Conformal Cooling Channels via Metal AM
Obsah
Introduction: Revolutionizing Aerospace Thermal Management with Metal AM
The aerospace industry operates at the extremes of performance, demanding components that are not only lightweight and strong but also capable of withstanding intense temperatures and pressures. Effective thermal management is paramount, directly impacting the efficiency, reliability, and lifespan of critical systems, from propulsion units to sensitive avionics. Traditional manufacturing methods, while mature, often impose significant limitations on the design of cooling features, particularly internal channels. This is where Metal Additive Manufacturing (Metal AM), also known as metal 3D tisk, emerges as a transformative technology, enabling the creation of highly complex Conformal Cooling Channels that were previously impossible or prohibitively expensive to produce.
Conformal cooling channels are intricate networks of internal passages designed to follow the contours of a part’s surface precisely. Unlike conventional cooling channels, which are often restricted to straight-line drills, conformal channels can snake around corners, vary in cross-section, and position themselves optimally close to heat sources. This intimate contact allows for significantly more efficient and uniform heat extraction, drastically improving thermal management performance.
Why does this matter so profoundly in aerospace?
- Vylepšený výkon: By managing heat more effectively, components like turbine blades can operate at higher temperatures, leading to increased engine efficiency and thrust. Cooled electronics maintain stable operating conditions, ensuring reliability.
- Snížení hmotnosti: Optimized cooling allows for more compact component designs. Furthermore, AM enables the integration of cooling features directly into structural components, eliminating the need for separate, bulky cooling assemblies and contributing to overall aircraft weight savings – a critical factor in aerospace economics.
- Increased Component Life: Uniform cooling minimizes thermal stress concentrations and hot spots, reducing wear and tear and extending the operational lifespan of expensive, mission-critical parts.
- Svoboda designu: Metal AM unshackles engineers from the constraints of traditional manufacturing. It allows for the creation of organic, complex geometries optimized purely for thermal performance, leading to innovative solutions for previously intractable heat challenges.
The ability to manufacture these advanced cooling geometries relies heavily on processes like Selective Laser Melting (SLM), Electron Beam Melting (EBM), and Direct Metal Laser Sintering (DMLS). These layer-by-layer techniques use high-energy sources (lasers or electron beams) to selectively fuse fine metal powders, building parts directly from digital models. This precise control enables the intricate internal pathways characteristic of conformal cooling.
For procurement managers and engineers in aerospace, understanding the potential of metal AM for conformal cooling is no longer optional; it’s essential for staying competitive. It represents a paradigm shift in how thermal challenges are addressed, moving from compromised, manufacturability-driven designs to highly optimized, performance-driven solutions. This technology empowers the development of next-generation aircraft and spacecraft with unprecedented capabilities. Firmy hledající dodavatelé leteckých komponentů specializing in advanced manufacturing techniques are increasingly turning to poskytovatelé služeb v oblasti AM kovů capable of delivering these complex parts with the required precision and material properties. As a leading provider of comprehensive 3D tisk z kovu solutions, including advanced equipment and high-performance powders, Met3dp is at forefront of this revolution, empowering aerospace innovation.
The integration of metal AM is not merely an alternative manufacturing route; it’s an enabler of superior design and functionality. It allows for the consolidation of multiple parts into a single, complex component with integrated cooling, reducing assembly time, potential leak paths, and overall system complexity. This introduction sets the stage for exploring the specific applications, advantages, materials, and considerations involved in leveraging metal AM for producing high-performance conformal cooling channels in the demanding aerospace sector. We will delve into how this technology addresses specific pain points, the materials best suited for the task, and how to navigate the design and production process successfully. The focus remains on providing aerospace engineering solutions that push the boundaries of thermal performance through advanced additive manufacturing aerospace techniky.
Critical Applications: Where Conformal Cooling Excels in Aerospace
The benefits of conformal cooling channels realized through metal additive manufacturing are not theoretical; they are being actively applied across a spectrum of critical aerospace systems where efficient thermal management is non-negotiable. The ability to place cooling exactly where it’s needed, following complex part contours, unlocks performance gains and design possibilities previously unattainable. Let’s explore some key application areas:
1. Gas Turbine Engines (Jet Engines & Power Generation Turbines):
- Lopatky a lopatky turbíny: These components operate in arguably the harshest environment within an aircraft, exposed to extremely high temperatures (often exceeding the melting point of the alloys used) and mechanical stresses. Conformal cooling channels, intricately woven within the blade’s internal structure and following the complex airfoil shape, allow for more effective cooling using less bleed air diverted from the engine’s compressor.
- Dopad: Higher Turbine Inlet Temperatures (TIT) can be achieved, directly boosting engine thrust and fuel efficiency. Uniform cooling reduces thermal gradients, minimizing creep and thermal fatigue, thereby extending blade life and improving engine reliability. Metal AM enables complex internal geometries like turbulators and pin-fin arrays within the channels for enhanced heat transfer. Materials like IN625 (Inconel 625) are frequently used due to their exceptional high-temperature strength and corrosion resistance.
- Combustion Chambers: Liners and components within the combustor face intense heat from fuel burning. Conformal cooling integrated into these structures helps maintain structural integrity, prevent buckling, and ensure complete combustion, reducing harmful emissions.
- Nozzles: Exhaust nozzles, particularly complex variable geometry nozzles, benefit from integrated conformal cooling to manage the extreme temperatures of the exhaust gases while optimizing aerodynamic performance.
2. Avionics and Electronics Cooling:
- Integrated Cold Plates & Heat Sinks: Modern aircraft are packed with powerful electronics for navigation, communication, control, and mission systems. These generate significant heat in often tightly packed spaces. Metal AM allows for the creation of highly customized cold plates and heat sinks with conformal channels that precisely match the layout of heat-generating components (CPUs, FPGAs, power amplifiers).
- Dopad: More efficient heat dissipation ensures electronics operate within safe temperature limits, preventing failures and ensuring system reliability. The design freedom of AM enables lightweight, compact cooling solutions integrated directly into electronic enclosures, saving valuable space and weight. Materials like AlSi10Mg (Aluminum Silicon Magnesium alloy) are ideal due to their excellent thermal conductivity and low density. Wholesale metal powder suppliers catering to the aerospace electronics sector see growing demand for these lightweight alloys.
- Power Electronics Modules: Cooling for high-power components like inverters and converters in electric or hybrid-electric propulsion systems is critical. Conformal cooling ensures effective heat removal, enabling higher power densities.
3. Environmental Control Systems (ECS):
- Výměníky tepla: Aircraft ECS manage cabin air temperature, pressure, and quality. This involves complex heat exchangers operating with air and refrigerant cycles. Metal AM enables the design of highly compact, efficient heat exchangers with intricate fin structures and conformal flow paths optimized for maximum heat transfer within minimal volume and weight constraints.
- Dopad: Lighter, smaller ECS components contribute to overall aircraft efficiency. Improved heat exchanger performance enhances passenger comfort and system responsiveness. Part consolidation through AM can reduce the number of joints and potential leak points compared to traditionally brazed or welded assemblies.
4. Rocket Engines and Spacecraft Thermal Control:
- Combustion Chambers & Nozzles: Similar to jet engines but operating at even more extreme temperatures and pressures, rocket engine components rely heavily on advanced cooling. Regenerative cooling, where cryogenic fuel is passed through channels in the chamber and nozzle walls, is common. Metal AM allows for highly optimized regenerative cooling channel geometries, maximizing heat absorption and engine performance (specific impulse).
- Satellite Thermal Control: Maintaining stable temperatures for sensitive instruments and electronics in the vacuum of space, with extreme temperature swings between sun exposure and shadow, is vital. AM enables complex radiator panels and heat pipes with integrated, conformal fluid loops.
Target Industries and Functions:
The primary beneficiaries are clearly within the aerospace and defense manufacturing sectors. However, the principles and technologies extend to related high-performance industries:
- Motorsport: Formula 1 and other racing series use AM for conformal cooling in engine components, brakes, and gearboxes for performance gains.
- High-Performance Industrial Equipment: Gas turbines for power generation, specialized tooling requiring cooling (e.g., injection molds – although typically using different materials), and high-power laser systems can all benefit.
The core function enabled by AM conformal cooling channels is precision thermal management – removing heat efficiently and uniformly from critical locations within complex, high-performance components. This directly translates to improved efficiency, enhanced reliability, extended component life, and often, significant weight reduction, making it a cornerstone technology for aerospace manufacturing services focused on next-generation systems. Procurement managers seeking industrial 3D printing partners need providers with proven experience in these demanding applications.
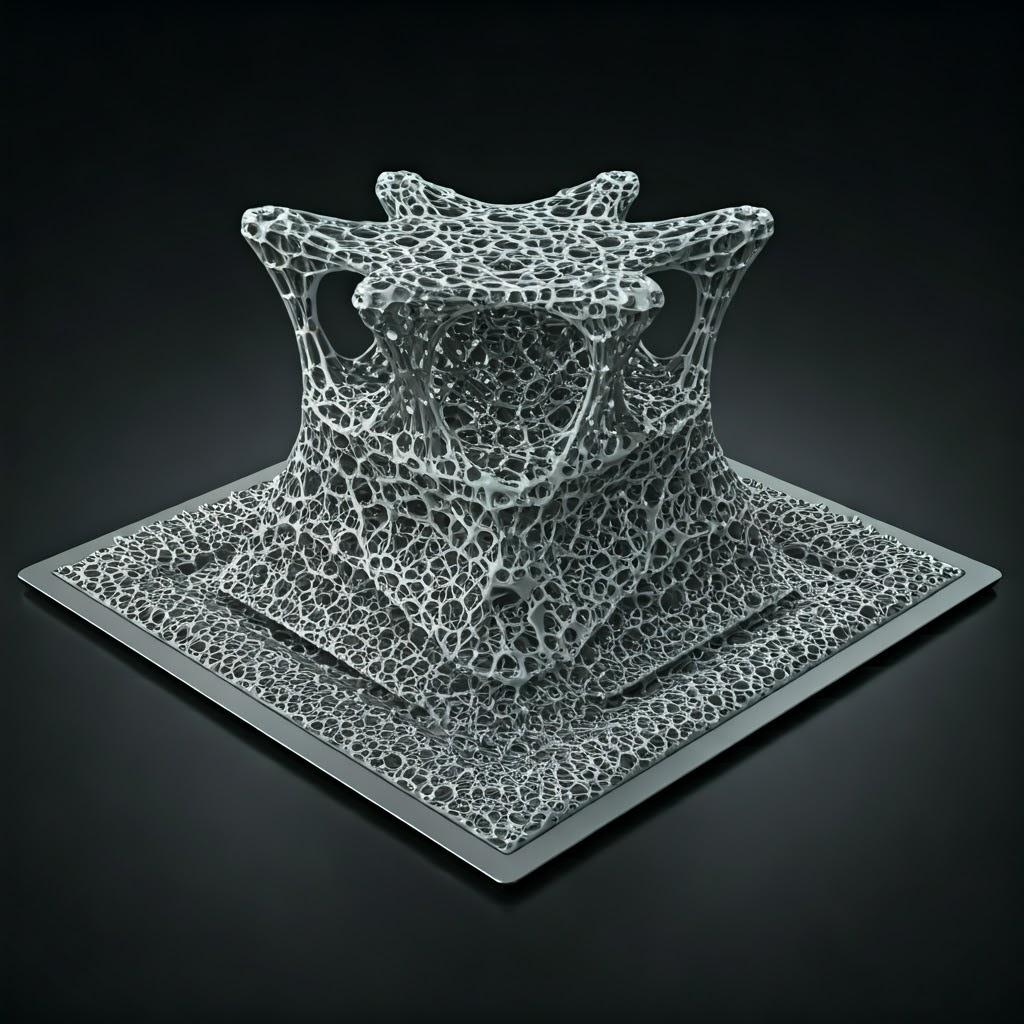
The Additive Advantage: Why Metal 3D Printing for Complex Cooling Geometries?
While traditional manufacturing methods like casting, machining (drilling), and brazing have served the aerospace industry for decades, they inherently limit the complexity and efficiency of cooling channel designs. Metal Additive Manufacturing fundamentally overcomes these limitations, offering a suite of advantages that are particularly compelling for creating conformal cooling channels. Engineers and procurement metal AM specialists recognize these benefits as drivers for adopting the technology:
1. Unparalleled Design Freedom & Geometric Complexity:
- Traditional: Limited to linear drill paths, simple bends (requiring multiple setups or complex tooling), or assembling multiple machined pieces (introducing joints and potential failure points). Achieving true conformity to curved surfaces is extremely difficult and costly.
- Metal AM: Builds parts layer-by-layer directly from a 3D CAD model. This allows for:
- True Conformal Paths: Channels can follow the exact contours of complex surfaces (e.g., airfoil shapes, combustion chamber walls, electronic component layouts).
- Variable Cross-Sections: Channel diameter and shape can be varied along the path to optimize flow rate and heat transfer locally.
- Komplexní vnitřní vybavení: Features like trip strips, turbulators, pin fins, and complex manifolds can be integrated directly inside the channels during the build process to enhance turbulence and heat exchange, without requiring secondary assembly.
- Organic Shapes: Designs can be optimized using topology optimization and generative design algorithms, resulting in lightweight, high-performance structures impossible to make otherwise.
2. Part Consolidation & Reduced Assembly:
- Traditional: Complex cooling systems often require manufacturing multiple individual components (e.g., plates, tubes, manifolds) and then joining them through welding, brazing, or fasteners. Each joint represents a potential leak path, added weight, and an additional manufacturing/inspection step.
- Metal AM: Enables the printing of a single, monolithic component with fully integrated internal cooling channels.
- Výhody: Eliminates joints, drastically reducing potential leak paths and improving overall system reliability. Reduces part count, simplifying supply chains and assembly processes. Often leads to lighter final components as connecting flanges and fasteners are eliminated. This is a key value proposition for contract manufacturing aerospace providers using AM.
3. Enhanced Thermal Performance & Efficiency:
- Traditional: Straight-line channels often result in suboptimal distances from the heat source and uneven cooling, leading to hot spots and thermal gradients. Limited internal features restrict heat transfer enhancement.
- Metal AM: Conformal channels minimize the distance between the coolant and the heat source across the entire critical surface area.
- Výhody: More efficient heat extraction leads to lower component temperatures and/or allows systems to operate at higher power/temperature levels. Uniform cooling minimizes thermal stresses, extending component life. Integrated turbulence-enhancing features maximize the heat transfer coefficient between the coolant and the channel wall.
4. Significant Weight Reduction:
- Traditional: Design compromises for manufacturability often lead to thicker walls or bulkier cooling assemblies than strictly necessary for thermal performance.
- Metal AM: Design freedom allows for material placement only where structurally or thermally needed. Topology optimization can further strip away unnecessary mass. Part consolidation inherently reduces weight.
- Výhody: Critical for aerospace, where every kilogram saved translates to fuel efficiency or increased payload capacity. This aligns perfectly with the goals of lightweight metal components development.
5. Accelerated Prototyping & Design Iteration:
- Traditional: Creating prototypes with complex internal cooling features often involves expensive tooling (casting molds) or laborious multi-stage machining and assembly processes. Design iterations are slow and costly.
- Metal AM: Prototypes can be printed directly from CAD data relatively quickly, without the need for tooling.
- Výhody: Allows engineers to rapidly test and validate different cooling channel designs, perform functional testing, and iterate towards an optimal solution much faster and more cost-effectively. Shortens the development cycle for new aerospace components.
6. Material Variety & Optimization:
- Metal AM: Supports a growing range of aerospace-grade alloys, including high-temperature superalloys (like IN625), lightweight aluminum alloys (like AlSi 10Mg), titanium alloys, and copper alloys, allowing selection of the optimal material for the specific thermal and structural requirements. Dodavatelé kovových prášků like Met3dp offer a portfolio optimized for AM processes.
- Traditional: Material choice might be constrained by the manufacturing process itself (e.g., castability, machinability).
Comparative Summary:
Vlastnosti | Traditional Manufacturing (Drilling/Brazing) | Výroba aditiv kovů (AM) | Advantage of AM |
---|---|---|---|
Geometric Complexity | Low (Straight lines, simple bends) | High (Conformal paths, variable sections, internal features) | Optimal thermal design, design freedom |
Conformity | Poor / Difficult | Vynikající | Uniform & efficient cooling |
Konsolidace částí | Low (Multiple parts, assembly needed) | High (Single monolithic part possible) | Reduced leaks, weight, assembly time |
Tepelný výkon | Moderate / Compromised | High / Optimized | Higher operating temps, longer life, efficiency |
Hmotnost | Often Higher | Potentially Significantly Lower | Fuel savings, increased payload |
Prototyping Speed | Slow / Costly | Fast / Relatively Lower Cost (for complex parts) | Faster development cycles, rapid iteration |
Tooling Requirement | High (Molds, Jigs, Fixtures) | Low / None | Cost savings, flexibility |
Interní funkce | Very Limited / Post-machined | High (Integrated during build) | Enhanced heat transfer |
Export do archů
In essence, metal AM shifts the paradigm from “designing for manufacturability” to “manufacturing the optimal design.” For aerospace conformal cooling channels, where performance and reliability are paramount, the advantages offered by additive manufacturing provide a compelling case for its adoption, driving innovation in aerospace thermal management solutions.
Material Matters: Selecting Optimal Powders for Aerospace Cooling
The performance, reliability, and manufacturability of additively manufactured conformal cooling channels are critically dependent on the choice of metal powder. The material must not only withstand the demanding operating conditions of aerospace environments but also be amenable to the specific metal AM process being used (e.g., SLM, EBM). For aerospace cooling applications, two materials frequently stand out: AlSi 10Mg a IN625 (Inconel 625). The selection hinges on a trade-off between thermal properties, temperature resistance, weight, and cost.
High-Quality Powders: The Foundation of Reliable AM Parts
Before diving into specific materials, it’s crucial to emphasize the importance of powder quality. Consistent particle size distribution (PSD), high sphericity, good flowability, low satellite content, and controlled chemical composition are essential for achieving dense, defect-free parts with predictable mechanical and thermal properties. Inferior powder can lead to issues like porosity, poor surface finish, inconsistent layer fusion, and ultimately, component failure.
This is where specialized metal powder suppliers play a vital role. Companies like Met3dp, utilizing advanced powder production techniques such as Vacuum Induction Melting Gas Atomization (VIGA) and Plasma Rotating Electrode Process (PREP), focus on producing high-purity, highly spherical metal powders specifically optimized for additive manufacturing processes. Their rigorous quality control ensures lot-to-lot consistency, which is critical for certified aerospace component production. Met3dp’s commitment extends beyond standard alloys; they possess expertise in developing and manufacturing innovative materials like TiNi, TiTa, TiAl, TiNbZr, and various superalloys, tailored for demanding applications. Partnering with a provider like Met3dp ensures access to vysoce kvalitní kovové prášky that form the bedrock of reliable AM components.
1. AlSi10Mg: The Lightweight Thermal Performer
- Popis: AlSi10Mg is an aluminum alloy containing silicon and magnesium. It’s a well-established casting alloy also widely adapted and optimized for metal AM processes like SLM.
- Key Properties & Benefits for Cooling Channels:
- Vynikající tepelná vodivost: Aluminum alloys generally offer very good thermal conductivity (around 120-150 W/m·K for AM AlSi10Mg, depending on post-processing), enabling efficient heat transfer from the component into the coolant.
- Nízká hustota: With a density of approximately 2.67 g/cm³, it’s significantly lighter than steels or nickel alloys, contributing directly to weight savings – a primary driver in aerospace. This makes it ideal for lightweight metal components.
- Dobrý poměr pevnosti a hmotnosti: While not as strong as steel or superalloys, especially at elevated temperatures, it offers a respectable strength-to-weight ratio, suitable for many structural components incorporating cooling.
- Dobrá odolnost proti korozi: Exhibits good resistance to atmospheric corrosion.
- Vynikající tisknutelnost: Generally considered one of the easier aluminum alloys to process via SLM, allowing for fine features and complex geometries.
- Omezení:
- Omezený výkon při vysokých teplotách: Mechanical properties (strength, creep resistance) degrade significantly at temperatures above ~150-200°C. This restricts its use in components exposed to very high temperatures, like hot sections of turbine engines.
- Lower Ductility compared to wrought alloys.
- Typical Aerospace Cooling Applications: Avionics enclosures, electronics cold plates, heat sinks, ECS heat exchangers, structural brackets with integrated cooling, drone components. Ideal where weight saving is critical and operating temperatures are moderate.
2. IN625 (Inconel 625): The High-Temperature Champion
- Popis: IN625 is a nickel-chromium-molybdenum-niobium superalloy renowned for its outstanding high-temperature strength, corrosion resistance, and fatigue life.
- Key Properties & Benefits for Cooling Channels:
- Excellent High-Temperature Strength & Creep Resistance: Maintains significant strength and resists deformation (creep) at temperatures up to 800-900°C, and retains useful properties even higher for short durations. This is crucial for components in engine hot sections.
- Outstanding Corrosion and Oxidation Resistance: Resists a wide range of corrosive environments, including high-temperature oxidation, making it suitable for harsh combustion and exhaust environments.
- High Fatigue Strength: Critical for components subjected to cyclic thermal and mechanical loads.
- Good Weldability/Printability: While more challenging to print than AlSi10Mg (requiring careful parameter control to manage residual stresses), IN625 is one of the more processable nickel superalloys via AM.
- Omezení:
- Lower Thermal Conductivity: Compared to aluminum alloys, IN625 has significantly lower thermal conductivity (around 10-12 W/m·K). This means cooling channels might need to be designed differently (e.g., larger surface area, enhanced turbulence features) to achieve the same level of heat removal.
- Vysoká hustota: With a density of approximately 8.44 g/cm³, it is substantially heavier than aluminum alloys.
- Vyšší cena materiálu: Nickel superalloys are inherently more expensive than aluminum alloys.
- Typical Aerospace Cooling Applications: Turbine blades and vanes (internal cooling passages), combustion chamber liners, exhaust system components, nozzle flaps, heat shields – applications demanding exceptional performance at extreme temperatures. Often specified by dodavatelé leteckých komponentů for propulsion systems.
Úvahy o výběru materiálu:
The choice between AlSi10Mg and IN625 (or other potential alloys like Titanium alloys, e.g., Ti6Al4V) involves a careful analysis of the specific application requirements:
Faktor | AlSi 10Mg | IN625 (Inconel 625) | Key Consideration |
---|---|---|---|
Max Operating Temp. | Low-Moderate (~150-200°C max) | Very High (~800-900°C+) | Dominant factor for material selection. |
Tepelná vodivost | Vysoký | Nízký | Impacts channel design for heat transfer efficiency. |
Density / Weight | Nízký | Vysoký | Critical for weight-sensitive applications. |
Síla | Moderate (Good Strength/Weight) | Very High (Especially at Temp.) | Structural integrity under load and temperature. |
Odolnost proti korozi | Dobrý | Vynikající | Required for harsh chemical/oxidizing environments. |
Náklady | Dolní | Vyšší | Budget constraints vs. performance needs. |
Možnost tisku | Generally Easier | More Challenging (Stress Management) | Process complexity and achievable feature detail. |
Export do archů
Why Met3dp’s Powders Matter Here:
Met3dp’s focus on producing vysoce kvalitní kovové prášky using advanced atomization techniques (Gas Atomization, PREP) ensures that whether an engineer selects AlSi10Mg for a lightweight avionics cooler or IN625 for a demanding turbine blade, the starting material meets the stringent requirements for aerospace applications. Their capabilities extend to inovativní slitiny, offering potential for future advancements in thermal management materials. Consistent sphericity and flowability, as emphasized by Met3dp, translate directly to more reliable printing processes and denser end components, crucial for the integrity of internal cooling channels where defects can compromise performance or lead to catastrophic failure. Partnering with a knowledgeable provider like Met3dp, offering both state-of-the-art printing equipment and premium powders, provides a robust foundation for leveraging the full potential of metal AM for aerospace cooling.
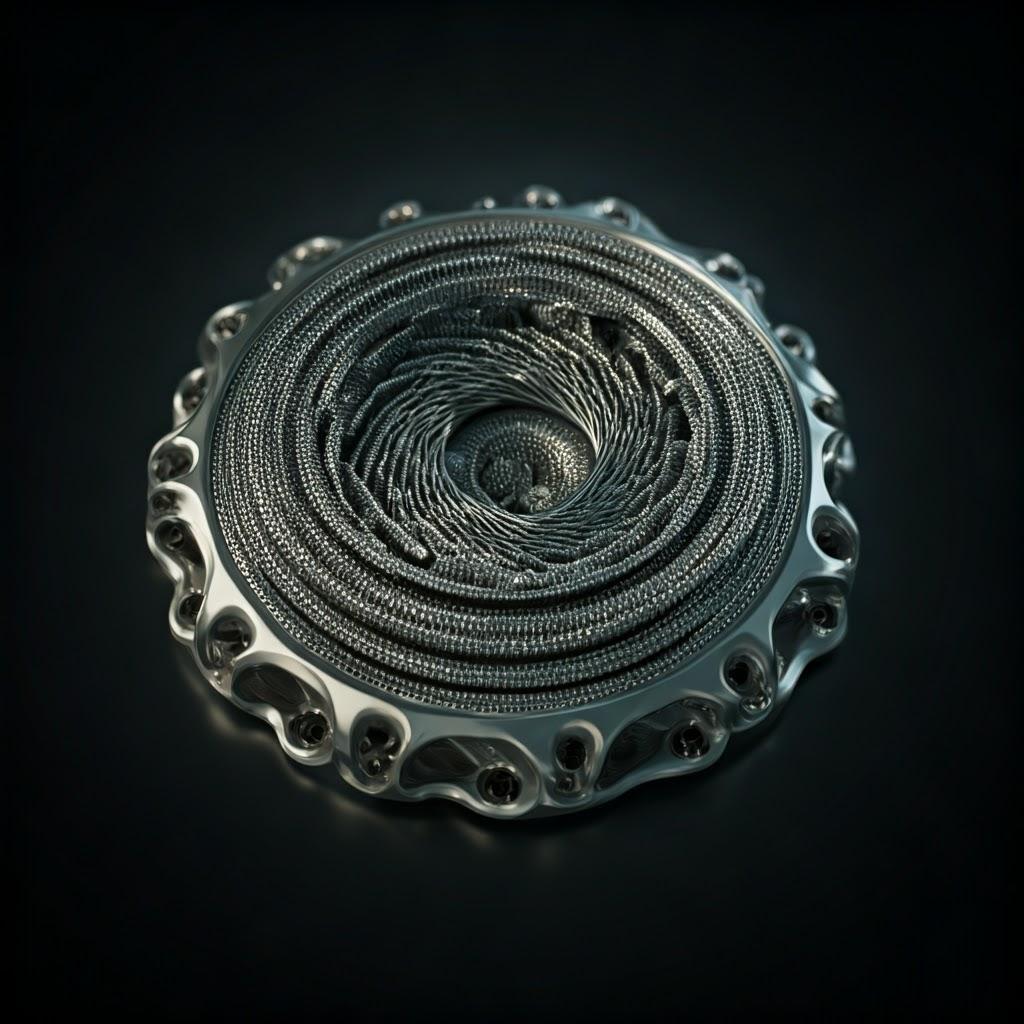
Design for Additive Manufacturing (DfAM): Optimizing Channels for Printability and Performance
Simply replicating a design intended for traditional manufacturing using Metal Additive Manufacturing (AM) rarely unlocks the full potential of the technology, especially when dealing with complex internal geometries like conformal cooling channels. Design pro aditivní výrobu (DfAM) is a crucial methodology that involves tailoring the component’s design to leverage AM’s strengths while accounting for its specific constraints and nuances. Applying DfAM principles from the outset is essential for achieving functional, reliable, and cost-effective aerospace cooling components. Engineers working with metal AM design rules must consider several key factors:
1. Channel Path Planning and Geometry:
- Flow Optimization: The primary goal is efficient cooling. Computational Fluid Dynamics (CFD) analysis should be integral to the design process. CFD simulates fluid flow and heat transfer within the proposed channel geometry, allowing engineers to:
- Identify potential stagnation zones or areas of poor flow.
- Optimize channel cross-sections (circular, elliptical, rectangular) and dimensions along the path to maintain desired flow rates and maximize heat transfer where needed most.
- Validate the effectiveness of integrated turbulence-enhancing features.
- Minimum Radii and Smooth Transitions: While AM allows for complex curves, overly sharp bends can increase pressure drop, create stagnant zones, and potentially be harder to print reliably or clear of powder. Aim for smooth, gradual transitions and adhere to minimum bend radii recommended for the specific AM process and material.
- Conformity: Ensure the channel path truly follows the contours of the heat source area as closely as possible, maintaining a consistent distance for uniform heat extraction. DfAM enables this precise placement.
2. Self-Supporting Angles and Support Structures:
- Overhangs and Angles: Metal AM processes like SLM build layer by layer. Steep overhangs or horizontal features require support structures underneath them during the build process to prevent collapse or distortion. Internal channels inherently create overhangs (the “roof” of the channel).
- Self-Supporting Geometries: Designing channels with cross-sections that minimize the need for internal supports is highly desirable. Angles typically greater than 45 degrees from the horizontal can often be self-supporting (depending on the material, machine, and parameters). Teardrop or diamond shapes are sometimes used for horizontal channel sections instead of purely circular ones to create self-supporting geometries.
- Support Strategy (If Unavoidable): If internal supports are necessary within channels, they must be designed for effective removal during post-processing. This adds complexity and cost. DfAM focuses on minimizing or eliminating this need through clever orientation and geometric choices. External supports holding the part to the build plate are standard and removed later.
3. Wall Thickness and Minimum Feature Size:
- Minimum Wall Thickness: There’s a limit to how thin walls can be reliably printed. This depends on the AM machine’s resolution, laser/beam spot size, powder characteristics, and material. For cooling channels, the wall separating the coolant from the hot component material must be thick enough for structural integrity and leak prevention but thin enough to minimize thermal resistance. Typical minimum printable wall thicknesses might range from 0.3mm to 0.8mm, but design guidelines often recommend slightly thicker walls for robustness, especially in critical aerospace applications.
- Minimum Channel Diameter: Similarly, there’s a minimum diameter for channels that can be reliably printed and, crucially, cleared of unfused powder after the build. This often dictates the lower limit for channel sizing, typically around 0.5mm to 1.0mm, though specific capabilities should be confirmed with the metal 3D printing service bureau.
- Aspect Ratio: Very tall, thin walls can be prone to warping or instability during printing. DfAM considers feature aspect ratios to ensure manufacturability.
4. Integrating Flow Enhancement Features:
- Turbulators: AM allows the direct integration of internal features like ribs, bumps, spirals (trip strips), or pin-fin arrays within the cooling channels. These features disrupt the laminar flow boundary layer, induce turbulence, and significantly increase the convective heat transfer coefficient between the coolant and the channel wall.
- Složitost návrhu: Designing these features requires careful CFD analysis to ensure they enhance heat transfer effectively without causing excessive pressure drop. The ability to print these intricate features reliably is a key advantage of AM.
5. Designing for Powder Removal:
- Trapped Powder: Unfused metal powder within the complex, often tortuous paths of conformal cooling channels is a major challenge. The design must facilitate powder removal during post-processing.
- Access Points: Incorporate strategically located access ports or openings (which might be sealed later) to allow for flushing, blowing, or vibrating powder out of the channels.
- Channel Continuity: Avoid designs that create completely isolated internal voids where powder cannot be removed. Ensure all channel sections have an entry and exit path.
- Smooth Internal Surfaces: While AM produces inherently rougher surfaces, design choices can influence this. Smoother internal surfaces (achieved via orientation, parameters, or geometry choices like avoiding sharp internal corners) can aid powder removal.
6. Leveraging Simulation Tools:
- Optimalizace topologie: Software tools can optimize material distribution for maximum stiffness or thermal performance while minimizing weight, often resulting in organic-looking structures ideal for AM. This can be applied to the overall component design incorporating the cooling channels.
- Simulace procesu: Simulating the AM build process itself can help predict potential issues like residual stress accumulation and distortion, allowing designers to modify the geometry or support strategy proactively.
Met3dp’s Role in DfAM:
Successfully implementing DfAM requires not just theoretical knowledge but also practical experience with specific AM processes and materials. Partnering with an experienced additive manufacturing services provider like Met3dp can be invaluable. Their application development services can assist clients in:
- Optimizing designs for their specific AM equipment (including industry-leading print volume and accuracy).
- Advising on material-specific design constraints (e.g., self-supporting angles for IN625 vs. AlSi10Mg).
- Leveraging simulation tools effectively.
- Developing strategies for support generation and powder removal based on extensive process knowledge.
By integrating DfAM principles, engineers can move beyond simply printing existing designs to truly harnessing metal AM for creating next-generation aerospace cooling solutions with superior performance and reliability.
Achieving Precision: Tolerances, Surface Finish, and Dimensional Accuracy in AM Cooling Channels
While metal additive manufacturing offers unparalleled geometric freedom, achieving the tight tolerances, specific surface finishes, and overall dimensional accuracy required for many aerospace applications, including conformal cooling channels, demands careful process control, understanding of material behavior, and often, post-processing steps. Procurement managers and engineers need realistic expectations about the precision achievable directly from the AM process and what finishing steps might be necessary.
1. Tolerances in Metal AM:
- General Achievable Tolerances: As a general guideline, metal AM processes like SLM and EBM can typically achieve dimensional tolerances in the range of ±0.1mm to ±0.3mm over moderate distances (e.g., 100mm), with potentially tighter tolerances (e.g., ±0.05mm) possible on smaller features with optimized process parameters and high-end machines. However, these are general figures and can vary significantly based on:
- Machine Calibration & Quality: High-precision, well-maintained machines like those emphasizing accuracy and reliability are crucial. Met3dp highlights these aspects in their equipment offerings.
- Materiál: Different materials exhibit varying degrees of shrinkage and warping during processing (e.g., IN625’s higher thermal gradients can induce more stress/distortion than AlSi10Mg).
- Part Geometry & Size: Larger parts and complex geometries with significant thermal mass variations are more prone to distortion.
- Orientace na stavbu: The orientation of the part on the build plate affects accuracy due to the layer-wise nature and support requirements.
- Parametry procesu: Laser/beam power, scan speed, layer thickness, and scan strategy all influence final dimensions.
- Aerospace Requirements: Aerospace components often demand tighter tolerances, especially for mating surfaces, interfaces, and critical flow paths. While AM might achieve near-net-shape, critical tolerances often require post-machining.
- standardy: Standards like ISO/ASTM 52900 series provide frameworks for specifying AM tolerances.
2. Surface Finish (Roughness):
- As-Built Surface Roughness: Metal AM parts inherently have a rougher surface finish compared to machined parts. The roughness depends heavily on:
- Distribuce velikosti částic (PSD): Finer, more uniform powders generally lead to smoother surfaces. This underscores the importance of vysoce kvalitní kovové prášky like those from Met3dp.
- Tloušťka vrstvy: Thinner layers typically result in smoother surfaces but increase build time.
- Surface Orientation: Surfaces built parallel to the build plate (downward-facing) or at steep angles tend to be rougher due to the “stair-stepping” effect and adherence of partially melted powder particles. Vertical walls are generally smoother. Upward-facing surfaces are often the smoothest.
- Parametry procesu: Energy input and scan strategy influence melt pool dynamics and surface texture.
- Typical Ra Values: As-built surface roughness (Ra) for SLM/EBM parts often ranges from 6 µm to 25 µm (or higher for certain orientations/materials).
- Impact on Cooling Channels: Internal channel surface roughness is particularly important:
- Pressure Drop: Rougher surfaces increase frictional losses, leading to higher pressure drop for the coolant flow.
- Heat Transfer: Roughness can sometimes enhance turbulent heat transfer, but excessive roughness might hinder flow development or create nucleation sites for boiling if not intended.
- Powder Removal: Rougher internal surfaces can make complete powder removal more difficult.
- Fatigue Life: Surface roughness can act as initiation sites for fatigue cracks, potentially impacting component life under cyclic loading.
- Zlepšení povrchové úpravy: Post-processing techniques like abrasive flow machining (AFM), chemical etching/polishing, or electro-chemical polishing (ECP) are often required to achieve smoother internal channel surfaces (e.g., Ra < 5 µm or even lower) if needed for performance or cleanability.
3. Dimensional Accuracy and Metrology:
- Controlling Distortion: Residual stresses built up during the rapid heating and cooling cycles of AM can cause part distortion, especially after removal from the build plate or during heat treatment. Strategies to control this include optimized support structures, specific scan strategies (e.g., island scanning), build plate heating, and post-build stress relief heat treatments.
- Metrology and Inspection: Verifying the dimensional accuracy and internal geometry of complex AM parts with conformal channels requires advanced metrology techniques:
- Coordinate Measuring Machines (CMM): For external dimensions and accessible features.
- Optical / Laser Scanning: To capture overall form and compare against the CAD model.
- Computed Tomography (CT) Scanning: Crucial for non-destructively inspecting and measuring internal channels, detecting defects (porosity, cracks), verifying wall thicknesses, and assessing internal surface roughness qualitatively. CT scanning is becoming an indispensable tool for qualifying critical AM aerospace parts.
- Achieving Final Accuracy: For features requiring very high precision (e.g., sealing faces, bearing interfaces, precise channel inlet/outlet diameters), CNC obrábění is typically employed as a post-processing step on the near-net-shape AM part.
Achieving Precision with Service Providers:
Working with a metal 3D printing service bureau experienced in aerospace applications is critical for achieving the required precision. They should possess:
- Calibrated, high-resolution AM systems.
- Robust process control and monitoring.
- Expertise in material-specific parameters and DfAM for tolerance control.
- Established post-processing capabilities (or strong partnerships).
- Advanced metrology equipment, including CT scanning capabilities for internal feature validation.
Understanding the interplay between design (DfAM), the chosen AM process (e.g., SLM, EBM – see Met3dp’s information on tiskových metod), material properties, and post-processing is key to successfully manufacturing aerospace conformal cooling channels that meet demanding precision requirements.
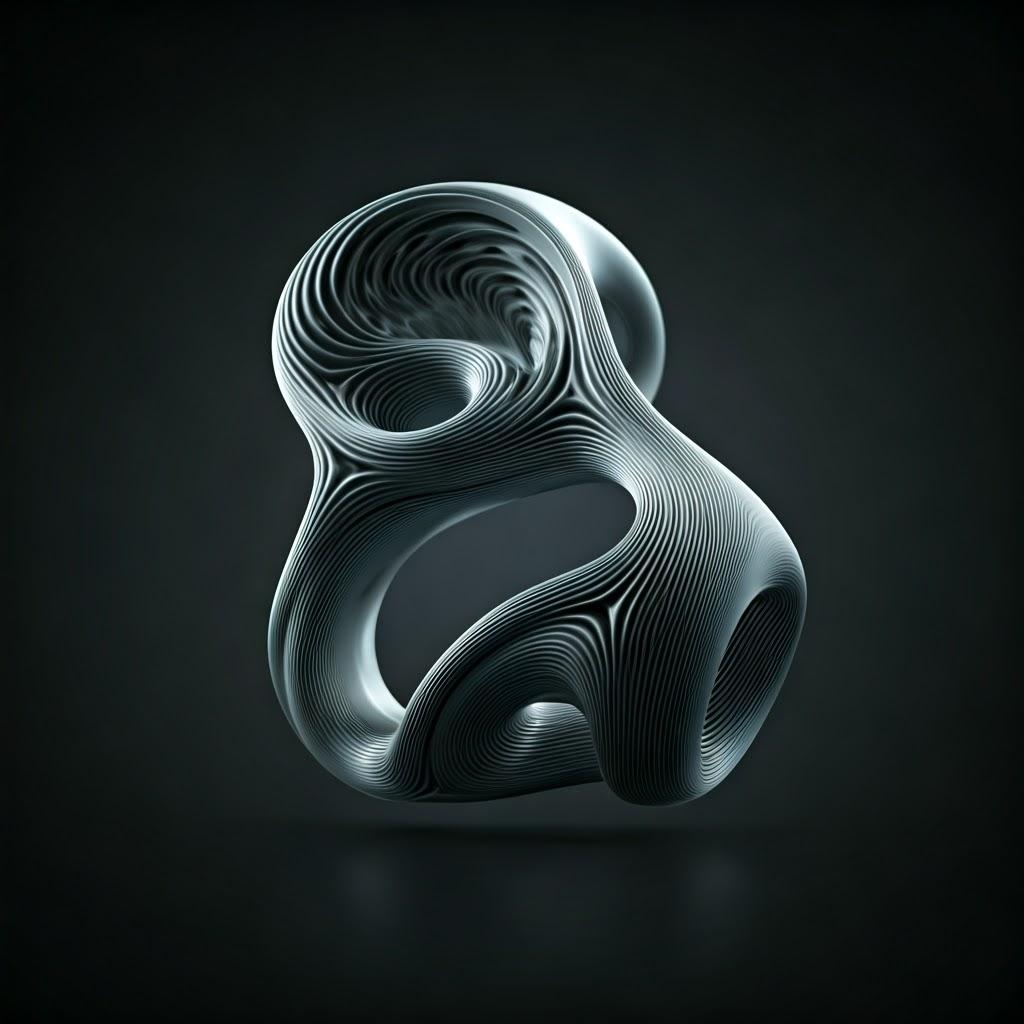
Beyond the Print: Essential Post-Processing for Aerospace Cooling Components
Producing a metal part layer-by-layer using additive manufacturing is often just the first step in the manufacturing workflow, particularly for high-performance aerospace components like conformal cooling channels. A series of metal AM post-processing steps are typically required to transform the as-built part into a functional, certified component that meets stringent aerospace standards for mechanical properties, dimensional accuracy, surface finish, and cleanliness. Neglecting or improperly executing these steps can compromise the integrity and performance of the final product.
Key post-processing stages include:
1. Stress Relief Heat Treatment:
- Účel: The rapid heating and cooling inherent in powder bed fusion processes induce significant residual stresses within the printed part. These stresses can cause distortion upon removal from the build plate or during subsequent machining, and can negatively impact fatigue life.
- Proces: Parts are typically heat-treated while still attached to the build plate in a controlled atmosphere furnace. The specific temperature cycle (heating rate, soak temperature, time, cooling rate) depends heavily on the material (e.g., different cycles for AlSi10Mg vs. IN625) and the part geometry.
- Důležitost: This is often a mandatory first step before any mechanical work (like support removal or machining) to ensure dimensional stability.
2. Part Removal from Build Plate:
- Proces: Once stress-relieved, the part(s) must be separated from the build plate. This is typically done using:
- Wire Electrical Discharge Machining (Wire EDM): Offers precision cutting with minimal induced stress.
- Band Sawing: Faster but less precise and can induce mechanical stress.
- Machining: Milling the base of the part away from the plate.
- Úvaha: The method chosen depends on part geometry, required precision at the base, and cost factors.
3. Support Structure Removal:
- Účel: Support structures, necessary during the build for overhangs and stability, must be removed.
- Proces: This can be a labor-intensive process, often involving:
- Manual breaking/cutting (for easily accessible supports).
- CNC machining (milling, grinding) for more integrated or harder-to-reach supports.
- Wire EDM or electrochemical machining in some cases.
- Výzva: Removing supports without damaging the part surface requires care. Internal supports within cooling channels are particularly challenging and ideally avoided through DfAM. Design often includes witness marks or features to guide accurate machining removal.
4. Powder Removal (Depowdering):
- Účel: Critically important for components with internal channels. All unfused powder must be removed from the intricate pathways.
- Proces: Requires a combination of methods:
- Compressed air blow-out.
- Vibration tables.
- Ultrasonic cleaning baths.
- Flushing with appropriate solvents or liquids.
- Thermal cycling (can sometimes help loosen sintered powder).
- Verification: Often verified using visual inspection (borescopes for channels), weighing the part, or CT scanning to ensure channels are clear. Incomplete powder removal can impede coolant flow, reduce thermal performance, or become loose debris causing downstream issues.
5. Hot Isostatic Pressing (HIP):
- Účel: To eliminate internal microporosity (voids) that can remain after the AM process, improve material density, and significantly enhance mechanical properties, particularly fatigue strength, ductility, and fracture toughness.
- Proces: Parts are subjected to high temperature (below melting point) and high inert gas pressure (typically Argon) simultaneously in a specialized HIP vessel. The pressure collapses internal voids, diffusion bonding the material across the void interfaces.
- Důležitost: Often mandatory for critical HIP aerospace components, especially rotating parts or those subjected to high cyclic loading, like turbine components made from IN625. It ensures material properties are closer to wrought or cast equivalents.
6. Machining (CNC):
- Účel: To achieve final dimensional tolerances on critical features, mating surfaces, interfaces (e.g., connection points for fluid lines), sealing surfaces, and to improve surface finish where required.
- Proces: Standard CNC obrábění operations (milling, turning, drilling, grinding) are performed on the near-net-shape AM part. Fixturing complex AM geometries can be challenging.
- Integration: DfAM should account for necessary machining stock on relevant surfaces.
7. Internal Channel Finishing:
- Účel: To improve the surface finish inside conformal cooling channels if the as-built roughness is insufficient for flow requirements or cleanliness standards.
- Proces: Specialized techniques may include:
- Abrasive Flow Machining (AFM): Pumping a putty-like media laden with abrasive particles back and forth through the channels to smooth surfaces and round edges.
- Chemical Polishing/Etching: Using chemical solutions to selectively remove material, smoothing the surface. Requires careful control and material compatibility.
- Electrochemical Polishing (ECP): Similar to chemical polishing but uses an electrical current to enhance material removal.
8. Surface Treatments and Coatings:
- Účel: To enhance wear resistance, corrosion protection, thermal barrier properties, or simply for cosmetic reasons.
- Proces: Depending on requirements, treatments can include:
- Shot peening (improves fatigue life).
- Polishing or Electropolishing (external surfaces).
- Anodizing (for aluminum alloys like AlSi10Mg).
- Painting or Powder Coating.
- Specialized aerospace coatings (e.g., thermal barrier coatings (TBCs) for hot section components).
9. Inspection and Testing:
- Účel: Final verification that the part meets all specifications.
- Proces: Includes:
- Dimensional inspection (CMM, scanning).
- Non-Destructive Testing (NDT): CT scanning (internal geometry/defects), Fluorescent Penetrant Inspection (FPI) (surface defects), Ultrasonic Testing (UT) (internal defects).
- Leak Testing: Essential for cooling channels to ensure integrity. Pressure testing or helium leak testing might be employed.
- Material certification checks.
Workflow Management:
Managing this complex sequence of post-processing steps requires significant expertise and coordination. Metal AM service providers like Met3dp often work with a network of qualified partners for specialized processes like HIP, NDT, and coating, offering a more streamlined workflow for their customers. Having access to robust printing technology, as detailed on pages like Met3dp’s product overview, is the starting point, but ensuring the entire end-to-end process is managed effectively is key to delivering flight-ready aerospace components.
Navigating Challenges: Overcoming Hurdles in AM Conformal Cooling Production
While metal additive manufacturing unlocks incredible potential for complex aerospace components like conformal cooling channels, the technology is not without its challenges. Understanding these potential hurdles and implementing effective mitigation strategies is crucial for successful adoption and production. Engineers and procurement specialists should be aware of common issues encountered during the manufacturing of AM parts with intricate internal geometries:
1. Residual Stress and Warping:
- Problem: The rapid, localized heating and cooling cycles inherent in laser or electron beam powder bed fusion create significant thermal gradients. As layers solidify and contract, internal stresses build up within the part. These stresses can cause:
- Warping/Distortion: The part may deform during the build, after removal from the build plate, or during post-process heat treatment.
- Cracking: In susceptible materials or poorly designed parts, high residual stress can lead to cracking during the build or later in service.
- Materials Affected: While present in most metals, it’s particularly challenging in materials with high thermal expansion coefficients or those processed at very high temperatures, like nickel superalloys (IN625) and certain titanium alloys.
- Strategie zmírnění dopadů:
- Optimized Support Structures: Well-designed supports anchor the part firmly to the build plate, resisting deformation during the build.
- Build Plate Heating: Pre-heating the build plate (common in EBM, increasingly used in SLM) reduces thermal gradients between the solidified part and the surrounding powder/plate.
- Scan Strategy Optimization: Using specific patterns (e.g., island scanning, alternating hatch directions) distributes heat input more evenly and reduces localized stress peaks.
- Process Parameter Control: Fine-tuning laser/beam power, speed, and focus affects the melt pool size and cooling rate.
- DfAM: Designing parts with more uniform cross-sections, avoiding large thermal masses connected by thin sections, and incorporating stress-relieving features.
- Post-Build Stress Relief: A critical heat treatment step performed before removing the part from the build plate.
2. Trapped Powder Removal:
- Problem: Ensuring complete removal of unfused metal powder from long, narrow, and tortuous internal cooling channels is a significant challenge. Trapped powder can:
- Block or restrict coolant flow, impairing thermal performance.
- Become sintered (partially fused) making it even harder to remove.
- Become loose debris during operation, potentially damaging downstream components.
- Strategie zmírnění dopadů:
- DfAM for Depowdering: Designing channels with adequate diameters (avoiding extremely fine passages), smooth bends, and strategically placed access ports for cleaning. Avoiding dead-end cavities.
- Optimized Build Orientation: Orienting the part to facilitate powder drainage via gravity during the build and after.
- Effective Depowdering Techniques: Utilizing a combination of compressed air, vibration, ultrasonic cleaning, and potentially specialized flushing rigs.
- Kvalita prášku: Highly spherical powders with good flowability (a focus of suppliers like Met3dp) are generally easier to remove than irregular powders.
- Verification: Using borescopes, airflow tests, weighing, or CT scanning to confirm channels are clear.
3. Porosity:
- Problem: Small voids or pores can form within the printed material due to incomplete fusion between layers, gas entrapment from the powder or shield gas, or keyholing (vapor depression collapse) from excessive energy input. Porosity can:
- Reduce mechanical properties (especially fatigue strength and ductility).
- Act as crack initiation sites.
- Potentially create leak paths if pores link up.
- Strategie zmírnění dopadů:
- Optimalizované parametry procesu: Precise control over energy density (power, speed, hatch spacing, layer thickness) is crucial to ensure full melting and fusion without overheating. Different additive manufacturing methods might have varying sensitivities.
- Vysoce kvalitní prášek: Using powder with low internal gas porosity, controlled chemistry, and consistent PSD minimizes gas entrapment issues. Met3dp’s use of advanced atomization (Gas Atomization, PREP) targets low-porosity powders.
- Proper Shielding Gas: Maintaining a high-purity inert gas atmosphere (Argon or Nitrogen) in the build chamber prevents oxidation and contamination.
- Izostatické lisování za tepla (HIP): Highly effective at closing internal porosity, significantly improving material integrity (often mandatory for critical parts).
- Detection: CT scanning is the primary method for detecting and quantifying internal porosity non-destructively.
4. Ensuring Leak Integrity:
- Problem: For cooling channels carrying fluids, preventing leaks through the channel walls or at interfaces is paramount. Leaks can arise from interconnected porosity, cracks, or defects at interfaces.
- Strategie zmírnění dopadů:
- Robust DfAM: Ensuring adequate wall thicknesses, avoiding stress concentrations.
- Process Control & Parameter Optimization: Minimizing porosity and cracking during the build.
- HIP: Closing internal voids significantly reduces the risk of interconnected porosity leading to leaks.
- Post-Machining: Ensuring smooth, precise sealing surfaces at interfaces.
- Rigorous Leak Testing: Implementing appropriate pressure decay, tracer gas (e.g., Helium), or hydrostatic testing protocols as part of the quality assurance process.
5. Surface Finish Control:
- Problem: Achieving the required internal surface finish for optimal fluid dynamics or cleanliness can be challenging due to the nature of layer-wise manufacturing.
- Strategie zmírnění dopadů:
- Optimized Orientation and Parameters: Selecting build orientation and parameters known to produce smoother surfaces on critical internal features.
- DfAM: Using channel shapes less prone to down-skin roughness.
- Následné zpracování: Employing techniques like AFM or chemical/electrochemical polishing if as-built finish is insufficient.
Addressing these aerospace additive manufacturing challenges requires a combination of robust design practices (DfAM), meticulous process control during the AM build, high-quality materials, appropriate post-processing techniques, and thorough inspection and testing protocols. Partnering with experienced poskytovatelé služeb v oblasti AM kovů who understand these challenges and have proven mitigation strategies is key to successfully implementing AM for demanding applications like aerospace conformal cooling channels.
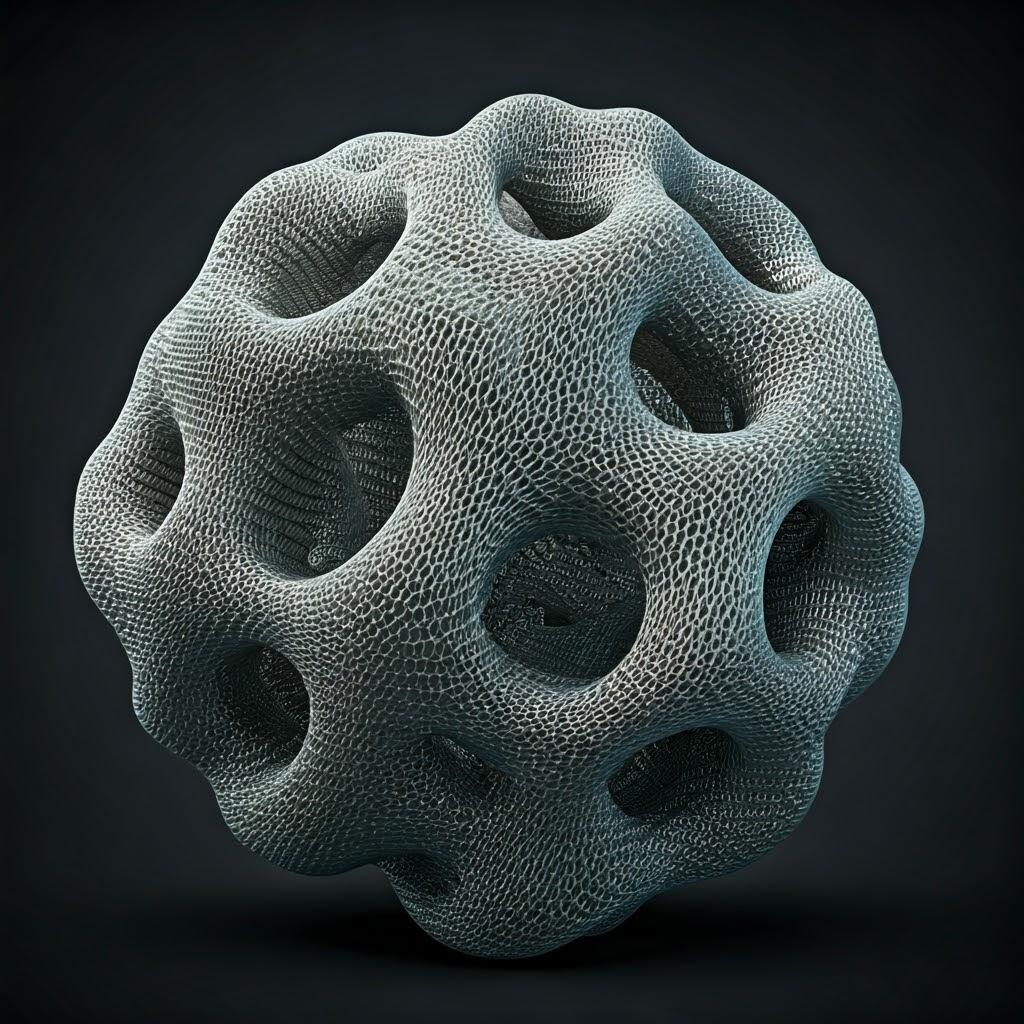
Partnering for Success: Choosing Your Metal AM Service Provider for Aerospace Applications
Selecting the right manufacturing partner is always critical, but for complex, high-consequence aerospace components like conformal cooling channels produced via metal additive manufacturing, the choice demands exceptional diligence. Not all poskytovatelé služeb v oblasti AM kovů possess the specialized expertise, rigorous quality systems, and certified processes required to meet the demanding standards of the aerospace industry. Engineers and procurement managers must evaluate potential partners based on a comprehensive set of criteria to ensure successful outcomes and mitigate risks.
Here’s what to look for when selecting your aerospace AM supplier:
1. Aerospace Certifications and Quality Management System (QMS):
- Certifikace AS9100: This is the internationally recognized QMS standard for the aviation, space, and defense industries. Certification to AS9100 (or equivalent standards like EN 9100) demonstrates that the provider has implemented rigorous processes for quality control, traceability, risk management, and continuous improvement specifically tailored to aerospace requirements. It is often a non-negotiable prerequisite for aerospace certified 3D printing.
- ISO 9001 Certification: A foundational QMS standard, indicating robust general quality processes.
- Documented QMS: Beyond certifications, review their quality manual. Understand their processes for material receiving inspection, powder handling and traceability, build process monitoring, parameter control, non-conformance management, corrective actions, and final inspection.
2. Machine Capabilities and Technology:
- Vhodná technologie AM: Do they operate the right type of metal AM machines (e.g., SLM, EBM) suitable for your chosen material (AlSi10Mg, IN625) and application requirements (resolution, surface finish, productivity)?
- Machine Fleet & Condition: Assess the size, age, and maintenance status of their machine fleet. Do they have sufficient capacity and redundancy? Are the machines calibrated regularly? Providers like Met3dp, offering their own line of printers emphasizing industry-leading print volume, accuracy and reliability, demonstrate a deep understanding of the hardware requirements.
- Objem sestavení: Ensure their machines can accommodate the size of your component.
- Monitorování procesů: Inquire about in-situ monitoring capabilities (e.g., melt pool monitoring, thermal imaging) which can provide valuable data for quality assurance.
3. Material Expertise and Handling:
- Proven Experience with Aerospace Alloys: Demonstrated success printing the specific alloys required (e.g., AlSi10Mg, IN625, Ti6Al4V) is crucial. Ask for examples or case studies.
- Powder Sourcing and Quality Control: Where do they source their powders? Do they have stringent incoming inspection and testing procedures for powder quality (PSD, morphology, chemistry, flowability)? Do they use high-quality powders from reputable suppliers or manufacturers like Met3dp, known for their advanced powder making systems (Gas Atomization, PREP)?
- Powder Management and Traceability: Strict protocols for powder handling, storage (preventing contamination and moisture uptake), segregation between different alloys, and lot traceability throughout the manufacturing process are essential for aerospace compliance. How is powder lifecycle (usage, recycling) managed and documented?
4. Post-Processing Capabilities (In-House or Managed Network):
- Comprehensive Workflow: As discussed previously, post-processing is critical. Does the provider offer necessary services like stress relief, HIP, support removal, CNC machining, surface finishing (AFM, polishing), NDT, and leak testing either in-house or through a network of qualified and audited partners?
- Integrated Management: Having a single point of contact who manages the entire workflow, including external partners, simplifies project management and ensures accountability.
5. Engineering Support and DfAM Expertise:
- Design for Additive Manufacturing (DfAM) Support: Can their engineering team provide guidance on optimizing your design for printability, performance, and cost-effectiveness? Can they assist with topology optimization or support structure strategy?
- Simulace procesu: Do they utilize build process simulation tools to predict and mitigate potential issues like distortion?
- Application Knowledge: Do they understand the specific challenges and requirements of aerospace thermal management applications? Met3dp, with its focus on industrial applications and služby vývoje aplikací, positions itself as more than just a printer; they are a solutions provider.
6. Inspection and Metrology Capabilities:
- Advanced Equipment: Access to appropriate metrology tools, including CMMs, 3D scanners, and critically, CT scanning for non-destructive internal inspection of cooling channels, is vital.
- Qualified Personnel: Certified NDT inspectors and metrology technicians are required.
- Podávání zpráv: Clear, comprehensive inspection reports documenting compliance with specifications are essential deliverables.
7. Track Record and Reputation:
- Aerospace Experience: Have they successfully delivered similar projects for other aerospace clients? Can they provide references or case studies (while respecting confidentiality)?
- Reliability and Communication: Assess their responsiveness, communication clarity, and commitment to meeting deadlines.
Evaluating Potential Partners:
Kritéria | Key Questions to Ask | Why It Matters for Aerospace Cooling Channels | How Met3dp Aligns (Based on Provided Info) |
---|---|---|---|
Certifications & QMS | Are you AS9100 certified? Can we review your QMS documentation? How do you ensure traceability? | Ensures compliance, process control, risk management critical for flight safety. | While AS9100 isn’t explicitly stated, focus on “industrial applications,” “accuracy,” “reliability” suggests commitment to high standards. QMS is implied by focus on quality powders/equipment. |
Machine Capabilities | Which AM machines/technology do you use? What’s your build volume? How are machines calibrated and maintained? | Ensures capability for specific materials, part size, required precision. | Offers own SEBM printers; emphasizes industry-leading volume, accuracy, reliability. |
Materiálová odbornost | What’s your experience with AlSi10Mg/IN625? How do you qualify & manage powders? Can you provide material certs? | Guarantees correct material properties, minimizes defects from powder issues. | Specializes in high-performance powders (AlSi10Mg, IN625, Ti alloys, superalloys); uses advanced atomization (Gas/PREP); emphasizes quality. |
Následné zpracování | What post-processing do you offer in-house? How do you manage external partners (e.g., for HIP, NDT)? | Ensures a complete, managed workflow from print to finished part. | Provides “comprehensive solutions,” implying management of necessary steps. |
Engineering Support (DfAM) | Can you assist with design optimization? Do you offer build simulation? What’s your aerospace application expertise? | Leverages AM benefits fully, prevents costly errors, ensures functional design. | Offers “application development services”; collective expertise in metal AM. |
Inspection & Metrology | What inspection methods do you use (esp. internal)? Do you have CT scanning? How do you verify leak integrity? | Crucial for verifying internal channels, detecting defects, ensuring part integrity. | Focus on “accuracy” suggests strong metrology, though specific methods need confirmation. |
Track Record | Can you share relevant aerospace case studies or references? | Builds confidence in their ability to deliver complex, critical parts. | Positions itself as a “leader in metal AM,” implying a strong track record in relevant fields (aerospace, medical, automotive). |
Export do archů
Výběr správného metal AM partner is an investment in quality, reliability, and success. Thorough vetting against these criteria will help ensure your additively manufactured conformal cooling channels meet the rigorous demands of the aerospace industry. Learning more about potential partners, perhaps starting with their company profile like Met3dp’s O nás page, is a good first step.
Understanding Investment: Cost Drivers and Lead Times for AM Conformal Cooling Channels
While metal additive manufacturing enables revolutionary designs like conformal cooling channels, understanding the associated costs and production timelines is essential for project planning and budgeting. Both metal AM cost analysis a doba realizace aditivní výroby estimation involve several interacting factors specific to the technology and the application’s complexity.
Cost Drivers for AM Conformal Cooling Channels:
The final price of an additively manufactured component is influenced by more than just the raw material weight. Key cost drivers include:
- Design Complexity & Part Geometry:
- Podpůrné struktury: Designs requiring extensive support structures (especially internal ones) increase material consumption, print time, and post-processing labor for removal. DfAM optimization to minimize supports directly reduces cost.
- Part Density/Volume: While AM allows for lightweighting, the actual volume of material printed directly impacts cost (material consumption and print time). Topology optimized, lattice-filled structures can be more cost-effective than solid blocks.
- Minimum Feature Sizes: Very fine features or thin walls might require slower print speeds or specialized parameters, increasing machine time.
- Material Type and Consumption:
- Powder Cost: Raw material costs vary significantly. Aerospace-grade nickel superalloys like IN625 are substantially more expensive per kilogram than aluminum alloys like AlSi10Mg or even titanium alloys. High-quality, tightly specified powders for aerospace command a premium.
- Amount of Material Used: Includes the material in the final part plus any support structures and potentially test coupons printed alongside. Efficient nesting of multiple parts on a build plate can reduce per-part material overhead associated with the build platform base.
- AM Process Time (Machine Time):
- Výška stavby: This is often the dominant factor in print time. Taller parts take longer, regardless of how many are nested on the plate. Orienting parts to minimize height can reduce print time.
- Part Volume & Density: The total volume of material to be melted directly impacts the time spent scanning each layer.
- Layer Thickness & Parameters: Thinner layers improve resolution but increase the number of layers and thus print time. Optimized parameters balance speed and quality.
- Hodinová sazba stroje: The cost of operating sophisticated metal AM systems (including depreciation, maintenance, energy, inert gas, labor) translates to an hourly rate charged for machine time.
- Požadavky na následné zpracování:
- Labor Intensity: Support removal, manual finishing, and inspection can be labor-intensive.
- Specialized Processes: Steps like HIP, CNC machining, AFM for internal channels, NDT (especially CT scanning), and specialized coatings add significant cost due to equipment, expertise, and time required. The extent of post-processing needed is a major cost variable.
- Quality Assurance and Certification:
- Inspection Level: Rigorous inspection protocols (multiple NDT methods, detailed dimensional reports, leak testing) required for aerospace add cost.
- Dokumentace: Generating comprehensive traceability documentation, material certifications, and certificates of conformity takes time and resources.
- Objem objednávky:
- Setup Costs: There are fixed costs associated with setting up each build (file preparation, machine setup, powder loading). Spreading these costs over a larger number of identical parts reduces the per-part cost.
- Learning Curve: For repeat orders, efficiencies can often be found in printing and post-processing.
Lead Time Considerations:
Additive manufacturing lead times for certified aerospace parts are often measured in weeks, not days. It’s crucial to have realistic expectations:
- Pre-Processing: Design review, DfAM checks, build simulation, file preparation, and build planning can take several days, especially for complex parts or first-time builds.
- Queue Time: The part needs to be scheduled onto an available machine, which depends on the service provider’s backlog.
- Print Time: Can range from many hours to several days, depending primarily on build height and volume. A tall, complex IN625 part could easily take 5-10 days or more to print.
- Následné zpracování: This often constitutes the longest portion of the lead time.
- Cooling and Stress Relief: 1-2 days.
- Part Removal/Support Removal: 1-3 days (highly variable).
- HIP Cycle (including shipping to/from HIP provider if outsourced): 5-10 days.
- CNC Machining: 2-7 days (depending on complexity and scheduling).
- Internal Finishing (AFM, etc.): 2-5 days.
- NDT & Inspection: 2-5 days.
- Potential Iterations/Rework: Can add significant delays if issues are found.
- Doprava: Standard shipping times apply.
Typical Overall Lead Time: For a complex aerospace conformal cooling channel requiring multiple post-processing steps and rigorous QA, a typical lead time might range from 4 to 10 weeks, or potentially longer for very complex first articles.
Cost vs. Traditional Methods:
While the direct 3D printing price aerospace components might seem high compared to a simple machined part, a true comparison requires considering the Total Cost of Ownership (TCO) and value proposition:
- Performance Gains: Improved thermal efficiency can lead to significant operational savings (fuel) or enable higher performance (thrust, power density).
- Snížení hmotnosti: Reduced weight translates directly to cost savings in aerospace operations.
- Konsolidace částí: Eliminating multiple parts, assembly steps, and potential failure points reduces manufacturing complexity, inventory, and long-term maintenance risk.
- Development Speed: Faster prototyping and iteration cycles can shorten time-to-market for new aerospace systems.
Metal AM is often most cost-effective for highly complex, low-to-medium volume parts where its unique geometric capabilities provide significant performance or system-level benefits that outweigh the potentially higher per-part manufacturing cost.
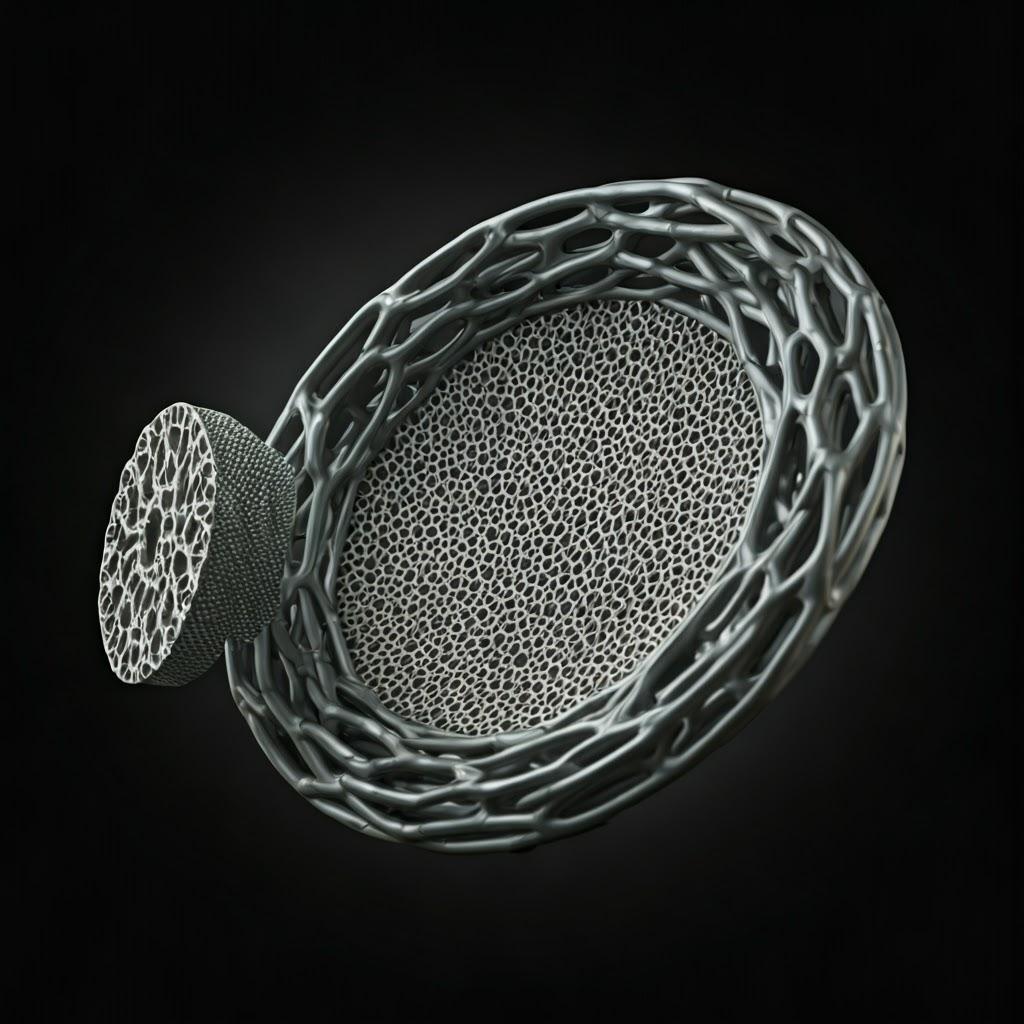
Frequently Asked Questions (FAQ): Metal AM for Aerospace Cooling Channels
Here are answers to some common questions regarding the use of metal additive manufacturing for producing conformal cooling channels in aerospace applications:
Q1: How is the performance and reliability of AM conformal cooling channels validated to ensure they meet or exceed traditional methods?
A: Validation is a multi-faceted process critical for qualifying any aerospace component, especially one manufactured using newer techniques like AM. It typically involves a combination of simulation, non-destructive testing (NDT), and physical testing:
- Computational Fluid Dynamics (CFD) & Finite Element Analysis (FEA): During the design phase (DfAM), extensive CFD simulations are used to predict fluid flow behavior (pressure drop, flow distribution) and heat transfer performance within the channels. FEA is used to analyze thermal stresses and structural integrity under operational loads and temperatures. These simulations compare the predicted performance of the AM design against baseline traditional designs or performance requirements.
- Nedestruktivní zkoušení (NDT):
- CT Scanning: Essential for verifying the internal geometry of the cooling channels post-print, ensuring they match the CAD model, checking for obstructions (trapped powder), measuring wall thicknesses, and detecting internal defects like porosity or cracks.
- Fluorescent Penetrant Inspection (FPI) / Dye Penetrant Inspection (DPI): Used to detect surface-breaking defects (cracks, porosity).
- Ultrasonic Testing (UT) / Radiographic Testing (RT): May be used in specific cases for volumetric inspection, though CT is often preferred for complex internal geometries.
- Physical Testing:
- Flow Testing: Measuring actual pressure drop versus flow rate through the channels using air or relevant coolant fluid. This validates the CFD predictions and checks for blockages.
- Thermal Performance Testing: Mounting the component in a test rig simulating operational heat loads and coolant flow conditions. Thermocouples or thermal imaging measure surface temperatures and heat dissipation rates to confirm cooling effectiveness matches design intent.
- Leak Testing: Critically important. Applying pressure (hydrostatic or pneumatic, sometimes using a tracer gas like helium) to verify the integrity of the channel walls and any interfaces.
- Proof Pressure / Burst Testing: Subjecting the component to pressures significantly higher than operating pressure to verify structural integrity and safety margins.
- Mechanical Property Testing: Printing representative “witness coupons” alongside the main part using the same material batch and parameters. These coupons undergo tensile testing, fatigue testing, etc., to verify that the material properties meet specifications after all processing steps (including HIP).
- Durability/Fatigue Testing: For components under cyclic loads (thermal cycles, pressure cycles), subjecting prototype parts to accelerated life testing to validate fatigue resistance and predict operational lifespan.
Validation relies on comparing the results of these tests against established aerospace standards, component specifications, and potentially data from traditionally manufactured counterparts. The rigorous aerospace component validation AM process ensures parts are fit for purpose and safe for flight.
Q2: What level of material traceability and process certification can be expected when sourcing critical aerospace parts via metal AM?
A: Traceability and certification are cornerstones of aerospace manufacturing safety and quality, and reputable AM service providers catering to this sector must adhere to stringent requirements, often mandated by standards like AS9100. You should expect:
- Powder Traceability: Complete “cradle-to-grave” tracking of the metal powder. This includes:
- Supplier Certificates of Conformance (CoC) for each powder batch, detailing chemical composition, particle size distribution (PSD), morphology, and flow characteristics.
- Unique identification and tracking of each powder batch used within the AM provider’s facility.
- Records linking specific powder batches (and even recycled powder usage history) to specific build jobs and individual parts.
- Process Parameter Logging: Detailed recording of all critical parameters during the AM build process for each part. This includes machine ID, operator ID, build file version, layer thickness, laser/beam power, scan speed, hatch spacing, build plate temperature, oxygen levels/gas flow, etc. This data log forms part of the part’s “birth certificate.”
- Build Traceability: Each part is uniquely identified (e.g., serial number physically marked or tagged) and linked to its specific build job, position on the build plate, and associated process/powder data.
- Witness Coupon Testing: As mentioned, test coupons are often printed with each build. Results from testing these coupons (tensile, hardness, microstructure analysis) are documented and linked to the parts produced in that build, providing verification of the material’s properties as-built and post-processed.
- Post-Processing Records: Documentation confirming that all required post-processing steps (stress relief, HIP, machining, NDT) were performed according to specified procedures, including records of parameters used (e.g., HIP charts, machining reports, NDT results).
- Certificates of Conformity (CoC): The final deliverable document from the AM service provider, certifying that the part conforms to the purchase order requirements, drawing specifications, and relevant aerospace standards. This CoC summarizes or references all the supporting traceability and testing documentation.
This comprehensive material traceability additive manufacturing system ensures accountability and allows for thorough investigation if any issues arise later in the component’s lifecycle.
Q3: For complex conformal cooling channels, is metal AM actually cost-competitive with traditional manufacturing methods?
A: This is a nuanced question without a simple yes/no answer. It depends heavily on the specific component, its complexity, the production volume, and how “cost” is defined (per-part cost vs. total lifecycle value).
- Direct Per-Part Cost: For relatively simple geometries manufacturable via conventional drilling/milling/brazing, traditional methods may have a lower per-part cost, especially at high volumes where tooling amortization is spread widely. Metal AM often involves higher capital equipment costs, more expensive raw materials (especially high-quality powders), and potentially extensive post-processing, leading to a higher direct manufacturing cost per part, particularly for simpler designs or very high volumes.
- Where AM Becomes Competitive:
- Extreme Complexity: When the conformal cooling channel design is so intricate (highly curved paths, internal features, variable cross-sections) that it becomes physically impossible or prohibitively expensive and time-consuming to make traditionally (requiring numerous complex machining setups, intricate brazing/welding operations with high failure rates), AM becomes the enabling technology and thus cost-effective by comparison, as it může produce the part.
- Konsolidace částí: If AM allows consolidating multiple traditionally manufactured pieces (e.g., a manifold block, multiple tubes, mounting brackets) into a single printed component, the savings in assembly labor, reduced part count (inventory, logistics), and elimination of joints (potential leak points, fasteners) can offset the higher printing cost.
- Performance & Efficiency Gains (Lifecycle Value): This is often the strongest argument for AM in aerospace. If the superior thermal performance enabled by conformal cooling leads to significant improvements in engine fuel efficiency, increased component lifespan (reducing replacement costs), or allows for lighter overall system design (compounding fuel savings), these operational benefits over the aircraft’s life can far outweigh a higher initial part cost. This is a key aspect of metal 3D printing ROI analysis.
- Rapid Prototyping & Development: AM significantly accelerates the design-build-test cycle for complex prototypes, reducing development time and costs compared to traditional methods requiring tooling.
- Low-to-Medium Volume Production: AM avoids the high upfront tooling costs associated with casting or injection molding, making it more economical for production runs where these tooling costs cannot be amortized effectively.
Conclusion on Cost: Metal AM is typically not chosen for conformal cooling channels primarily to save on the direct manufacturing cost of the channel itself compared to a simple traditional design. It’s chosen because it enables složité konstrukce that deliver performance, weight, or reliability benefits unattainable through traditional means. The cost justification often comes from the system-level value and lifecycle benefits derived from these enhanced capabilities, making it cost-competitive or even superior when considering the total picture.
Conclusion: The Future is Cool – Embracing Metal AM for Next-Generation Aerospace Thermal Solutions
The challenge of managing heat effectively remains a critical bottleneck in pushing the boundaries of aerospace performance. Traditional manufacturing methods, while refined over decades, inherently limit the geometric complexity possible for internal cooling features, often forcing compromises between thermal efficiency, manufacturability, and weight. As we’ve explored, Metal Additive Manufacturing (Metal AM) represents a fundamental paradigm shift, shattering these limitations and ushering in a new era of aerospace thermal management solutions.
The ability to create Conformal Cooling Channels – intricate pathways that precisely follow the contours of critical components like turbine blades, engine casings, and avionics modules – is arguably one of AM’s most impactful contributions to the aerospace sector. By placing cooling exactly where it’s needed most, AM enables:
- Unprecedented Thermal Performance: Achieving more uniform temperature distributions, eliminating hot spots, and allowing components to operate reliably at higher temperatures or power densities, leading directly to enhanced engine efficiency, greater thrust, and improved electronics reliability.
- Revolutionary Design Freedom: Empowering engineers to move beyond the constraints of drilling and milling, utilizing organic shapes, variable cross-sections, and integrated flow-enhancing features optimized purely for thermal and structural performance through DfAM.
- Significant Weight Reduction: Enabling lightweight designs through topology optimization and part consolidation, directly contributing to fuel savings and increased payload capacity – crucial metrics in aerospace economics.
- Enhanced Reliability: Consolidating multiple parts into single, monolithic components eliminates joints, brazes, and welds, drastically reducing potential leak paths and simplifying assembly. Rigorous process control and post-processing like HIP further enhance material integrity.
However, realizing these benefits requires a holistic approach. Success hinges on meticulous Design pro aditivní výrobu (DfAM), careful selection of appropriate high-performance materials like AlSi 10Mg nebo IN625 sourced as vysoce kvalitní kovové prášky, diligent execution of essential následné zpracování steps (including stress relief, HIP, machining, and finishing), and rigorous validation through advanced inspection and testing.
Navigating the complexities of process optimization, material science, and quality assurance demands expertise. Partnering with the right Poskytovatel služeb metal AM is therefore paramount. Choosing a supplier with proven aerospace experience, AS9100 certification, robust quality management systems, advanced equipment, material expertise, comprehensive post-processing capabilities, and strong engineering support is not just advisable – it’s essential for producing flight-worthy components.
Met3dp stands at the forefront of this technological wave, offering a synergistic combination of industry-leading metal 3D printing equipment a advanced spherical metal powders. With decades of collective expertise and a commitment to enabling next-generation manufacturing, Met3dp provides the comprehensive solutions – spanning printers, premium materials like TiNi, TiTa, TiAl, TiNbZr, CoCrMo, stainless steels, and superalloys, and application development services – needed to tackle the most demanding aerospace challenges. Our focus on powder quality through cutting-edge Gas Atomization and PREP technologies, combined with the accuracy and reliability of our printing systems, provides the foundation for manufacturing mission-critical parts.
The future of aerospace is inextricably linked with advancements in manufacturing technology. Metal AM, particularly for applications like conformal cooling, is not just an alternative production method; it is a key enabler for developing lighter, faster, more efficient, and more reliable aircraft and spacecraft. Embracing this technology today paves the way for the aerospace innovations of tomorrow.
Ready to explore how metal additive manufacturing can revolutionize your thermal management challenges? Návštěva Met3dp to learn more about our comprehensive Met3dp aerospace solutions and discover how our cutting-edge systems and high-performance powders can power your organization’s additive manufacturing goals. Contact us today to start the conversation.
Sdílet na
Facebook
Cvrlikání
LinkedIn
WhatsApp
E-mailem
MET3DP Technology Co., LTD je předním poskytovatelem řešení aditivní výroby se sídlem v Qingdao v Číně. Naše společnost se specializuje na zařízení pro 3D tisk a vysoce výkonné kovové prášky pro průmyslové aplikace.
Dotaz k získání nejlepší ceny a přizpůsobeného řešení pro vaše podnikání!
Související články
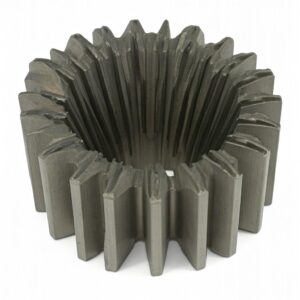
Vysoce výkonné segmenty lopatek trysek: Revoluce v účinnosti turbín díky 3D tisku z kovu
Přečtěte si více "
13. května 2025
Žádné komentáře
O Met3DP
Nedávná aktualizace
Náš produkt
KONTAKTUJTE NÁS
Nějaké otázky? Pošlete nám zprávu hned teď! Po obdržení vaší zprávy obsloužíme vaši žádost s celým týmem.
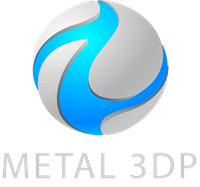
Kovové prášky pro 3D tisk a aditivní výrobu
SPOLEČNOST
PRODUKT
kontaktní informace
- Město Qingdao, Shandong, Čína
- [email protected]
- [email protected]
- +86 19116340731