaces, achievable curvature radii (avoiding sharp bends), and integration of inlet/outlet ports. Smooth internal surfaces are desirable to minimize pressure drop.
2. Designing for Manufacturability (The “A” in DfAM)
- Minimize Support Structures: Support structures are often necessary in Powder Bed Fusion (PBF) processes (like SLM/LPBF) to anchor parts to the build plate, support overhanging features (typically angles below 45 degrees from the horizontal), and dissipate heat. However, they add print time, consume material, require post-processing for removal, and can mar the surface finish.
- Strategies:
- Orientation: Optimize part orientation on the build plate to minimize the extent and complexity of overhangs.
- Self-Supporting Angles: Design features with angles greater than 45 degrees where possible.
- Internal Channels: Design channels with teardrop or diamond cross-sections instead of circular to make them self-supporting.
- Sacrificial Features: Incorporate features designed to be machined away later, which can act as supports during printing.
- Přístupnost: Ensure support structures are accessible for easy removal (manually or via machining).
- Strategies:
- Wall Thickness and Feature Size:
- Minimums: There are practical limits to how thin walls or fine features can be reliably printed. This depends on the material (e.g., AlSi10Mg generally allows finer features than CuCrZr due to melt pool stability), the machine’s laser spot size or beam characteristics, and process parameters. Typical minimum wall thicknesses might be 0.3-0.5 mm, but this needs verification with the metal AM service provider.
- Maximums: Very thick, solid sections can accumulate residual stress and potentially lead to distortion or cracking. Incorporating internal voids or lattice structures can mitigate this.
- Důslednost: Avoid abrupt changes in cross-section, which can exacerbate thermal stresses. Use generous fillets and radii.
- Residual Stress Management: Thermal cycles during printing create internal stresses.
- Design Impact: Sharp corners, large flat surfaces parallel to the build plate, and rapid changes in geometry can increase residual stress. Design with these factors in mind.
- Mitigation: Proper support strategies, optimized orientation, and post-print stress relief heat treatments are crucial (discussed later).
3. Simulation-Driven Design:
- CFD (Computational Fluid Dynamics): Essential for optimizing heat sink performance. Simulate fluid flow (air or liquid) through the heat sink geometry to predict pressure drop, flow distribution, velocity profiles, and heat transfer coefficients. Iterate designs based on CFD results to maximize cooling efficiency.
- FEA (Finite Element Analysis): Analyze thermal stresses, mechanical stresses under load, and vibration response. Ensure the design can withstand operating conditions. FEA is also crucial for topology optimization setup and validation.
- Build Process Simulation: Specialized software can simulate the AM build process itself, predicting potential issues like distortion, overheating of overhangs, or blade crashes (recoater interference). This allows for preemptive design adjustments or optimization of build parameters and supports.
4. Material-Specific Considerations:
- CuCrZr: High thermal conductivity means heat spreads rapidly, potentially requiring more robust support structures for thermal management during the build. Its reflectivity might necessitate specific laser parameters or strategies. Designs should account for the volumetric changes during precipitation hardening heat treatment.
- AlSi10Mg: Generally more forgiving to print. Its lower melting point and good laser absorption allow for faster build speeds and potentially finer features. Designs should consider the need for stress relief or T6 heat treatment to achieve desired properties and dimensional stability.
DfAM Services and Expertise:
Successfully implementing these design considerations often requires specialized knowledge. Partnering with a company offering comprehensive 3D tisk z kovu solutions, like Met3dp, can be invaluable. They possess expertise in DfAM principles, material behavior, simulation, and process optimization, helping clients translate performance requirements into printable, high-performance heat sink designs. Effective DfAM services bridge the gap between concept and successful additive manufacturing.
Summary Table: Key DfAM Considerations for AM Heat Sinks
Úvaha | Key Actions & Techniques | Benefit |
---|---|---|
Geometrická svoboda | Topology Optimization, Lattice Structures/TPMS, Conformal Cooling Channels | Maximize performance (thermal/structural), Lightweighting, Unique functionality |
Support Minimization | Optimize Orientation, Design Self-Supporting Angles/Channels, Ensure Accessibility | Reduce print time/cost, Simplify post-processing, Improve surface finish |
Feature/Wall Size | Adhere to minimum/maximum printable limits, Ensure gradual transitions, Use fillets | Ensure printability, Maintain structural integrity, Reduce stress concentrations |
Zbytkové napětí | Avoid large flat areas/sharp corners, Optimize supports, Plan for stress relief heat treatment | Prevent distortion/cracking, Ensure dimensional stability |
Simulace | Use CFD for thermal/fluid performance, FEA for structural/thermal stress, Build Simulation | Optimize design before printing, Predict performance, Mitigate build failures |
Výběr materiálu | Design according to specific material printability, thermal behavior, post-processing needs | Ensure successful printing and achieve desired final properties |
Export to Sheets
By embracing these DfAM principles, manufacturers can fully exploit the advantages of metal 3D printing to create next-generation electric motor heat sinks that outperform their traditional counterparts in efficiency, weight, and integration.
Achievable Tolerance, Surface Finish, and Dimensional Accuracy in 3D Printed Heat Sinks
While metal 3D printing offers incredible geometric freedom, it’s crucial for engineers and procurement managers to have realistic expectations regarding the achievable dimensional tolerances, surface finish, and overall accuracy of the printed parts. These factors directly impact the heat sink’s fit, assembly, interfacing with other components, and potentially its fluid dynamic performance. Understanding the typical capabilities and influencing factors of processes like Laser Powder Bed Fusion (LPBF) and Electron Beam Melting (EBM) is essential when specifying precision AM components.
1. Dimensional Tolerances:
- General Expectations: As a rule of thumb, metal PBF processes can typically achieve tolerances comparable to metal investment casting. General tolerances are often cited in the range of ±0.1 mm to ±0.2 mm for the first 25 mm, and ±0.05 mm to ±0.1 mm for each subsequent 25 mm, but this is highly dependent on the specific geometry, size, material, machine calibration, and process parameters.
- ISO Standards: While specific AM tolerance standards are evolving, some manufacturers reference general tolerance standards like ISO 2768 (medium ‘m’ or fine ‘f’ classes) as a starting point, but AM-specific deviations are common. It’s best to discuss specific tolerance requirements with the AM service provider.
- Factors Influencing Tolerance:
- Machine Calibration: Accuracy of the laser/electron beam positioning system, scanner calibration, and layer thickness control.
- Vlastnosti materiálu: Thermal expansion/contraction during heating and cooling, shrinkage during solidification and cooling to room temperature. Different alloys behave differently.
- Thermal Stresses: Residual stresses built up during the process can cause warping and distortion, affecting final dimensions.
- Part Geometry & Size: Larger parts and complex geometries with varying cross-sections are generally harder to hold to tight tolerances due to cumulative stress and thermal effects.
- Podpůrné struktury: How the part is supported affects its stability during the build and potential distortion during removal.
- Build Orientation: The direction a feature is built relative to the layers can impact its dimensional accuracy.
- Následné zpracování: Stress relief heat treatments can cause minor dimensional changes. Machining operations are often required for critical tolerances.
- Critical Dimensions: For features requiring tighter tolerances than achievable in the as-built state (e.g., mating surfaces, bearing fits, port connections), secondary CNC machining is typically employed. It’s crucial to include extra material (machining allowance or stock) in the AM design for these features. Clearly define critical dimensions and tolerances using Geometric Dimensioning and Tolerancing (GD&T) on drawings.
2. Surface Finish (Roughness):
- As-Built Surface Roughness: The surface finish of as-built metal AM parts is inherently rougher than machined surfaces. It’s influenced by powder particle size, layer thickness, melt pool dynamics, and build orientation.
- Typical Values (Ra):
- Side Walls (Vertical): Often smoother, Ra typically 6-15 µm.
- Top Surfaces (Upward-facing): Can be relatively smooth depending on melt parameters, Ra 5-12 µm.
- Bottom Surfaces (Downward-facing/Supported): Tend to be the roughest due to support interaction or partially melted powder adherence, Ra can range from 15 µm to over 30 µm.
- Internal Channels: Often difficult to finish post-print, retaining a higher as-built roughness. This impacts pressure drop in fluid channels.
- Stair-Stepping Effect: Curved or angled surfaces built layer-by-layer exhibit a characteristic “stair-step” effect, contributing to roughness. Thinner layers reduce this effect but increase build time.
- Typical Values (Ra):
- Improving Surface Finish: If a smoother surface is required for aesthetic reasons, improved fatigue life, or fluid dynamics (reducing friction/pressure drop in channels), various post-processing techniques are used:
- Abrasive Blasting (Bead/Sand Blasting): Provides a uniform matte finish, removes loose powder. Ra typically 5-10 µm.
- Tumbling/Vibratory Finishing: Uses media to smooth surfaces and edges, particularly effective for batches of smaller parts.
- CNC Machining: Provides the best surface finish and accuracy for specific features.
- Polishing (Manual or Automated): Can achieve very smooth, mirror-like finishes (Ra < 1 µm) but is often labor-intensive and geometry-dependent.
- Electropolishing: An electrochemical process that removes a small layer of material, smoothing surfaces, particularly effective on complex shapes and internal channels if electrolyte flow can be managed.
3. Dimensional Accuracy & Quality Control:
- Verification: Ensuring the final part meets specifications requires robust quality control metal printing procedures.
- 3D Scanning: Non-contact optical scanners (laser or structured light) compare the final part geometry against the original CAD model, generating a deviation map. Useful for complex shapes.
- Coordinate Measuring Machines (CMM): Tactile probing provides highly accurate measurements of specific features and dimensions, essential for verifying critical tolerances.
- Process Monitoring: In-situ monitoring of the melt pool, layer deposition, and thermal conditions during the build can provide early indications of potential quality issues.
- Nedestruktivní zkoušení (NDT): Techniques like CT scanning (Computed Tomography) can inspect internal features and detect defects like porosity without damaging the part.
- Achieving High Accuracy:
- Collaboration: Close collaboration between the designer and the AM service provider (like Met3dp, with its focus on accuracy and reliability) is key. Discuss critical features, tolerances, and datums early in the process.
- Optimized Build Setup: Careful planning of part orientation, support strategy, and build parameters.
- Material Characterization: Understanding the specific shrinkage and thermal behavior of the chosen powder batch.
- Post-Processing Planning: Designing with necessary machining stock and planning appropriate stress relief and finishing steps.
Summary Table: Tolerance & Surface Finish in Metal AM Heat Sinks
Parametr | Typical As-Built Range | Influencing Factors | Možnosti dodatečného zpracování | Úvahy |
---|---|---|---|---|
Rozměrová tolerance | ±0.1 to ±0.2 mm (initial) + additional per length | Machine, Material, Geometry, Size, Supports, Orientation, Thermal Stress | CNC Machining (for critical features) | Specify critical tolerances via GD&T; Add machining stock. |
Drsnost povrchu (Ra) | 5-30+ µm (highly variable by surface orientation) | Powder Size, Layer Thickness, Melt Pool, Orientation, Supports | Blasting, Tumbling, Machining, Polishing, Electropolishing | Roughness impacts fluid flow (pressure drop); Internal channels hard to finish. |
Rozměrová přesnost | Dependent on control factors | Calibration, Process Control, Material Consistency, Post-Processing Stability | 3D Scanning, CMM Inspection, NDT (CT Scan) | Requires robust QC; Collaboration with provider is key. |
Export to Sheets
Procurement managers and engineers specifying 3D printed heat sinks should clearly communicate their tolerance and surface finish requirements. While AM offers geometric freedom, achieving tight tolerances and very smooth finishes often necessitates incorporating post-processing steps like CNC machining into the production plan and budget. Partnering with an experienced provider ensures these requirements are understood and met through optimized printing and appropriate finishing techniques.
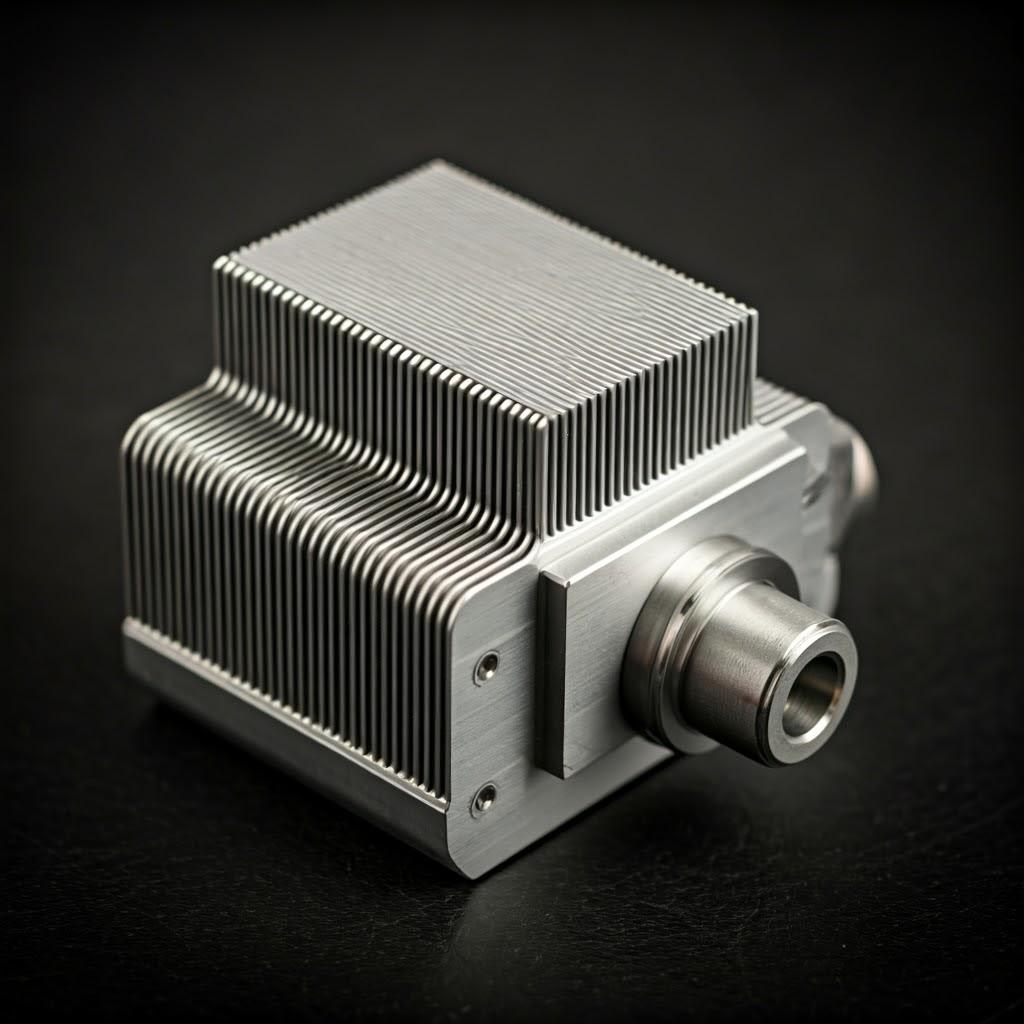
Essential Post-Processing Steps for 3D Printed Metal Heat Sinks
Producing a metal heat sink using additive manufacturing doesn’t end when the printer stops. The “as-built” part, fresh off the build plate, requires a series of essential post-processing steps to transform it into a functional, reliable component meeting performance and quality specifications. These steps are critical for relieving internal stresses, achieving final dimensions and surface finish, removing temporary support structures, and optimizing material properties. Understanding this workflow is vital for accurately estimating costs, lead times, and ensuring the final heat sink performs as intended.
The typical post-processing sequence for metal AM heat sinks (particularly using LPBF) involves:
- Powder Removal / Depowdering:
- Proces: Once the build chamber cools, the build plate with the attached part(s) is removed. Loose, unmelted powder surrounding and potentially inside the part must be carefully removed. This is typically done using vacuum systems, brushes, and compressed air within a controlled environment to contain the valuable metal powder for sieving and potential reuse.
- Importance: Ensures no loose powder interferes with subsequent steps or remains trapped within internal channels (critical for fluid flow). Thorough cleaning is essential.
- Stress Relief Heat Treatment (Often Done on Build Plate):
- Proces: Due to the rapid heating and cooling cycles during printing, significant residual stresses build up within the part. To prevent distortion or cracking when the part is cut from the build plate, a stress relief heat treatment is often performed before removal. The entire build plate with the part(s) is placed in a furnace and heated to a specific temperature (below the aging or annealing temperature, e.g., ~300°C for AlSi10Mg, higher for CuCrZr but below solutionizing temp), held for a period, and then slowly cooled. Inert atmosphere (Argon, Nitrogen) is typically required to prevent oxidation.
- Importance: Reduces internal stresses, significantly improving dimensional stability for subsequent steps and preventing unexpected warping. Critical for maintaining accuracy.
- Part Removal from Build Plate:
- Proces: The stress-relieved part needs to be separated from the build plate. Common methods include:
- Drátové elektroerozivní obrábění (EDM): Precise method, good for complex or delicate interfaces, minimal mechanical stress introduced.
- Bandsaw: Faster, more economical method suitable for simpler interfaces, but introduces more mechanical force.
- Obrábění: Milling or turning can also be used.
- Importance: Frees the individual part for further processing. The chosen method depends on part geometry, material, required precision at the base, and cost considerations.
- Proces: The stress-relieved part needs to be separated from the build plate. Common methods include:
- Odstranění podpůrné konstrukce:
- Proces: The temporary support structures printed to anchor the part and support overhangs must be removed. This can be labor-intensive.
- Ruční odstranění: Breaking or cutting away supports using hand tools (pliers, cutters). Possible for accessible, weaker supports.
- CNC Machining: Milling or grinding away supports, often required for robust supports or critical surfaces where support contact occurred.
- EDM: Can be used for inaccessible internal supports in some cases.
- Importance: Essential for achieving the final part geometry and function. Poorly removed supports can leave witness marks, affect tolerances, or block internal channels. Design for support removal (DfSR) is a key DfAM principle.
- Proces: The temporary support structures printed to anchor the part and support overhangs must be removed. This can be labor-intensive.
- Property-Enhancing Heat Treatment (Material Dependent):
- Proces: Unlike stress relief, this step aims to achieve the final desired material microstructure and mechanical properties. The specific cycle depends heavily on the alloy:
- CuCrZr (Precipitation Hardening): Requires a two-stage process: 1) Žíhání roztoků: Heating to a high temperature (~950-1000°C) to dissolve chromium and zirconium into the copper matrix, followed by rapid quenching. 2) Stárnutí: Reheating to a lower temperature (~450-500°C) for a specific time to allow Cr and Zr precipitates to form, significantly increasing hardness, strength, and thermal conductivity. Precise control of temperature and time is crucial. Inert atmosphere required.
- AlSi10Mg (T6 Treatment): Often involves: 1) Řešení Ošetření: Heating (~520-540°C) to dissolve Mg₂Si precipitates. 2) Kalení: Rapid cooling (water or polymer) to trap elements in solution. 3) Artificial Aging: Reheating (~160-180°C) to precipitate fine Mg₂Si particles, increasing strength and hardness. Stress relief might be sufficient if only dimensional stability, not peak strength, is needed.
- Importance: Tailors the material properties (strength, hardness, conductivity, ductility) to meet the application requirements. This is often mandatory to achieve datasheet properties for alloys like CuCrZr. Specialized heat treatment services AM providers or in-house calibrated furnaces are needed.
- Proces: Unlike stress relief, this step aims to achieve the final desired material microstructure and mechanical properties. The specific cycle depends heavily on the alloy:
- Finishing & Surface Treatment:
- Proces: Depending on requirements, various finishing steps may be applied:
- Obrábění: CNC milling or turning to achieve tight tolerances on mating surfaces, seal faces, or port connections. Requires careful fixture design for potentially complex AM shapes.
- Abrasive Blasting (Bead/Sand): Creates a uniform matte finish, cleans surfaces.
- Tumbling / Vibratory Finishing: Smooths surfaces and edges, deburrs.
- Leštění: Achieves smooth, low-Ra surfaces for specific areas.
- Anodizing (for Aluminum): Improves corrosion resistance and wear resistance, can be decorative.
- Plating (Nickel, etc.): Can enhance wear resistance, corrosion protection, or solderability.
- Importance: Achieves final dimensional specifications, required surface texture (for aesthetics, flow, or contact), and adds protective layers if needed.
- Proces: Depending on requirements, various finishing steps may be applied:
- Cleaning and Inspection:
- Proces: Final cleaning to remove any machining fluids, blasting media, or residues. Comprehensive inspection using methods like CMM, 3D scanning, visual checks, and potentially NDT (like CT scanning for internal channel verification or defect detection) ensures the part meets all specifications before shipping.
- Importance: Guarantees the part quality, dimensional accuracy, and cleanliness required for the application, especially critical for fluid-carrying components.
Workflow Summary:
Build Plate -> Depowdering -> Stress Relief -> Part Removal -> Support Removal -> Heat Treatment (Properties) -> Finishing/Machining -> Cleaning & Inspection -> Final Part
Considerations for Procurement:
When sourcing 3D printed heat sinks, procurement managers must ensure potential suppliers have the necessary in-house capabilities or qualified partners for all required post-processing steps. The cost and lead time associated with these steps can be significant and must be factored into the total project scope. A vertically integrated provider like Met3dp, with expertise spanning powders, printing, and potentially coordinating necessary post-processing, can streamline this complex workflow.
Common Challenges in 3D Printing Heat Sinks and Mitigation Strategies
While metal additive manufacturing unlocks incredible possibilities for heat sink design and performance, the process is not without its challenges. Understanding potential pitfalls and implementing effective mitigation strategies is crucial for ensuring successful builds, achieving desired quality, and maintaining cost-effectiveness. Engineers designing parts and procurement managers selecting suppliers should be aware of these common issues.
1. Warping and Distortion:
- Výzva: Significant temperature gradients during the layer-by-layer melting and solidification process induce residual stresses within the part. If these stresses exceed the material’s yield strength or the anchoring force of supports, the part can warp, distort, or even detach from the build plate. Large flat surfaces are particularly susceptible.
- Causes: High thermal gradients, insufficient support, large cross-sectional areas, inappropriate scan strategies, material properties (high thermal expansion).
- Mitigation Strategies:
- Optimized Orientation: Orienting the part to minimize large flat surfaces parallel to the build plate and reduce thermal mass concentration.
- Robust Support Structures: Using well-designed supports (density, type, location) to anchor the part firmly and conduct heat away effectively. Consider thermal supports specifically designed for heat dissipation.
- Build Plate Heating: Preheating the build plate (common in LPBF, essential in EBM) reduces thermal gradients between the part and the plate.
- Optimized Scan Strategy: Using specific laser/beam scanning patterns (e.g., island scanning, checkerboard patterns) to manage heat distribution and reduce localized stress buildup.
- Design Modification: Adding ribs or corrugations to large flat areas, reducing abrupt changes in thickness.
- Stress Relief Heat Treatment: Performing this step before part removal is critical for stabilizing the component (as discussed previously).
2. Porosity:
- Výzva: The presence of small voids or pores within the printed material compromises its density, mechanical properties (especially fatigue strength), and potentially thermal conductivity. Porosity can be internal or surface-connected.
- Causes:
- Process Parameter Issues: Incorrect laser/beam power, scan speed, or focus can lead to incomplete melting (lack-of-fusion porosity) or keyholing (gas entrapment porosity).
- Kvalita prášku: Poor quality powder with irregular shapes, internal porosity, or satellites can hinder uniform powder spreading and melting. Contaminated powder (e.g., moisture) can release gas during melting. Sourcing vysoce kvalitní kovové prášky from reputable suppliers like Met3dp, who utilize advanced atomization and quality control, is crucial.
- Gas Entrapment: Shielding gas issues (improper flow, contamination) can allow atmospheric gases into the melt pool. Gases dissolved in the powder can also contribute.
- Mitigation Strategies:
- Parameter Optimization: Developing and using validated process parameters specific to the material, machine, and geometry. This requires significant expertise.
- Powder Quality Control: Using high-quality, spherical powder with good flowability and low internal gas content. Implementing proper powder handling, storage, and recycling protocols.
- Shielding Gas Management: Ensuring high-purity inert shielding gas (Argon or Nitrogen) with appropriate flow rates and chamber oxygen level monitoring.
- Izostatické lisování za tepla (HIP): A post-processing step involving high pressure and temperature to close internal pores. Effective but adds cost and lead time. Often required for critical aerospace or medical components.
3. Support Structure Removal Difficulties:
- Výzva: Supports must be strong enough to do their job during printing but easy enough to remove afterward without damaging the part surface or leaving inaccessible remnants, especially in complex internal channels.
- Causes: Overly robust support design, poor accessibility, supports fused too strongly to the part, delicate part features near supports.
- Mitigation Strategies:
- DfAM for Support Reduction: Designing parts with self-supporting angles (>45°) and optimizing orientation to minimize the need for supports.
- Smart Support Design: Using support types (e.g., tree supports, block supports with specific interface layers) that balance strength with removability. Software tools offer various strategies.
- Accessibility Planning: Ensuring tools (manual or CNC) can reach the supports for removal.
- Material Choice: Some materials are easier to remove supports from than others.
- Techniky následného zpracování: Utilizing appropriate removal methods (manual, machining, potentially EDM).
4. Cracking (Solidification or Liquation Cracking):
- Výzva: Cracks can form during solidification or in the heat-affected zone due to high thermal stresses, particularly in alloys susceptible to hot tearing or liquation cracking (like some high-strength aluminum or nickel alloys; less common but possible in AlSi10Mg under non-optimal conditions).
- Causes: Material susceptibility, high thermal gradients, high residual stress, part geometry (sharp corners, rapid thickness changes).
- Mitigation Strategies:
- Parameter Optimization: Fine-tuning laser/beam parameters to control cooling rates.
- Build Plate Heating / Chamber Temperature Control: Reducing thermal gradients.
- Scan Strategy Modification: Managing heat input and stress accumulation.
- Alloy Selection/Modification: Choosing alloys less prone to cracking or using modified compositions if possible.
- Design Changes: Using fillets/radii, avoiding problematic geometries.
5. Surface Finish Issues:
- Výzva: Achieving the desired surface finish can be hampered by factors inherent to the PBF process.
- Stair-Stepping: Inevitable on curved/angled surfaces due to layering.
- Partially Melted Powder: Powder particles adhering loosely to downward-facing surfaces or side walls.
- Dross Formation: Oxide formation or melt pool ejecta creating surface imperfections.
- Mitigation Strategies:
- Orientation Optimization: Building critical surfaces vertically or as top surfaces where possible.
- Thinner Layer Thickness: Reduces stair-stepping but increases build time.
- Parameter Optimization: Fine-tuning melt pool parameters to minimize powder adhesion and dross.
- Následné zpracování: Employing appropriate finishing techniques (blasting, tumbling, machining, polishing) as required.
6. Quality Assurance and Consistency:
- Výzva: Ensuring consistent quality and properties from part-to-part and batch-to-batch requires rigorous process control and validation.
- Causes: Variations in powder quality, machine calibration drifts, inconsistent atmospheric conditions, deviations in post-processing.
- Mitigation Strategies:
- Robust Quality Management System (QMS): Implementing ISO 9001 or industry-specific standards (AS9100 for aerospace).
- Process Monitoring: Utilizing in-situ monitoring tools to track build conditions.
- Material Traceability & Testing: Strict control over powder batches, including testing properties.
- Machine Calibration & Maintenance: Regular schedules to ensure machine accuracy.
- Standardized Procedures: Documented procedures for printing and all post-processing steps.
- Final Inspection & Testing: Comprehensive dimensional checks, NDT, and potentially destructive testing on sample coupons.
Successfully navigating these challenges requires a combination of good design practices (DfAM), careful material selection and handling, precise process control, appropriate post-processing, and robust quality assurance metal printing protocols. Partnering with an experienced AM provider who proactively addresses these potential issues is key for procurement managers seeking reliable production of high-quality 3D printed heat sinks.
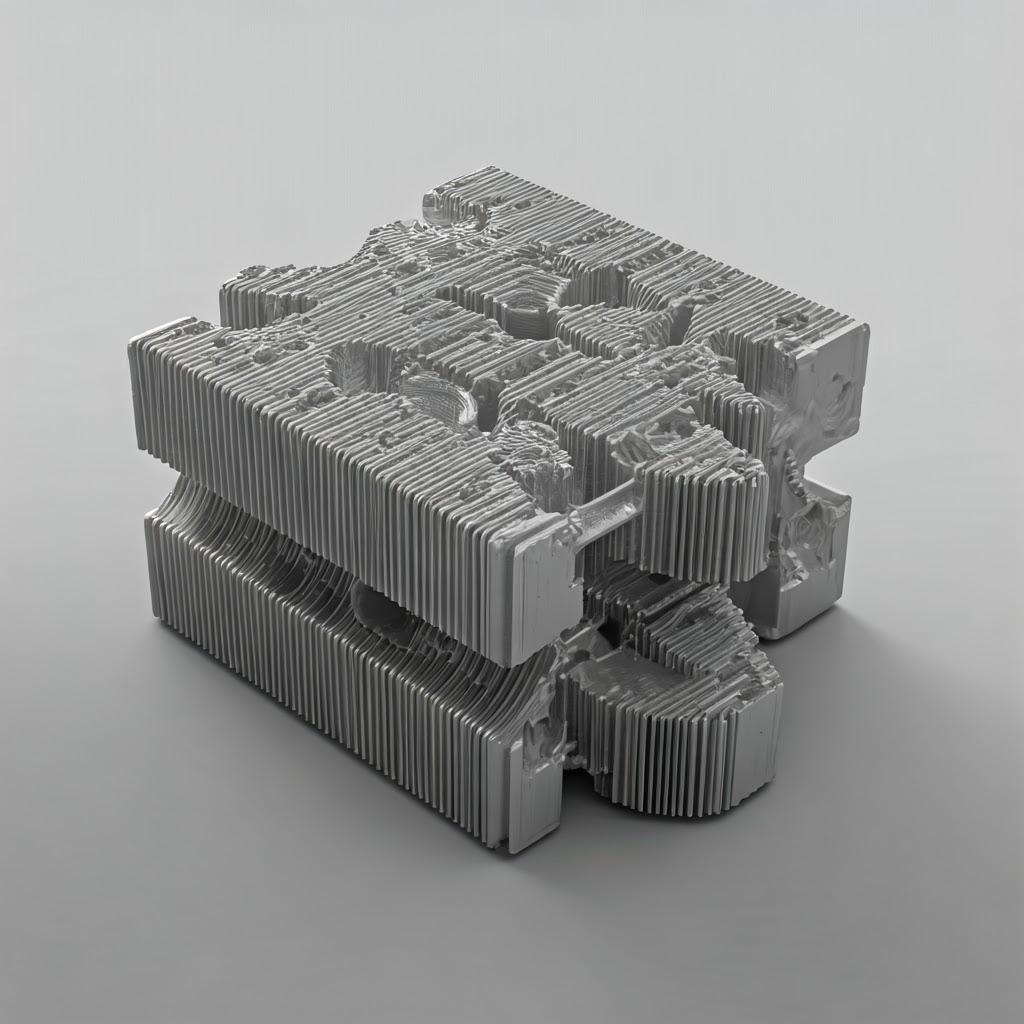
How to Choose the Right Metal 3D Printing Service Provider for Heat Sinks
Selecting the right manufacturing partner is as critical as the design and material choices when implementing 3D printed heat sinks. The unique demands of producing complex thermal management components using additive manufacturing require specific expertise and capabilities. For procurement managers and engineering teams embarking on metal AM supplier qualification, a thorough evaluation process is essential to ensure quality, reliability, and value. Choosing an inadequate supplier can lead to project delays, budget overruns, and substandard parts that fail to meet performance requirements.
Here are key criteria to evaluate when selecting a metal 3D printing service provider for electric motor heat sinks:
1. Technical Expertise & Engineering Support:
- Thermal Management Knowledge: Does the provider understand the principles of heat transfer, fluid dynamics, and the specific challenges of cooling electric motors? Can they offer DfAM advice tailored to thermal performance?
- Materials Science Depth: Do they have proven expertise with the specific alloys you need (CuCrZr, AlSi10Mg)? Do they understand the nuances of printing these materials and the required post-processing (especially heat treatments) to achieve optimal properties?
- DfAM Capability: Can they assist with or validate your design for additive manufacturing, including topology optimization, lattice generation, support strategy, and simulation (CFD/FEA)?
- Problem-Solving: Do they have a track record of troubleshooting complex AM builds and finding solutions?
2. Equipment, Technology & Capacity:
- Machine Portfolio: What types of metal AM machines do they operate (e.g., LPBF, EBM)? Are these machines suitable for the chosen material and part size? Do they have modern, well-maintained, and calibrated equipment? Met3dp, for example, emphasizes its industry-leading print volume, accuracy, and reliability.
- Objem sestavení: Can their machines accommodate the size of your heat sink?
- Capacity & Scalability: Do they have sufficient machine capacity to handle your prototyping needs and potentially scale to low or medium volume production? What is their typical backlog or queue time?
3. Material Capabilities & Quality Control:
- Powder Sourcing & Handling: Do they source high-quality powders from reputable suppliers, or like Met3dp, manufacture their own using advanced methods like technologie atomizace plynu a PREP? What are their procedures for powder quality control, handling, storage, and traceability?
- Materiálové znalosti: Can they demonstrate successful prints and documented material properties for CuCrZr and AlSi10Mg?
- Parameter Development: Do they have validated, optimized process parameters for the required materials?
4. Post-Processing Capabilities:
- Comprehensive Workflow: Can they manage the entire post-processing chain either in-house or through rigorously qualified partners? This includes:
- Stress Relief & Heat Treatment (Critically important: calibrated furnaces, controlled atmospheres, validated cycles for precipitation hardening/T6).
- Precise Part & Support Removal (Wire EDM, Bandsaw, Machining).
- CNC Machining (For critical tolerances and features).
- Surface Finishing (Blasting, Tumbling, Polishing, etc.).
- Cleaning & Inspection.
- Integrated Services: A provider offering a full suite of services simplifies the supply chain and ensures better process control.
5. Quality Management System (QMS) & Certifications:
- Formal QMS: Do they operate under a certified QMS like ISO 9001?
- Industry-Specific Certifications: Are they certified for relevant industries (e.g., AS9100 for aerospace, ISO 13485 for medical)? While not always required for industrial heat sinks, these certifications indicate a high level of process control and rigor.
- Documentation: Can they provide material certifications, certificates of conformity, inspection reports, and full traceability?
6. Experience & Track Record:
- Relevant Projects: Have they successfully produced parts similar in complexity, material, and application (thermal components, parts for automotive/aerospace/industrial sectors)? Can they provide case studies or references?
- Years in Business: How long have they specialized in metal AM?
7. Communication, Support & Project Management:
- Responsiveness: Are they quick to respond to inquiries and provide technical feedback?
- Clear Communication: Is communication professional, clear, and technically sound?
- Project Management: Do they have a structured approach to managing projects, providing updates, and handling potential issues?
8. Cost, Lead Time & Value:
- Transparent Quoting: Is their pricing clear and detailed, breaking down costs (material, machine time, labor, post-processing)?
- Konkurenční ceny: Is the pricing competitive relative to the market, considering the quality and services offered? Beware of quotes that seem too low, as they might compromise on quality or necessary post-processing.
- Reliable Lead Times: Can they provide realistic lead time estimates and demonstrate a history of meeting them?
- Overall Value: Consider the total value proposition, including expertise, quality, reliability, support, and risk mitigation, not just the unit price.
Evaluation Summary Table:
Kritéria | Klíčové otázky | Importance Level |
---|---|---|
Technické znalosti | Understand thermal/DfAM? Material science depth? Simulation capability? | Velmi vysoká |
Equipment & Technology | Suitable machines? Build volume? Calibration? Capacity/Scalability? | Vysoký |
Materiálové schopnosti | Proven expertise with CuCrZr/AlSi10Mg? Powder QC & handling? Parameter optimization? | Velmi vysoká |
Následné zpracování | In-house/partner capabilities for heat treat, machining, finishing, inspection? Integrated workflow? | Velmi vysoká |
Quality System/Certifications | ISO 9001? Industry certs (AS9100)? Traceability? Documentation? | Vysoký |
Experience & Track Record | Similar projects completed? Industry experience? Case studies/references? | Vysoký |
Communication & Support | Responsiveness? Clarity? Technical support? Project management? | Středně vysoké |
Cost, Lead Time & Value | Transparent pricing? Competitive? Reliable lead times? Overall value proposition? | Vysoký |
Export to Sheets
Výběr správného additive manufacturing partner is a strategic decision. Companies like Met3dp, offering comprehensive řešení aditivní výroby encompassing advanced printers, high-quality powders, and deep application expertise, represent the type of vertically integrated partner that can de-risk the adoption of AM for critical components like electric motor heat sinks. Conduct thorough due diligence, ask detailed questions, and potentially start with a pilot project to validate a supplier’s capabilities before committing to larger volume production.
Understanding Cost Factors and Lead Times for 3D Printed Heat Sinks
While metal 3D printing enables the creation of superior performance heat sinks, understanding the associated cost structure and typical lead times is essential for project planning, budgeting, and managing expectations. Unlike traditional manufacturing where tooling often dominates costs for low volumes, AM costs are more directly tied to material consumption, machine time, and labor-intensive post-processing.
Key Cost Drivers for Metal AM Heat Sinks:
- Náklady na materiál:
- Powder Price: The cost per kilogram of metal powder varies significantly by alloy. Copper alloys like CuCrZr are substantially more expensive than aluminum alloys like AlSi10Mg. Factor in market fluctuations.
- Part Volume: The actual volume of the final part directly determines the amount of powder consumed. Topology optimization and lattice structures play a key role here, potentially reducing material usage compared to solid designs.
- Support Structure Volume: Material used for support structures also contributes to cost. Efficient DfAM minimizes this.
- Powder Waste/Recycling: While powder can be recycled, there are losses and degradation over cycles, which factors into the effective material cost calculated by service providers.
- Machine Time:
- Doba výstavby: This is often the largest cost component. It depends on:
- Part Height (Z-axis): The primary driver, as printing is layer by layer.
- Part Volume/Density: Larger or denser parts require more material deposition per layer.
- Tloušťka vrstvy: Thinner layers improve resolution but significantly increase build time.
- Scan Strategy: Complex scanning patterns for managing stress or fine features can take longer.
- Number of Parts per Build: Nesting multiple parts efficiently on a build plate utilizes machine time better, reducing cost per part for bulk AM production.
- Machine Operating Costs: Amortization of expensive AM equipment, energy consumption (lasers/electron beams consume significant power), inert gas consumption, maintenance.
- Doba výstavby: This is often the largest cost component. It depends on:
- Náklady na pracovní sílu:
- Setup & Teardown: Preparing the build job, loading powder, removing the build plate, initial cleaning.
- Depowdering: Carefully removing loose powder from the part(s).
- Post-Processing Labor: This can be substantial:
- Manual support removal.
- Setup and operation for heat treatment cycles.
- Setup and operation for CNC machining.
- Manual finishing (polishing, deburring).
- Inspection and quality control tasks.
- Post-Processing Costs (Beyond Labor):
- Tepelné zpracování: Furnace time, energy consumption, inert gas cost. Specific cycles for CuCrZr or AlSi10Mg require precise control.
- Obrábění: CNC machine time, tooling costs, fixture design/fabrication if needed for complex shapes.
- Dokončovací práce: Cost of consumables (blasting media, polishing compounds), specialized equipment usage (e.g., electropolishing).
- HIP (if required): Hot Isostatic Pressing is an expensive process typically outsourced to specialized providers.
- Inspection Tools: Cost associated with using CMM, 3D scanners, NDT equipment.
- Design & Engineering:
- DfAM & Simulation: While potentially an upfront investment, optimizing the design using topology optimization, lattice structures, and simulation can significantly reduce material consumption and print time, lowering manufacturing costs.
- Složitost: Highly complex designs might require more sophisticated build planning and potentially more support, slightly increasing setup and post-processing effort. However, AM handles complexity far more cost-effectively than traditional methods.
- Quantity & Batch Size:
- Úspory z rozsahu: AM exhibits economies of scale, but differently than mass production methods. Setup costs are amortized over the batch. Full build plates are more cost-effective than partially filled ones. The cost per part generally decreases with volume, but the relationship is less steep than with methods involving hard tooling.
Cost Comparison Snapshot (Conceptual):
Vlastnosti | Metal AM (e.g., LPBF) | Traditional (e.g., CNC Machining from Billet) | Traditional (e.g., Die Casting) |
---|---|---|---|
Complexity Handling | Excellent (cost less sensitive to complexity) | Good (cost increases significantly with complexity) | Fair (limited by tooling possibilities) |
Náklady na nástroje | Žádný | None (but fixture costs may apply) | Velmi vysoká |
Cost per Part (Low Vol) | Mírná až vysoká | High (especially for complex parts) | Prohibitively High |
Cost per Part (High Vol) | Moderate (limited decrease) | Moderate (significant decrease from low vol) | Very Low (once tooling is amortized) |
Materiálový odpad | Nízký | High (subtractive) | Low (casting process) |
Sweet Spot | Complex geometries, Custom parts, Prototypes, Low-Med Vol. | Moderately complex parts, Medium-High Vol. | Simple to moderate shapes, Very High Vol. |
Export to Sheets
Lead Time Factors:
Lead time for a 3D printed heat sink is the sum of several stages:
- Quoting & Order Processing: 1-5 days (depending on complexity and provider responsiveness).
- Design Review & Build Prep: 1-3 days (DfAM checks, support strategy, nesting, slicing).
- Machine Queue Time: Highly variable (0 days to several weeks depending on provider backlog).
- Doba tisku: Hours to several days (depending on part height, volume, quantity).
- Cooling & Depowdering: 0.5-1 day.
- Následné zpracování: Highly variable:
- Stress Relief: ~1 day (including furnace time).
- Part/Support Removal: Hours to days (depending on complexity/method).
- Heat Treatment (Properties): 1-3 days (including furnace cycles).
- Machining: Days to weeks (depending on complexity and shop availability).
- Finishing: Hours to days.
- Inspection: Hours to days.
- Doprava: 1 day to 1 week+ (depending on location and method).
Typical Lead Time Ranges:
- Prototypes (Simple Post-Processing): 1-3 weeks common.
- Prototypes (Complex Post-Processing/Machining): 3-6 weeks+.
- Production Batches: Highly dependent on quantity and complexity, requires careful planning with the provider. Can range from weeks to months.
- Expedited AM Services: Many providers offer faster turnaround for a premium, often by prioritizing queue position and potentially optimizing build/post-processing steps where possible.
Understanding these cost drivers and lead time components allows for more accurate project planning and budgeting. Engage with potential metal AM suppliers early to get realistic quotes and timelines based on your specific heat sink design and requirements. Factor in the entire workflow, especially post-processing, as it significantly impacts both cost and delivery time in the supply chain AM.
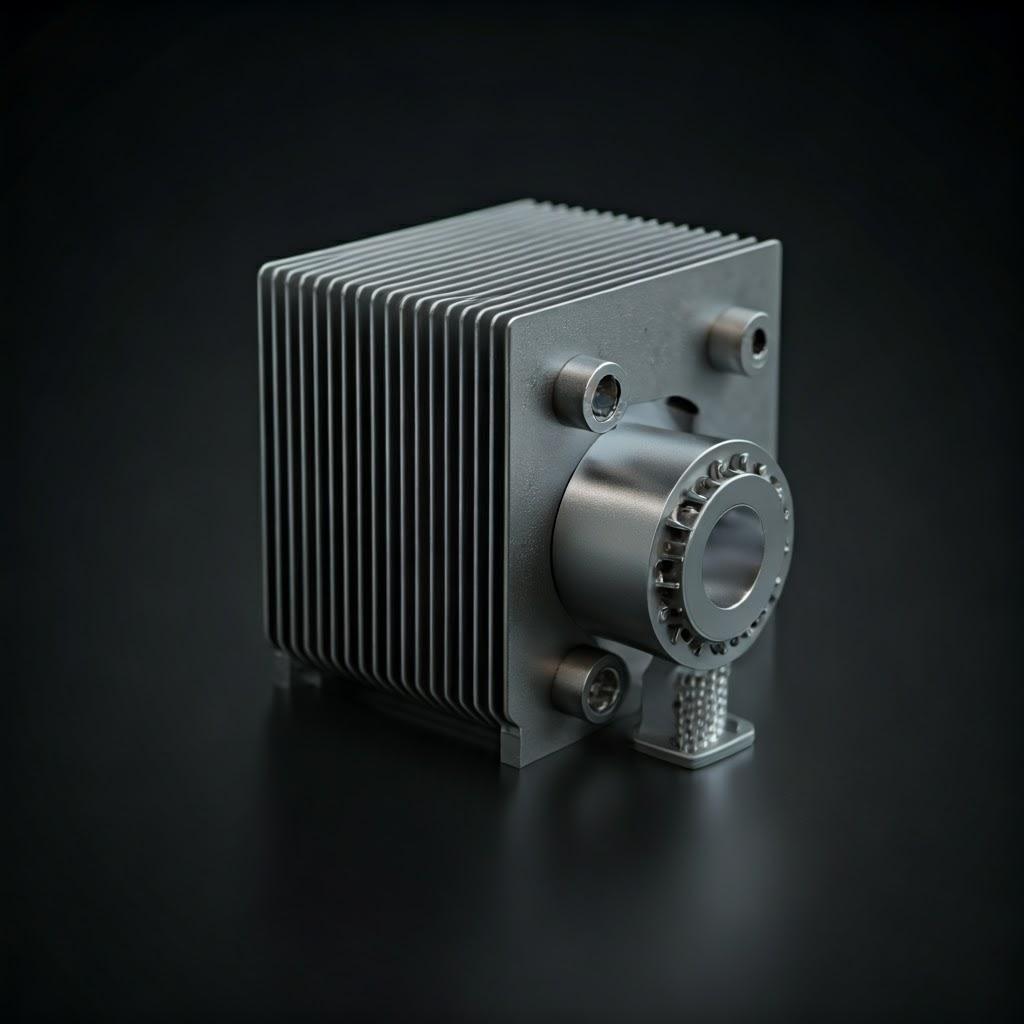
Frequently Asked Questions (FAQ) about 3D Printed Electric Motor Heat Sinks
Here are answers to some common questions engineers and procurement managers have about using metal additive manufacturing for electric motor heat sinks:
- How does the performance of 3D printed heat sinks compare to traditional ones (machined, cast, extruded)?
- Often significantly better, especially for complex designs. Metal AM allows for geometries impossible with traditional methods, such as topology-optimized shapes, internal lattice structures, and conformal cooling channels. These features can lead to:
- Higher Thermal Efficiency: Better heat dissipation for the same size/weight.
- Lower Weight: Achieving the same or better cooling with less material/mass.
- Improved Integration: Combining heat sink functions with mounting or structural features.
- The degree of improvement depends heavily on leveraging DfAM principles. A simple replication of a traditional design might offer little benefit, while a fully optimized AM design can provide substantial performance gains (e.g., 20-50% or more improvement in thermal resistance is often cited in case studies).
- Often significantly better, especially for complex designs. Metal AM allows for geometries impossible with traditional methods, such as topology-optimized shapes, internal lattice structures, and conformal cooling channels. These features can lead to:
- Are 3D printed metal heat sinks durable and reliable for demanding applications like automotive or aerospace?
- Yes, when designed, manufactured, and post-processed correctly.
- Vlastnosti materiálu: Metal AM parts made from alloys like AlSi10Mg or CuCrZr can achieve mechanical properties (strength, hardness, fatigue resistance) comparable to or sometimes exceeding those of cast parts, especially after appropriate heat treatments (e.g., T6 for AlSi10Mg, precipitation hardening for CuCrZr).
- Hustota: Properly controlled AM processes achieve near-full density (>99.5%, often >99.9%), minimizing porosity that could compromise integrity. HIP can be used for critical applications to eliminate remaining voids.
- Kontrola kvality: Reputable service providers implement rigorous quality control, including material testing, process monitoring, dimensional inspection (CMM, 3D scanning), and NDT (like CT scanning) to ensure parts meet specifications and are free from critical defects.
- Reliability depends on choosing the right material, optimizing the design for AM, ensuring high-quality printing, and performing necessary post-processing and validation.
- Yes, when designed, manufactured, and post-processed correctly.
- What is the typical cost difference between an AM heat sink and a comparable traditionally manufactured one?
- It depends heavily on složitost a objem.
- High Complexity / Low Volume: For highly complex geometries (e.g., topology optimized, internal channels, lattices) or custom one-offs/prototypes/low volumes, AM is often significantly cheaper because it avoids high tooling costs (casting) or extensive multi-axis machining setups and material waste (CNC).
- Simple Geometry / High Volume: For simple designs (like basic extruded or cast finned heat sinks) produced in large quantities, traditional methods are typically much cheaper due to lower per-part costs once tooling is amortized or due to high-speed machining/extrusion processes.
- The Crossover Point: There’s a “crossover” volume/complexity point where the costs become comparable. Identifying this requires specific quoting based on the part design and quantity. AM’s value often lies in performance gains and lightweighting that justify a potentially higher unit cost.
- It depends heavily on složitost a objem.
- Can internal channels or complex lattice structures in AM heat sinks be effectively cleaned and cleared of powder?
- Yes, but it requires careful design and process control.
- Depowdering: Effective powder removal immediately after printing using optimized orientation, vibration, compressed air, and sometimes specialized equipment is crucial.
- Design for Depowdering: Designing internal channels with sufficient diameters, smooth paths, and potentially access ports aids powder removal. Avoid “powder traps” – enclosed voids with no escape path.
- Inspection: Techniques like airflow testing, flushing, or even CT scanning can be used to verify internal channels are clear.
- Materiál: Powder flowability (influenced by sphericity and particle size distribution, a focus for powder producers like Met3dp) impacts the ease of depowdering.
- Yes, but it requires careful design and process control.
- What information does a metal AM service provider need to provide an accurate quote for a 3D printed heat sink?
- To get the most accurate quote, provide as much detail as possible:
- 3D model CAD: STEP or STL format (STEP is often preferred as it contains more data).
- Material Specification: Clearly state the desired alloy (e.g., CuCrZr, AlSi10Mg) and any required material standards.
- Technical Drawing (Recommended): Include critical dimensions, GD&T tolerances, surface finish requirements (Ra values for specific surfaces), and any specific inspection notes.
- Množství: Number of parts required (prototype vs. production volumes).
- Požadavky na následné zpracování: Specify required heat treatments (stress relief, T6, precipitation hardening), machining needs (highlighting critical features), surface finishes, and any special cleaning or coating requirements.
- Application Context: Briefly describe the application (e.g., cooling an EV motor, aerospace actuator) and key performance requirements (thermal load, operating temperature, structural loads) if possible, as it helps the provider understand the context and criticality.
- Required Certifications/Documentation: Specify any needed material certs, CofCs, or inspection reports.
- To get the most accurate quote, provide as much detail as possible:
Providing comprehensive information upfront enables the metal AM supplier to accurately assess the manufacturing feasibility, estimate costs for all process steps, and provide a reliable lead time.
Conclusion: The Future of High-Performance Electric Motor Cooling is Additive
The relentless drive for higher power density, increased efficiency, and reduced weight in electric motors across industries like automotive, aerospace, and industrial automation necessitates a paradigm shift in thermal management. Traditional cooling solutions are increasingly hitting performance barriers imposed by manufacturing limitations. Metal additive manufacturing emerges as a powerful enabler, offering unprecedented design freedom to create highly optimized, lightweight, and complex heat sinks that were previously unimaginable.
By leveraging DfAM principles like topology optimization, conformal cooling channels, and intricate lattice structures, engineers can design 3D printed heat sinks using high-performance materials like CuCrZr and AlSi10Mg that deliver superior thermal performance tailored precisely to the motor’s needs. The ability to consolidate parts, rapidly prototype, and achieve significant lightweighting further solidifies AM’s advantage for demanding applications. While challenges exist, understanding material behavior, process nuances, essential post-processing steps, and robust quality control allows these hurdles to be overcome.
Choosing the right manufacturing partner – one with deep technical expertise, advanced equipment, rigorous quality systems, and proven experience with relevant materials and applications – is paramount to successfully implementing this technology. Factors like cost and lead time require careful consideration, balancing the performance benefits against project budgets and timelines.
The journey into additive manufacturing for critical components like heat sinks represents more than just adopting a new production technique; it’s about embracing a new way of thinking about design and performance. As the technology continues to mature and materials science advances, metal 3D printing is poised to become an indispensable tool in the development of next-generation electric motors, enabling cooler operation, longer life, and enhanced capabilities. Companies seeking a competitive edge in advanced thermal management must explore the potential of AM. Partnering with a leader in the field can accelerate this journey.
Met3dp provides comprehensive Met3dp additive solutions, spanning SEBM printers, advanced metal powders manufactured using industry-leading techniques, and application development services. With decades of collective expertise, Met3dp delivers cutting-edge systems and materials to enable next-generation manufacturing. Contact Met3dp to explore how its capabilities can power your organization’s additive manufacturing goals and revolutionize your approach to electric motor cooling.
Sdílet na
Facebook
Cvrlikání
LinkedIn
WhatsApp
E-mailem
MET3DP Technology Co., LTD je předním poskytovatelem řešení aditivní výroby se sídlem v Qingdao v Číně. Naše společnost se specializuje na zařízení pro 3D tisk a vysoce výkonné kovové prášky pro průmyslové aplikace.
Dotaz k získání nejlepší ceny a přizpůsobeného řešení pro vaše podnikání!
Související články
O Met3DP
Nedávná aktualizace
Náš produkt
KONTAKTUJTE NÁS
Nějaké otázky? Pošlete nám zprávu hned teď! Po obdržení vaší zprávy obsloužíme vaši žádost s celým týmem.
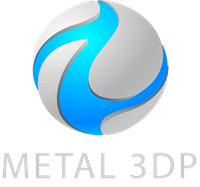
Kovové prášky pro 3D tisk a aditivní výrobu
SPOLEČNOST
PRODUKT
kontaktní informace
- Město Qingdao, Shandong, Čína
- [email protected]
- [email protected]
- +86 19116340731