Custom Tooling with Metal 3D Printing for Manufacturing
Obsah
Introduction: Revolutionizing Manufacturing with 3D Printed Custom Tooling
In the relentless pursuit of efficiency, speed, and innovation, modern manufacturing industries – from aerospace and automotive to medical device production and consumer goods – are constantly seeking transformative technologies. Custom tooling, encompassing jigs, fixtures, molds, dies, and other production aids, forms the bedrock of repeatable, high-quality manufacturing processes. Traditionally, producing this essential tooling has been a significant bottleneck, often characterized by long lead times, high costs, and design limitations inherent in subtractive methods like CNC machining or casting. However, the advent and maturation of výroba aditiv kovů (AM), commonly known as metal 3D printing, is fundamentally reshaping the landscape of custom tooling solutions. This technology offers an unprecedented ability to create highly complex, performance-optimized tooling with remarkable speed and growing cost-effectiveness, driving a paradigm shift in how manufacturers approach production setup and execution.
Metal 3D printing moves beyond the constraints of traditional methods by building parts layer by layer directly from digital models using specialized metal powders. This additive approach unlocks geometric freedoms previously unattainable, allowing for the integration of intricate internal features, topology-optimized lightweight structures, and, crucially for many tooling applications, conformal cooling channels that precisely follow the contours of the tool’s surface. The implications for manufacturing efficiency are profound. Faster tool iteration cycles, reduced material waste, extended tool life through optimized designs and materials, and improved final part quality due to superior thermal management are just a few of the benefits compelling engineers and procurement managers to explore and adopt additive manufacturing tooling.
Imagine slashing the lead time for a complex injection mold insert from months to weeks, or even days. Consider producing a lightweight, ergonomic assembly jig perfectly contoured to a specific component, improving worker efficiency and reducing fatigue. Visualize stamping dies with integrated cooling, drastically reducing cycle times and wear. These are not futuristic concepts; they are tangible realities enabled by metal 3D printing. This technology empowers manufacturers to respond more agilely to market demands, customize production lines rapidly, and achieve levels of performance previously considered impossible. As a leading provider of advanced additive manufacturing solutions, Met3dp recognizes the transformative potential of this technology for custom tooling. Leveraging industry-leading Selective Electron Beam Melting (SEBM) printers and high-performance metal powders produced via state-of-the-art Gas Atomization and Plasma Rotating Electrode Process (PREP) technologies, Met3dp empowers businesses to harness the full potential of metal 3D printing introduction for their critical tooling needs. This isn’t just about making tools differently; it’s about fundamentally improving the entire manufacturing value chain.
The transition towards additive manufacturing for tooling is not merely a trend but a strategic imperative for companies aiming to maintain a competitive edge. It addresses core manufacturing challenges: reducing time-to-market, lowering production costs, enhancing part quality, and enabling greater design innovation. For procurement managers seeking reliable custom tooling solutions suppliers, understanding the capabilities and nuances of metal AM is crucial. For design and manufacturing engineers, embracing Design for Additive Manufacturing (DfAM) principles unlocks the technology’s full potential. This article serves as a comprehensive guide for technical and purchasing professionals, exploring the diverse applications, compelling advantages, critical material considerations, design best practices, quality expectations, post-processing needs, potential challenges, supplier selection criteria, cost factors, and frequently asked questions surrounding the use of metal 3D printing for custom tooling. Join us as we delve into how this revolutionary technology is forging the future of efficient, agile, and high-performance manufacturing.
Diverse Applications: Where Metal 3D Printed Tooling Excels
The versatility of metal additive manufacturing allows it to address a vast spectrum of custom tooling requirements across numerous industries. Its ability to create complex geometries quickly and utilize high-strength, durable materials makes it an ideal solution for applications where traditional methods fall short in terms of speed, cost, or performance optimization. Procurement managers looking for B2B suppliers and wholesale options for industrial tooling use cases will find that metal AM offers compelling advantages for several key categories:
1. Jigs and Fixtures: These devices are essential for locating, supporting, and guiding workpieces or tools during manufacturing operations like assembly, machining, welding, or inspection.
- Traditional Challenges: Often require complex machining, leading to long lead times and high costs, especially for low-volume or highly customized designs. Weight can also be a factor in manual operations.
- AM Advantage:
- Rychlost: Rapid prototyping and production of custom jigs and fixtures in days, not weeks or months.
- Complexity & Conformance: Ability to create highly ergonomic shapes that perfectly match complex part geometries, improving accuracy and ease of use. Internal lattice structures can significantly reduce weight without sacrificing stiffness, crucial for handheld devices.
- Consolidation: Multiple components of a fixture assembly can often be consolidated into a single 3D printed part, reducing assembly time and potential points of failure.
- Příklady: Assembly jigs for aerospace components, CMM fixtures for quality control, welding fixtures for automotive frames, surgical guides (a type of medical fixture). Sourcing 3D printed jigs and fixtures enables faster line setup and changeovers.
2. Molds (Injection Molding, Blow Molding, Thermoforming): Tooling inserts, cores, and cavities used in various molding processes benefit immensely from AM, particularly due to the potential for conformal cooling.
- Traditional Challenges: Machining deep, complex cooling channels is difficult, expensive, and often impossible. This leads to sub-optimal cooling, longer cycle times, part warpage, and reduced tool life.
- AM Advantage:
- Conformal Cooling: The defining benefit. 3D printing allows cooling channels to be designed following the exact contours of the mold surface, typically 1-2mm beneath. This provides rapid, uniform cooling, drastically reducing cycle times (often by 20-50%+), improving part quality (less warpage, better dimensional stability), and extending mold life.
- Složitost: Enables intricate mold features, textures, and undercuts that are difficult or impossible to machine.
- Hybrid Approach: AM inserts can be integrated into conventionally machined mold bases, offering a cost-effective way to gain performance benefits where needed most.
- Příklady: Injection mold inserts for complex automotive interior parts, blow mold tooling for intricate bottle designs, thermoforming tools for packaging. Additive manufacturing molds with conformal cooling represent a significant competitive advantage.
3. Dies (Stamping, Forming, Die Casting): Tools used to cut, shape, or form materials like sheet metal or molten metal alloys face extreme stresses and temperatures.
- Traditional Challenges: High wear rates, long lead times for hard tooling, difficulty incorporating effective cooling in high-temperature processes like die casting.
- AM Advantage:
- Možnosti materiálu: Ability to print with high-strength, wear-resistant tool steels (like H13, M300) capable of withstanding demanding operations.
- Conformal Cooling (Die Casting): Similar to injection molding, integrated cooling channels can significantly improve cycle times, reduce thermal stress, minimize part soldering, and extend die life in die casting applications.
- Rapid Prototyping/Bridge Tooling: Quickly produce prototype or low-volume stamping/forming dies to validate designs before committing to expensive traditional tooling.
- Složitost: Create forming dies with complex surfaces for intricate sheet metal parts.
- Příklady: Sheet metal stamping dies for automotive body panels (especially for try-out or low volume), die casting inserts for complex aluminum components, forming tools for aerospace brackets. Sourcing metal printed dies can accelerate product development and improve high-temperature process efficiency.
4. End-of-Arm Tooling (EoAT) / Grippers: Custom grippers for robotic arms used in automated handling, assembly, and pick-and-place operations.
- Traditional Challenges: Often require assembly of multiple components, can be heavy, and customization for specific parts can be time-consuming.
- AM Advantage:
- Odlehčení: Topology optimization and lattice structures create strong yet lightweight grippers, allowing robots to move faster or handle heavier payloads.
- Přizpůsobení a složitost: Easily create grippers perfectly matched to the shape of the object being handled, improving grip security and reliability. Internal channels for vacuum or pneumatics can be directly integrated.
- Konsolidace částí: Reduce complexity and assembly time by printing monolithic gripper designs.
- Příklady: Custom grippers for handling delicate electronic components, EoAT for automotive assembly lines, specialized grippers for food processing.
Industry-Specific Applications:
- Letectví: Drill jigs, assembly fixtures, check gauges, low-volume forming tools.
- Automobilový průmysl: Stamping die prototypes, injection mold inserts, assembly fixtures, robotic grippers.
- Lékařský: Custom surgical guides, fixtures for medical device assembly, mold inserts for implant manufacturing (using biocompatible materials like Ti6Al4V or CoCrMo where needed, although tooling often uses tool steels).
- Průmyslová výroba: Machine tool components, workholding devices, forming tools, specialized repair tooling.
The breadth of these rapid tooling applications underscores the transformative impact of metal AM. By enabling faster production, complex designs, and enhanced performance through features like conformal cooling, metal 3D printing empowers manufacturers across sectors to overcome traditional tooling limitations and unlock new levels of productivity and innovation. Companies like Met3dp, with their expertise in both advanced printing technologies and material science, are key partners in realizing these benefits for diverse industrial tooling use cases.
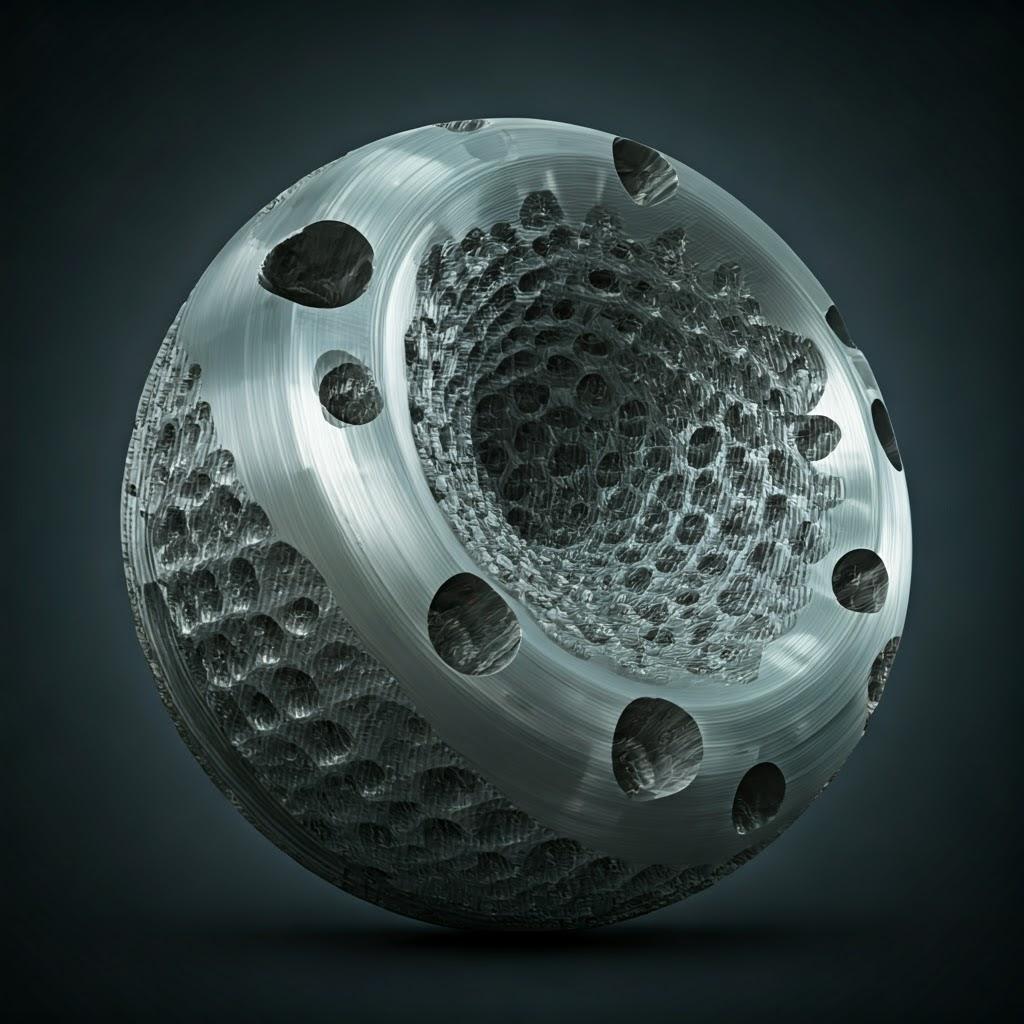
The Additive Advantage: Why Choose Metal 3D Printing for Custom Tooling?
While traditional manufacturing methods for tooling, such as CNC machining, EDM (Electrical Discharge Machining), and casting, remain relevant, metal additive manufacturing presents a compelling suite of advantages that directly address many of the inherent limitations of these older techniques. For engineers striving for optimal performance and procurement managers focused on total cost of ownership and supply chain agility, understanding these benefits is crucial when evaluating custom tooling solutions. The decision to utilize metal 3D printing often stems from its ability to deliver value across several key dimensions: speed, complexity, performance, and cost-effectiveness in specific scenarios.
1. Unprecedented Speed and Reduced Lead Times: This is arguably the most significant driver for adopting AM in tooling.
- Traditional: Creating complex tooling, especially molds and dies, can take weeks or even months due to intricate machining processes, multiple setups, and reliance on skilled labor availability. Design iterations are slow and costly.
- Additive: Metal 3D printers can often produce complex tooling components, such as mold inserts or custom fixtures, in a matter of days directly from a CAD file. This facilitates:
- Rychlé prototypování: Quickly create and test functional tooling prototypes, allowing for faster design validation and iteration.
- Nástroje pro mosty: Produce short-run tooling quickly to start production while conventional long-run tooling is being manufactured, shortening time-to-market.
- Výroba na vyžádání: Manufacture replacement or specialized tooling quickly as needed, reducing inventory requirements and downtime.
- Dopad: This acceleration dramatically shortens product development cycles and allows manufacturers to respond much faster to market changes or customer demands. Rapid tooling prototyping becomes feasible and highly effective.
2. Geometric Complexity and Design Freedom: AM builds parts layer by layer, freeing designers from many constraints imposed by subtractive methods.
- Traditional: Machining limitations restrict internal features, intricate shapes, and the ability to create certain undercuts or complex curves economically.
- Additive: Enables the creation of:
- Konformní chladicí kanály: As discussed previously, these intricate internal channels follow the shape of the tool surface, providing highly efficient and uniform cooling. This is extremely difficult or impossible to achieve conventionally but relatively straightforward with AM. The performance gains in cycle time and part quality for molding and die casting are substantial.
- Topology Optimization & Lightweighting: Algorithms can optimize tool designs to place material only where structurally needed, creating strong yet lightweight jigs, fixtures, and EoAT using internal lattice structures or organic shapes.
- Konsolidace částí: Complex assemblies can often be redesigned and printed as a single, monolithic part, reducing assembly time, potential leak paths (in cooled tools), and points of failure.
- Dopad: This allows for tooling that is not just faster to produce but performs significantly better, is more ergonomic, or enables entirely new manufacturing possibilities. Complex geometry tooling is no longer a major cost or time barrier.
3. Enhanced Performance and Tool Life: The combination of design freedom and advanced materials enables superior tooling performance.
- Conformal Cooling: Leads to faster cycles, reduced thermal stress, less part warpage, and often longer tool life due to lower peak temperatures and more uniform temperature distribution.
- Optimized Materials: AM allows the use of advanced tool steels (e.g., H13, Maraging Steels like M300/1.2709) and other high-performance alloys chosen specifically for the application’s wear, hardness, and temperature requirements. Companies like Met3dp offer a range of specialized powders optimized for demanding tooling applications.
- Surface Features: Textures, small engravings, or specific functional surfaces can be directly integrated into the tool design during printing.
- Dopad: Better performing tools lead directly to higher quality end parts, increased production throughput, and reduced operational costs.
4. Cost-Effectiveness (Context Dependent): While the initial cost per part for AM can sometimes be higher than traditional methods for simple geometries, the overall value proposition often makes it more cost-effective when considering the entire lifecycle and specific application needs.
- Reduced Lead Time Costs: Faster time-to-market translates to quicker revenue generation. Reduced downtime means lower operational losses.
- Reduced Labor: Highly automated AM processes reduce the need for constant skilled machinist oversight compared to complex multi-stage machining.
- Účinnost materiálu: AM is an additive process, typically generating less material waste (especially compared to machining from a large billet), although support structures do create some waste.
- Performance Gains: Faster cycle times and reduced scrap rates from conformally cooled tools directly impact profitability.
- Complexity “for Free”: Unlike machining, where complexity dramatically increases cost, in AM, complexity adds little to no extra cost beyond potentially longer print times or more support material.
- Tool Life Extension: Longer-lasting tools reduce replacement frequency and associated costs.
- Dopad: Při hodnocení traditional vs additive tooling, a total cost of ownership (TCO) analysis often reveals significant savings potential for AM, particularly for complex, high-performance, or time-sensitive tooling applications.
5. Customization and Agility: AM is ideally suited for producing highly customized or unique tooling components.
- Low Volume Viability: Economically produce one-off or very small batches of custom tools without the high setup costs of traditional methods.
- Easy Design Updates: Modify tool designs digitally and reprint quickly to adapt to product changes or process improvements.
- Dopad: Provides manufacturers with greater agility to adapt production lines, test new concepts, and meet niche market demands.
In summary, the compelling benefits of 3D printed tooling – speed, design freedom enabling features like conformal cooling channels, enhanced performance, potential cost savings, and agility – make it a powerful alternative and complement to traditional methods. By leveraging metal AM, manufacturers can overcome critical bottlenecks, improve operational efficiency, and unlock higher levels of productivity and innovation in their tooling strategies.
Material Matters: Selecting the Right Metal Powders for High-Performance Tooling
The success of any metal 3D printed custom tool hinges critically on the selection of the appropriate material. Tooling applications often involve demanding conditions: high mechanical stresses, elevated temperatures, abrasive wear, and the need for excellent dimensional stability. Additive manufacturing offers a growing portfolio of metal powders specifically suited to meet these challenges. Choosing the right powder requires understanding the specific requirements of the application (e.g., injection molding vs. stamping die vs. fixture) and the properties offered by different alloys. For procurement managers sourcing additive manufacturing materials suppliers and engineers specifying tooling, familiarity with common high-performance tooling powders is essential.
Met3dp, leveraging its advanced powder production capabilities including Gas Atomization and PREP technologies, manufactures a range of high-quality spherical metal powders optimized for AM processes like Selective Electron Beam Melting (SEBM) and Laser Powder Bed Fusion (LPBF). Our focus on powder quality – high sphericity, good flowability, controlled particle size distribution, and low oxygen/impurity content – ensures customers can print dense, high-integrity tooling components with superior mechanical properties. Among the most relevant materials for custom tooling are specialized tool steels and maraging steels.
Recommended Tooling Powders:
1. H13 Tool Steel:
- Popis: A versatile chromium-molybdenum-vanadium hot-work tool steel, widely used in traditional tooling and well-adapted for AM. Known for its excellent combination of high toughness, wear resistance, good thermal fatigue resistance, and dimensional stability at elevated temperatures.
- Klíčové vlastnosti:
- High hardness (typically achievable up to 45-52 HRC after heat treatment).
- Excellent temper resistance (maintains hardness at high temperatures).
- Good thermal conductivity (beneficial for cooling applications).
- High toughness and ductility (resists cracking under impact).
- Good machinability (relative to other tool steels) after annealing.
- AM Considerations: Requires careful control of printing parameters and mandatory post-print heat treatment (hardening and tempering) to achieve desired properties. Stress relief cycles are often necessary.
- Typické aplikace: Injection mold inserts & cores (especially with conformal cooling), die casting dies, extrusion dies, forging tools, forming tools, structural components requiring high strength and hardness. H13 tool steel 3D printing is a popular choice for demanding thermal and mechanical loads.
2. Maraging Steels (e.g., M300 / 1.2709 / MS1):
- Popis: A family of low-carbon, high-nickel steels strengthened by the precipitation of intermetallic compounds during aging, rather than by carbon content like traditional tool steels. They offer ultra-high strength, good toughness, and excellent dimensional stability during heat treatment. M300 (often designated similarly to 1.2709 or MS1 in AM) is a common grade.
- Klíčové vlastnosti:
- Very high hardness and strength (typically achievable up to 50-55+ HRC after simple aging heat treatment).
- Exceptional toughness compared to traditional tool steels at similar hardness levels.
- Excellent weldability (useful for repairs or hybrid manufacturing).
- Minimal and predictable distortion during low-temperature aging heat treatment (typically ~480-500°C), simplifying post-processing compared to the high-temperature quenching and tempering needed for H13.
- Good machinability in the as-printed or solution-annealed state.
- AM Considerations: Relatively easy to print with good density. The simple aging heat treatment is a significant advantage, reducing post-processing complexity and risk of distortion.
- Typické aplikace: High-performance injection molds (cores, inserts, lifters), tooling requiring high strength and toughness, intricate die casting components, high-precision fixtures, aerospace components. The M300 maraging steel AM (nebo 1.2709 powder properties) make it ideal for high-precision tooling where minimal distortion during heat treatment is critical.
Comparison Table: H13 vs. Maraging Steel (M300/1.2709) for AM Tooling
Vlastnosti | Nástrojová ocel H13 | Maraging Steel (M300 / 1.2709) | Key Advantage Summary |
---|---|---|---|
Primary Alloying | Cr, Mo, V, C | Ni, Co, Mo, Ti | Different strengthening mechanisms |
Typical Hardness (HRC) | 45-52 | 50-55+ | Maraging steel generally achieves higher hardness/strength |
Houževnatost | Dobrý | Excellent (at high hardness) | Maraging steel offers better toughness |
Tvrdost za tepla | Velmi dobře | Good (but may soften above aging temp) | H13 generally better for very high operating temps |
Tepelné zpracování | Complex (Austenitize, Quench, Temper) | Simple (Age Hardening ~480°C) | Maraging steel much simpler, less distortion risk |
Dimensional Stability (HT) | Moderate (risk of distortion) | Excellent (minimal distortion) | Maraging steel superior for precision applications |
Svařitelnost | Veletrh | Vynikající | Maraging steel easier to repair/modify |
Náklady | Generally Lower | Generally Higher | H13 often more cost-effective material |
Common AM Use | Hot work tooling, Molds, Dies | High-precision molds, High-strength tools | Both widely used, choice depends on specific needs |
Export to Sheets
Other Potential Materials: While H13 and Maraging Steels are workhorses, other materials can be considered depending on the specific tooling need:
- Stainless Steels (e.g., 17-4PH, 316L): Used for tooling requiring corrosion resistance, such as in food processing or some medical applications, or for less demanding fixtures. Often easier to print and post-process.
- Cobalt Chrome (CoCrMo): Offers excellent wear resistance, high strength at elevated temperatures, and corrosion resistance. Used for highly demanding wear components or specific medical tooling.
- Titanium Alloys (e.g., Ti6Al4V): Lightweight, strong, and corrosion-resistant. Used for lightweight fixtures, robotic grippers, or specific medical/aerospace tooling (though less common for high-wear molds/dies). Met3dp offers innovative Titanium alloys like TiNi, TiTa, TiAl, TiNbZr which could find niche tooling applications. Explore Met3dp’s diverse metal powder offerings.
- Nickel Superalloys (e.g., Inconel 718): Used for tooling operating in extremely high-temperature or corrosive environments.
Choosing the Right Material Supplier: Výběr kovový prášek pro nástroje involves more than just picking an alloy. The quality of the powder directly impacts the quality of the final tool. Partnering with a reputable supplier like Met3dp ensures:
- Consistent Chemistry: Tight control over alloy composition.
- Optimized Particle Characteristics: High sphericity, controlled size distribution (PSD), and good flowability suitable for specific AM processes (like SEBM or LPBF).
- Low Impurities: Minimizing oxygen and other contaminants that can degrade mechanical properties.
- Batch Traceability: Ensuring consistency and quality control.
- Technická podpora: Expertise to help select the best material for the application.
In conclusion, material selection is a cornerstone of successful metal 3D printed tooling. By carefully considering the application requirements and leveraging the properties of advanced materials like H13 and M300/1.2709, manufacturers can produce high-performance, durable tooling that delivers significant operational advantages. Partnering with an experienced additive manufacturing materials supplier like Met3dp provides access to high-quality powders and the expertise needed to make informed material choices.
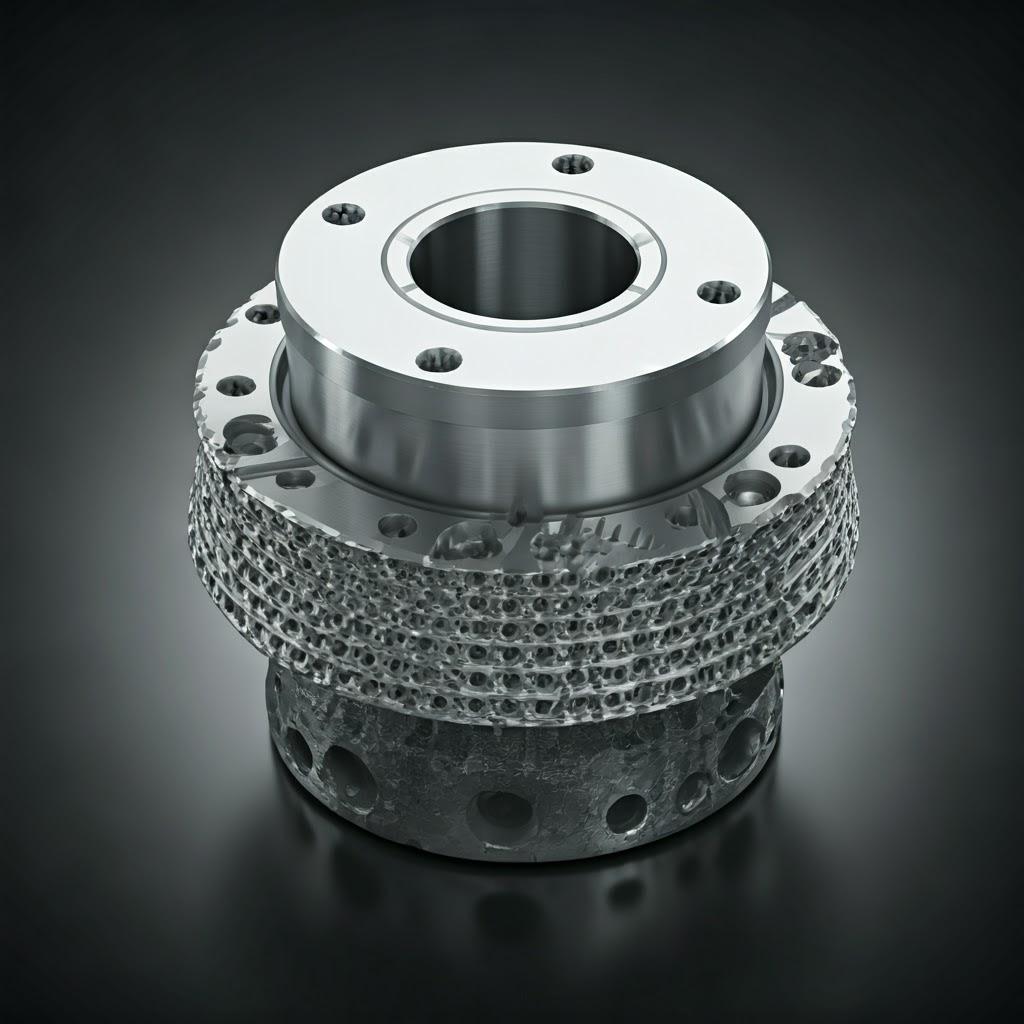
Design for Additive Manufacture (DfAM): Optimizing Tooling for Metal 3D Printing
Simply replicating a traditionally designed tool using metal additive manufacturing rarely unlocks the full potential of the technology. To truly harness the speed, complexity, and performance advantages discussed earlier, engineers must embrace Design for Additive Manufacture (DfAM). DfAM is not just a set of rules but a mindset shift, encouraging designers to think additively from the outset, considering the layer-by-layer build process, material characteristics, support structure requirements, and post-processing steps. Applying DfAM principles specifically to custom tooling – whether it’s molds, dies, jigs, or fixtures – is critical for achieving functional, cost-effective, and high-performing results. Procurement managers should also be aware of DfAM, as it directly impacts the feasibility, cost, and quality of the 3D printed tools they source. Investing in DfAM expertise, either internally or through a knowledgeable service provider like Met3dp, is key to maximizing the ROI of metal AM for tooling.
Core DfAM Principles for Custom Tooling:
- Orientation and Support Strategy:
- Build Orientation: How a part is oriented on the build plate significantly affects print time, surface finish on different faces, the amount and location of required support structures, and potential thermal stresses. For tooling, critical functional surfaces (e.g., mold cavity faces, fixture locating points) should ideally be oriented to minimize or eliminate supports, often facing upwards or being self-supporting.
- Overhangs and Angles: Metal AM processes have limitations on the angle of overhangs that can be built without support structures. Angles typically greater than 45 degrees from the horizontal are often self-supporting, but this varies by material, machine (different considerations for SEBM vs LPBF might apply, understanding various tiskových metod is helpful), and specific geometry. Designs should maximize self-supporting features where possible.
- Support Structure Design: Supports are often necessary but add material cost, print time, and post-processing effort (removal). DfAM involves:
- Minimizing Supports: Orienting the part and modifying non-critical features (e.g., using chamfers instead of sharp overhangs) to reduce reliance on supports.
- Designing for Removal: Ensuring supports are accessible for removal tools (manual or machining). Using optimized support types (e.g., lattice or tree supports) can reduce material usage and contact points. Consider adding features that aid removal, like access windows if internal supports are unavoidable.
- Support Material Considerations: Some advanced systems offer soluble or easily breakable support materials, simplifying removal.
- Feature Design and Resolution:
- Minimum Wall Thickness: AM processes have limits on how thin a feature can be reliably printed. This varies by machine and material but is typically in the range of 0.4-1.0 mm. Tooling designs must respect these limits to ensure structural integrity and printability.
- Minimum Feature Size: Small holes, pins, or intricate details also have limitations based on the laser/electron beam spot size and powder particle size. Very fine features might require post-print machining.
- Hole Orientation: Horizontal holes often print with better dimensional accuracy than vertical holes due to the layer-wise construction. Very small holes, especially horizontal ones, might require support or be better drilled after printing.
- Sharp Corners: Sharp internal corners can be stress concentration points. Using fillets or radii can improve fatigue life and reduce stress, which is critical in high-load tooling applications.
- Designing for Conformal Cooling (Molds & Dies):
- This is a prime example of DfAM enabling superior performance. Key considerations include:
- Channel Path: Design channels to follow the contours of the heat-generating surfaces (mold cavity, die face) at a consistent distance (typically 1-3 mm below the surface). Avoid sharp bends that impede flow.
- Channel Diameter & Shape: Diameters typically range from 2-10 mm. Circular cross-sections are common, but AM allows for optimized shapes (e.g., teardrop) to improve heat transfer or reduce pressure drop. Ensure diameters are large enough to be printable and avoid clogging.
- Flow Optimization: Design for turbulent flow (promotes heat transfer) and ensure balanced flow across different cooling circuits. Computational Fluid Dynamics (CFD) simulation is often used to optimize channel layouts.
- Inlets/Outlets: Design robust connections for external cooling lines, often incorporating standard fitting interfaces that may require post-machining.
- Depowdering: Ensure internal channels can be effectively cleared of unfused powder after printing. This might involve designing access ports or ensuring sufficient channel diameter.
- This is a prime example of DfAM enabling superior performance. Key considerations include:
- Lightweighting with Topology Optimization and Lattice Structures:
- Especially relevant for jigs, fixtures, and robotic EoAT where weight reduction improves ergonomics or robotic performance.
- Topology Optimization: Software tools analyze load conditions and remove material from non-critical areas, resulting in organic-looking, highly efficient structures.
- Mřížové struktury: Replacing solid volumes with internal repeating unit cells (lattices) can dramatically reduce weight and material consumption while maintaining required stiffness and strength. Different lattice types (e.g., cubic, octet truss) offer varying properties.
- Použití: Create ergonomic, lightweight assembly jigs; reduce inertia in robotic grippers; minimize material cost in large fixtures. Lattice structures tooling offers significant advantages in handling and automation.
- Konsolidace částí:
- Leverage AM’s complexity advantage to redesign multi-part tooling assemblies into a single, monolithic printed component.
- Výhody: Reduces assembly labor and time, eliminates potential leak paths (e.g., at joints in cooling channels), reduces part count and inventory management, often improves overall stiffness and strength by eliminating joints.
- Example: Combining multiple pieces of a complex fixture or integrating clamps directly into a jig body.
- Designing for Post-Processing:
- AM parts, especially tooling, almost always require post-processing. DfAM includes planning for these steps:
- Machining Allowances: Add extra stock material (e.g., 0.5-1.0 mm) to surfaces that require high precision, specific flatness, or smooth finishes achievable only through CNC machining (e.g., mold parting lines, fixture locating datums, sealing surfaces).
- Workholding Features: Incorporate features into the design that simplify holding the part during subsequent machining or finishing operations.
- Heat Treatment Considerations: Understand how the chosen material (e.g., H13 vs. M300) will respond to heat treatment and whether the design can withstand the thermal stresses without excessive distortion. Simple, robust geometries generally fare better.
- AM parts, especially tooling, almost always require post-processing. DfAM includes planning for these steps:
By thoughtfully applying these DfAM for tooling principles, manufacturers can move beyond simply substituting traditional methods and truly innovate, creating custom tooling that is faster to produce, lighter, longer-lasting, and performs better than ever before. Collaborating with AM experts like Met3dp ensures that designs are optimized not only for function but also for manufacturability using advanced metal AM design guidelines.
Precision and Quality: Understanding Tolerance, Surface Finish, and Accuracy in 3D Printed Tooling
When specifying and procuring custom tooling, achieving the required levels of precision, surface quality, and overall dimensional accuracy is paramount. Tooling often interfaces directly with final parts or other manufacturing equipment, demanding tight control over critical dimensions and surface characteristics. While metal additive manufacturing offers remarkable geometric freedom, it’s essential for engineers and procurement managers to have realistic expectations regarding the inherent tolerances and surface finish achievable directly from the printer, and to understand the role of post-processing in meeting stringent requirements. Ensuring quality control 3D printed parts intended for tooling applications involves careful process management, appropriate metrology, and often, secondary finishing operations.
Dimensional Accuracy and Tolerance:
- As-Built Accuracy: The accuracy of a metal AM part directly after printing and stress relief, but before any machining. Typical achievable tolerances depend heavily on:
- Machine Calibration: Well-maintained and calibrated machines (like the industry-leading systems utilized by Met3dp) provide better accuracy.
- Process (LPBF vs. SEBM): Different processes may have slightly different inherent accuracy capabilities. SEBM often operates at higher temperatures, potentially leading to different stress/distortion behavior than LPBF.
- Materiál: Different metal powders exhibit varying shrinkage and stress characteristics during printing and cooling.
- Part Size and Geometry: Larger parts and complex geometries are generally more prone to thermal distortion and deviation. Internal stresses built up during the print can cause warping.
- Orientation and Supports: Build orientation affects how thermal gradients accumulate, and support structures influence stability during the build.
- Typical As-Built Tolerances: While process and material specific, general guidelines for well-controlled metal AM processes might be in the range of:
- ±0.1 mm to ±0.3 mm for smaller features (< 50 mm).
- ±0.2% to ±0.5% of the nominal dimension for larger features.
- Poznámka: These are general estimates; specific capabilities should be confirmed with the AM service provider (like Met3dp, known for accuracy and reliability). Achieving tighter tolerances often requires post-machining.
- Post-Machined Tolerances: For critical dimensions, such as mold parting lines, ejector pin holes, fixture locating surfaces, or bearing interfaces, post-print CNC machining is typically employed. Machining can achieve much tighter tolerances, often comparable to traditional manufacturing:
- ±0.01 mm to ±0.05 mm is readily achievable, depending on the machining process and setup.
- Importance for Tooling: Understanding these tolerance ranges is crucial. Non-critical features of a jig might be acceptable with as-built tolerances, while the working surfaces of a precision mold insert will almost certainly require machining.
Surface Finish (Roughness):
- As-Built Surface Finish: Metal AM parts inherently have a rougher surface finish compared to machined surfaces due to the layer-by-layer process and partially melted powder particles adhering to the surface. Surface roughness (commonly measured as Ra – Arithmetic Average Roughness) depends on:
- Orientation: Upward-facing surfaces and steep angles generally have the best finish. Downward-facing surfaces supported by structures tend to be rougher after support removal. Sidewalls show layer lines.
- Tloušťka vrstvy: Thinner layers generally result in a smoother finish but increase print time.
- Powder Particle Size: Finer powders can contribute to smoother surfaces.
- Process Parameters: Laser/beam power, scan speed, etc., influence the melt pool characteristics and resulting surface.
- Typical As-Built Ra Values:
- LPBF: Often in the range of 6-15 µm Ra (240-600 µin Ra).
- SEBM: Can sometimes be slightly rougher due to process characteristics, potentially 15-35 µm Ra (600-1400 µin Ra).
- Poznámka: These are typical ranges and can vary significantly.
- Post-Processed Surface Finish: Various finishing techniques are used to improve the surface finish for tooling applications:
- Bead Blasting/Shot Peening: Provides a uniform matte finish, removes loose powder, and can impart compressive stress (improving fatigue life). Ra values might improve slightly or remain similar but become more uniform.
- Tumbling/Vibratory Finishing: Can smooth surfaces and edges, especially for smaller parts. Achievable Ra depends on media and time.
- Grinding/Polishing: Essential for mold surfaces requiring smooth finishes for part ejection or optical clarity, and for high-wear surfaces. Manual or automated polishing can achieve very smooth finishes, often < 0.1 µm Ra (< 4 µin Ra) for mirror finishes on molds.
- Obrábění: Provides a controlled, smooth surface finish typical of milling or turning operations (e.g., 0.8-3.2 µm Ra or better).
- Importance for Tooling: Surface finish impacts friction, wear resistance, part release (in molds), paint adhesion (for fixtures), and aesthetics. The required finish dictates the necessary post-processing steps. Understanding surface finish additive manufacturing capabilities and limitations is key.
Quality Control and Verification:
- Process Monitoring: Advanced AM systems incorporate in-situ monitoring (melt pool monitoring, thermal imaging) to track build consistency.
- Material Quality Control: Ensuring powder quality (chemistry, particle size, flowability) is crucial – a core focus for integrated providers like Met3dp.
- Nedestruktivní zkoušení (NDT):
- CT Scanning (Computed Tomography): Increasingly used to detect internal defects like porosity or lack of fusion, and to verify complex internal features like conformal cooling channels are clear and match the design.
- Dye Penetrant / Magnetic Particle Inspection: Used to detect surface-breaking cracks.
- Dimensional Metrology:
- CMM (Coordinate Measuring Machine): Used for high-precision verification of critical dimensions, especially after machining.
- 3D Scanning: Optical or laser scanners capture the full geometry of the printed part, allowing comparison to the original CAD model to assess overall deviation and accuracy. Useful for complex shapes where CMM is difficult.
- Hardness Testing: Verifying that the tool has achieved the specified hardness after heat treatment is critical for performance and wear resistance.
In conclusion, while metal 3D printing offers incredible design possibilities for custom tooling, achieving the necessary metal 3D printing tolerances, surface finish, and overall dimensional accuracy tooling often requires a combination of careful DfAM, controlled printing processes, and appropriate post-processing and quality verification steps. Working with an experienced partner who understands these nuances and possesses robust quality management systems is essential for obtaining reliable, high-performance tooling that meets demanding manufacturing requirements.
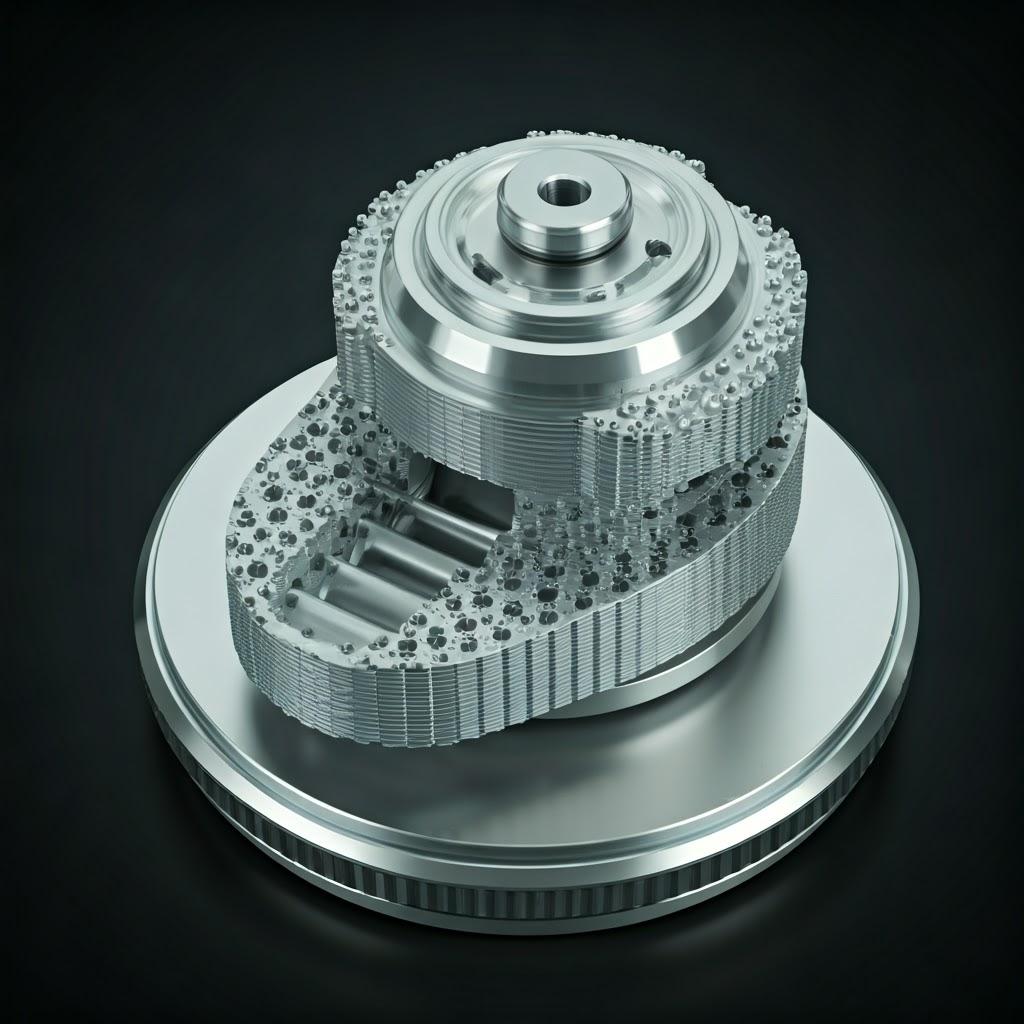
Beyond the Print: Essential Post-Processing for 3D Printed Metal Tooling
Creating a custom tool doesn’t end when the metal 3D printer finishes its job. The “green” part, fresh off the build plate, is rarely ready for immediate use in a demanding manufacturing environment. A series of critical post-processing additive manufacturing steps are almost always required to transform the as-built component into a functional, durable, and precise tool. These steps are necessary to relieve internal stresses, remove support structures, achieve the desired material properties (hardness, strength, toughness), attain critical dimensions and surface finishes, and ensure overall tool performance and longevity. Understanding these common post-processing requirements is vital for engineers designing the tools and for procurement managers factoring total cost and lead time into sourcing decisions. Ignoring or improperly executing these steps can lead to premature tool failure, poor performance, and compromised final part quality.
Common Post-Processing Stages for Metal AM Tooling:
- Úleva od stresu:
- Why it’s needed: The rapid heating and cooling cycles inherent in layer-by-layer metal AM processes create significant internal stresses within the printed part. These stresses can cause distortion, warping (especially after removal from the build plate), and even cracking if not relieved.
- Proces: Typically the first step after printing, often performed while the part is still attached to the build plate to maintain stability. Involves heating the part in a furnace to a specific temperature (below the material’s transformation temperature, e.g., ~550-650°C for H13, or an annealing cycle depending on the goal), holding it for a set duration, and then slowly cooling it. This allows the material’s microstructure to relax, reducing internal stresses.
- Importance: Absolutely critical for dimensional stability and preventing subsequent distortion or cracking during support removal, machining, or heat treatment.
- Part Removal from Build Plate:
- Proces: Once stress-relieved (if performed on the plate), the part needs to be separated from the metal build plate it was printed on. Common methods include:
- Drátové elektroerozivní obrábění (EDM): Offers precise, low-force cutting, ideal for delicate parts or when a clean cut surface is needed.
- Sawing (Band Saw): A faster, more common method for robust parts, but leaves a rougher surface that usually requires subsequent machining.
- Obrábění: Milling or grinding can also be used in some cases.
- Proces: Once stress-relieved (if performed on the plate), the part needs to be separated from the metal build plate it was printed on. Common methods include:
- Odstranění podpůrné konstrukce:
- Why it’s needed: Support structures, necessary for printing overhangs and complex geometries, must be removed.
- Proces: This can be one of the most labor-intensive steps, depending on the complexity and accessibility of the supports. Methods include:
- Ruční odstranění: Using pliers, grinders, chisels, or other hand tools. Requires skill and care to avoid damaging the part surface. Often leaves witness marks that need further finishing.
- CNC Machining: Milling or turning can precisely remove supports, especially larger or more integrated ones. Often used in conjunction with manual methods.
- Wire EDM: Can be used to access and cut supports in difficult-to-reach areas.
- DfAM Impact: Designing for support removal (accessibility, minimizing contact points) significantly impacts the ease and cost of this stage.
- Heat Treatment (Hardening/Aging):
- Why it’s needed: As-printed metal parts, even after stress relief, typically do not possess the optimal hardness, strength, or wear resistance required for tooling applications. Heat treatment develops the final desired microstructure and mechanical properties. The specific treatment depends heavily on the material.
- Proces:
- Tool Steels (e.g., H13): Requires a multi-stage process:
- Austenitizing: Heating to a high temperature (e.g., ~1000-1050°C) to dissolve carbides and form austenite.
- Kalení: Rapidly cooling (in air, oil, or inert gas) to transform austenite into hard martensite.
- Temperování: Reheating to a lower temperature (e.g., ~500-600°C, often performed multiple times) to reduce brittleness, increase toughness, and achieve the target hardness (e.g., 45-52 HRC). Heat treatment tool steel like H13 is crucial but requires careful control to minimize distortion.
- Maraging Steels (e.g., M300/1.2709): Requires a much simpler, lower-temperature aging process:
- Zpevnění věkem: Heating to a moderate temperature (typically ~480-500°C) for several hours. This causes precipitation of strengthening intermetallic compounds. No quenching is required. The major advantage is minimal distortion during this process.
- Tool Steels (e.g., H13): Requires a multi-stage process:
- Atmosphere Control: Heat treatments are typically performed in vacuum furnaces or controlled inert atmospheres to prevent oxidation and decarburization, especially at high austenitizing temperatures.
- Machining (CNC):
- Why it’s needed: To achieve tight tolerances on critical dimensions, create flat sealing surfaces (like mold parting lines), machine threads or precise holes, and obtain specific surface finishes that are better than the as-built state.
- Proces: Standard CNC machining 3D prints techniques like milling, turning, grinding, and EDM are used. Considerations include:
- Workholding: Securely fixture the potentially complex AM part.
- Material Hardness: Machining fully hardened tool steel is challenging and requires appropriate cutting tools and parameters. Machining is often easier before the final hardening step (though maraging steels are often aged after machining due to their dimensional stability).
- Stock Allowance: DfAM should include sufficient material for machining operations.
- Povrchová úprava:
- Why it’s needed: To achieve the required surface roughness for function (e.g., smooth mold surface for part release, textured surface for grip), aesthetics, or wear resistance.
- Proces: Various methods depending on the goal:
- Bead Blasting / Sand Blasting: Cleans the surface, provides a uniform matte finish, removes loose powder.
- Tumbling / Vibratory Finishing: Smoothes edges and surfaces, particularly for batches of smaller parts.
- Broušení: Used for high precision flatness and smooth finishes on specific features.
- Leštění: Manual or automated polishing, essential for injection molds requiring high gloss or optical clarity. Can achieve mirror finishes (<0.1 µm Ra). Metal part polishing is often a highly skilled, manual process for complex molds.
- Coating (e.g., PVD, Nitriding): Applying specialized coatings can further enhance wear resistance, reduce friction, or improve release properties for demanding tooling applications.
The specific sequence and combination of these surface finishing tooling and other post-processing steps will vary based on the tool type, material, and application requirements. It’s crucial for manufacturers to partner with AM service providers like Met3dp who possess not only advanced printing capabilities but also comprehensive post-processing expertise and equipment, ensuring a streamlined workflow from digital file to finished, functional tool. Neglecting this “beyond the print” stage undermines the significant investments made in additive manufacturing technology.
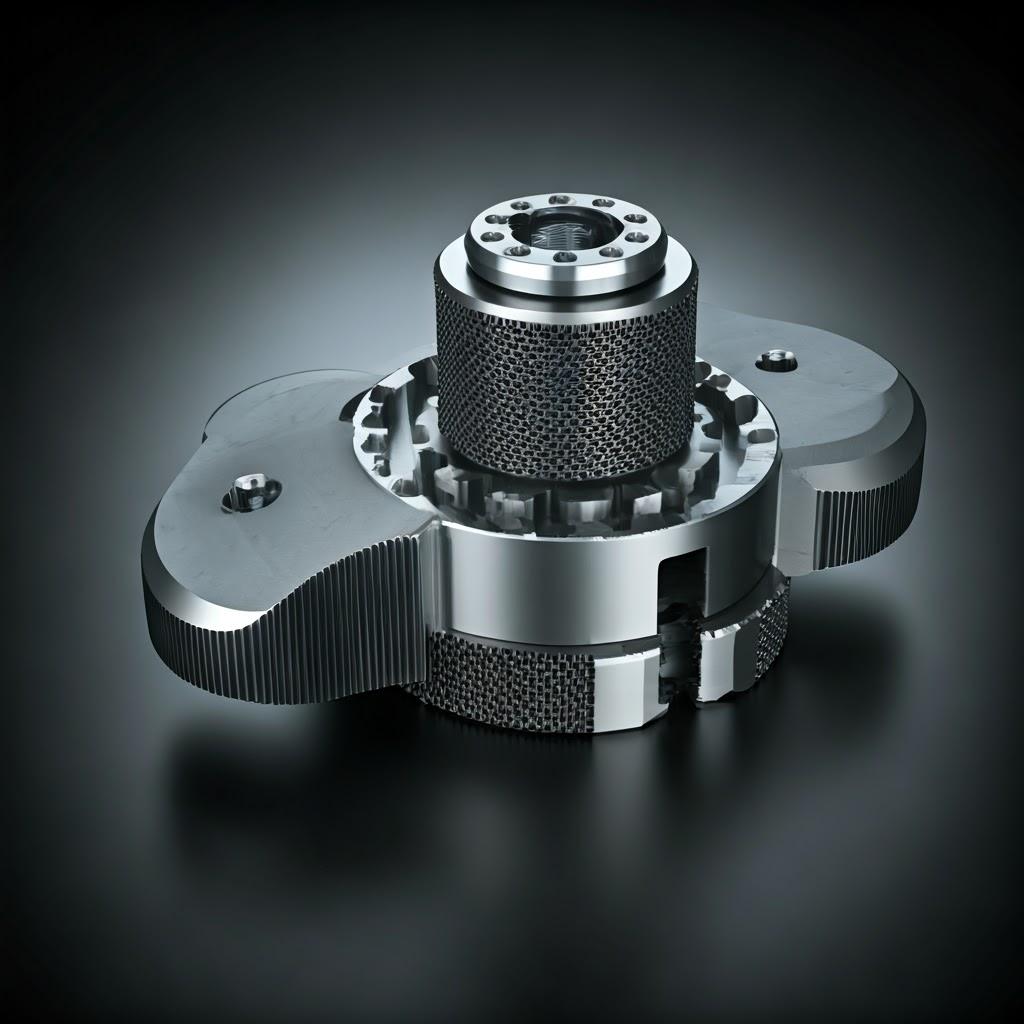
Overcoming Hurdles: Common Challenges in Metal 3D Printed Tooling and Solutions
While metal additive manufacturing offers transformative potential for custom tooling, it’s not without its challenges. Like any advanced manufacturing process, achieving consistent, high-quality results requires careful control, process understanding, and troubleshooting expertise. Engineers and procurement managers should be aware of potential hurdles to set realistic expectations and work proactively with their AM partners to mitigate risks. Addressing these challenges head-on is key to successfully implementing metal AM for demanding tooling applications. Fortunately, ongoing advancements in technology, materials, software, and process knowledge provide effective solutions and mitigation strategies.
Common Challenges and Mitigation Strategies:
- Residual Stress, Distortion, and Warpage:
- Výzva: The rapid, localized heating and cooling inherent in AM builds internal stresses. If these stresses exceed the material’s yield strength or are unevenly distributed, they can cause the part to warp, distort its shape (especially after removal from the build plate), or even crack. This is particularly relevant for large parts or complex geometries common in tooling. Mitigating tooling warpage je prvořadým zájmem.
- Causes: High thermal gradients, rapid cooling rates, material shrinkage characteristics, part geometry (e.g., large flat sections, sharp transitions).
- Solutions & Mitigation:
- Optimized Build Strategy: Careful selection of build orientation, appropriate support structures (acting as heat sinks and anchors), and optimized scan strategies (e.g., island scanning, rotating scan vectors) can help manage heat buildup and distribution.
- Process Parameter Control: Fine-tuning laser/electron beam power, scan speed, and layer thickness for the specific material and geometry. Using pre-heating (common in SEBM and some LPBF systems) reduces thermal gradients.
- Thermal Simulation: Software can predict stress accumulation and distortion, allowing for design adjustments or compensated geometries before printing.
- Mandatory Stress Relief: Performing a proper stress relief heat treatment cycle immediately after printing, often while still on the build plate, is crucial to relax internal stresses before further processing.
- Robust Part Design (DfAM): Avoiding large, unsupported flat areas, using gradual thickness transitions, and designing for inherent stiffness can help resist warping.
- Odstranění podpůrné konstrukce:
- Výzva: Removing support structures, especially internal or intricate ones, can be time-consuming, costly, and risk damaging the part surface. Support removal challenges are a significant factor in overall cost and lead time.
- Causes: Dense supports, poor accessibility, strong bonding between support and part, delicate part features.
- Solutions & Mitigation:
- DfAM for Support Minimization: Design parts with self-supporting angles (>45°) where possible. Orient the part to reduce the need for critical surface supports.
- Optimized Support Design: Use support types (e.g., lattice, tree, conical) that minimize contact points and material usage while providing necessary stability. Utilize software features that optimize support placement and type.
- Designing for Access: Ensure tools (manual or machine) can reach the supports for removal. Consider adding temporary access holes or windows if internal supports are unavoidable.
- Material Choice: Some materials are inherently easier or harder to remove supports from.
- Techniky následného zpracování: Employing wire EDM or specialized machining techniques for difficult supports.
- Pórovitost:
- Výzva: The presence of small voids or pores within the printed metal. Porosity can act as stress concentrators, reducing the tool’s strength, fatigue life, and potentially affecting thermal conductivity or surface finish after polishing. Detecting and controlling porosity in 3D printing is vital for critical tooling.
- Causes: Trapped gas during atomization or printing, vaporization of volatile elements, insufficient energy density (lack of fusion between layers/tracks), keyholing (instability caused by excessive energy density), poor powder quality or handling (moisture, contamination).
- Solutions & Mitigation:
- Optimized Process Parameters: Precise control over laser/beam power, scan speed, hatch spacing, and layer thickness is key to achieving full melting and fusion. Parameter development is crucial for each material/machine combination.
- High-Quality Powder: Using powder with low gas content, controlled particle size distribution, and high sphericity (like those produced via advanced atomization at Met3dp) is essential. Proper powder handling and storage procedures (e.g., drying, sieving) prevent contamination and moisture pickup.
- Controlled Build Atmosphere: Maintaining a high-purity inert gas atmosphere (Argon or Nitrogen) in the build chamber minimizes oxidation and contamination during printing.
- Izostatické lisování za tepla (HIP): A post-processing step involving high pressure and temperature. HIP can effectively close internal pores, significantly improving density and mechanical properties. Often used for critical applications like aerospace components, but adds cost and lead time. May be considered for highly demanding tooling.
- Quality Control (CT Scanning): Using CT scans to non-destructively inspect parts for internal porosity.
- Surface Finish and Defects:
- Výzva: As-built surfaces can be rough and may exhibit defects like partially melted powder particles (“balling”), dross formation, or witness marks from supports. These affect friction, wear, release properties (molds), and aesthetics.
- Causes: Process instabilities, suboptimal parameters, support structure interaction, powder spatter.
- Solutions & Mitigation:
- Parameter Optimization: Fine-tuning parameters to ensure stable melt pools and smooth layer deposition.
- Optimized Orientation & Supports: Positioning critical surfaces optimally and using well-designed supports.
- Následné zpracování: Employing appropriate finishing techniques (blasting, tumbling, machining, polishing) to achieve the required surface quality. See the previous section on Post-Processing.
- Cracking:
- Výzva: Cracks can form during printing or subsequent heat treatment, particularly in high-carbon or crack-sensitive alloys, or due to high residual stresses.
- Causes: High residual stress, brittle material phases, rapid cooling, material incompatibility (in multi-material prints), existing defects acting as initiation sites.
- Solutions & Mitigation:
- Výběr materiálu: Choosing alloys with better printability and lower crack susceptibility where possible (e.g., maraging steels often exhibit lower cracking risk than some tool steels during printing).
- Řízení procesu: Using pre-heating, optimized scan strategies to manage thermal gradients, and careful parameter selection.
- Úleva od stresu: Prompt and proper stress relief is critical.
- Heat Treatment Control: Careful ramping and cooling rates during post-print heat treatments, especially for sensitive materials like H13.
Successfully navigating these challenges requires a deep understanding of materials science, AM process physics, DfAM principles, and robust quality control procedures. Partnering with an experienced metal AM process optimization expert like Met3dp, who combines high-quality materials, advanced printing technology (including SEBM), and comprehensive post-processing capabilities, significantly mitigates these risks. Open communication between the customer and the AM provider regarding application requirements and potential challenges is key to achieving successful outcomes in metal 3D printed tooling.
Choosing Your Partner: Selecting the Ideal Metal 3D Printing Service Provider for Tooling
Successfully leveraging metal additive manufacturing for custom tooling goes beyond understanding the technology; it requires collaborating with the right manufacturing partner. The quality, performance, cost-effectiveness, and timely delivery of your 3D printed tools depend heavily on the capabilities, expertise, and processes of your chosen service provider. For engineers and procurement managers tasked with sourcing metal 3D printing service B2B solutions, evaluating potential suppliers requires a thorough assessment across several critical dimensions. Making an informed decision ensures access to not just printing capacity, but also the crucial technical support and quality assurance needed for demanding tooling applications.
When evaluating potential custom tooling supplier evaluation criteria, consider the following factors:
1. Technical Expertise and Tooling Application Experience:
- Deep AM Knowledge: Does the provider possess fundamental knowledge of metallurgy, AM process physics (LPBF, SEBM, etc.), and materials science relevant to tooling?
- Tooling Specialization: Have they successfully produced tooling similar to your application (e.g., injection molds with conformal cooling, high-precision fixtures, stamping dies)? Can they provide case studies or examples? Experience matters significantly in anticipating challenges and optimizing outcomes for specific tool types.
- DfAM Support: Do they offer Design for Additive Manufacturing consultation? Can they assist your engineers in optimizing existing designs or developing new ones specifically for AM to maximize benefits like conformal cooling or lightweighting? This collaborative capability adds significant value.
- Problem-Solving Skills: How do they handle potential issues like print failures, distortion control, or achieving specific material properties?
2. Machine Capabilities and Technology:
- Appropriate Technology: Do they operate the right type of AM technology (e.g., Laser Powder Bed Fusion – LPBF, Selective Electron Beam Melting – SEBM) for your material and application needs? SEBM, for instance, often used by providers like Met3dp, excels with reactive materials or applications requiring high productivity and reduced residual stress due to its vacuum environment and higher processing temperatures.
- Objem sestavení: Can their machines accommodate the size of your required tooling?
- Machine Quality and Maintenance: Are their machines from reputable manufacturers? Do they have rigorous maintenance schedules to ensure consistent performance and accuracy?
- Process Monitoring: Do their machines incorporate in-situ monitoring capabilities to track build consistency and potentially detect defects during the print?
3. Material Portfolio and Quality Control:
- Relevant Materials: Do they offer the specific tool steels (H13, M300/1.2709) or other alloys required for your application?
- Kvalita prášku: This is paramount. Inquire about their powder sourcing and quality control procedures. Do they manage powder testing, handling, storage, and traceability rigorously? Companies like Met3dp, which manufacture their own high-quality powders using advanced Gas Atomization and PREP technologies, offer a distinct advantage in material consistency and optimization. They ensure high sphericity, controlled particle size distribution, good flowability, and low impurity levels critical for dense, high-performance tooling.
- New Material Development: Are they engaged in researching or offering advanced or specialized alloys (like Met3dp’s TiNi, TiTa, TiAl, TiNbZr, CoCrMo) that might offer unique benefits for future tooling needs?
4. Comprehensive Post-Processing Capabilities:
- In-House vs. Outsourced: Does the provider handle critical post-processing steps like stress relief, heat treatment (with calibrated furnaces and expertise in specific cycles for tool steels), CNC machining, and surface finishing in-house, or do they rely on external vendors? In-house capabilities generally offer better control, potentially faster turnaround, and streamlined accountability.
- Specific Expertise: Do they have demonstrated expertise in the specific post-processing required for nástroje? This includes precise heat treatment to achieve target hardness without excessive distortion, high-precision machining of critical features, and potentially advanced polishing for molds.
- Equipment: Do they possess the necessary equipment (furnaces, CNC machines, metrology tools) to handle the required post-processing steps effectively?
5. Quality Management System and Certifications:
- QMS: Do they operate under a robust Quality Management System (QMS)? Certifications like ISO 9001 are a baseline indicator of established quality processes. For industries like aerospace, AS9100 certification may be relevant.
- Traceability: Can they provide full traceability from raw material (powder batch) through printing and post-processing to the final part?
- Metrology and Inspection: What dimensional inspection capabilities do they have (CMM, 3D scanning)? Do they offer NDT options like CT scanning if required for internal integrity verification?
6. Lead Time, Capacity, and Responsiveness:
- Quoting Speed: How quickly can they provide detailed quotes based on your requirements?
- Stated Lead Times: What are their typical lead times for parts similar to yours? Understand what factors might influence this (e.g., current backlog, post-processing complexity).
- Produkční kapacita: Do they have sufficient machine capacity to handle your potential volume or meet urgent requirements?
- Communication: Are they responsive, transparent, and easy to communicate with? Good project management and communication are essential.
7. Cost Structure and Transparency:
- Clear Pricing: Is their pricing structure transparent? Do they break down costs associated with printing, material, supports, and post-processing?
- Value Proposition: Evaluate cost not just in isolation but in the context of quality, expertise, lead time, and the overall value provided (e.g., DfAM support, integrated post-processing). Sometimes the cheapest option is not the best value, especially for critical tooling.
8. Location and Logistics:
- Doprava: Consider shipping costs and times, especially for international suppliers. Understand customs and import/export requirements if applicable. Met3dp, headquartered in Qingdao, China, serves a global market, leveraging efficient logistics.
- Site Visits/Audits: Is it possible to visit their facility or conduct an audit (even virtually) to assess their operations firsthand?
Met3dp as Your Partner: Met3dp stands out as a leading provider by offering a comprehensive, integrated solution. With decades of collective expertise, we combine industry-leading SEBM printers known for accuracy and reliability, advanced metal powder production capabilities ensuring material quality, a wide portfolio including standard and innovative alloys, and robust application development services. Our commitment extends from initial consultation and DfAM support through meticulous printing and post-processing, ensuring you receive tooling optimized for performance and reliability. To learn more about our company and values, please visit our O nás strana.
Choosing the right partner is a strategic decision. By carefully evaluating these criteria, you can identify a metal 3D printing service B2B provider that possesses the technical prowess, quality commitment, and comprehensive capabilities required to successfully deliver high-performance custom tooling for your manufacturing needs.
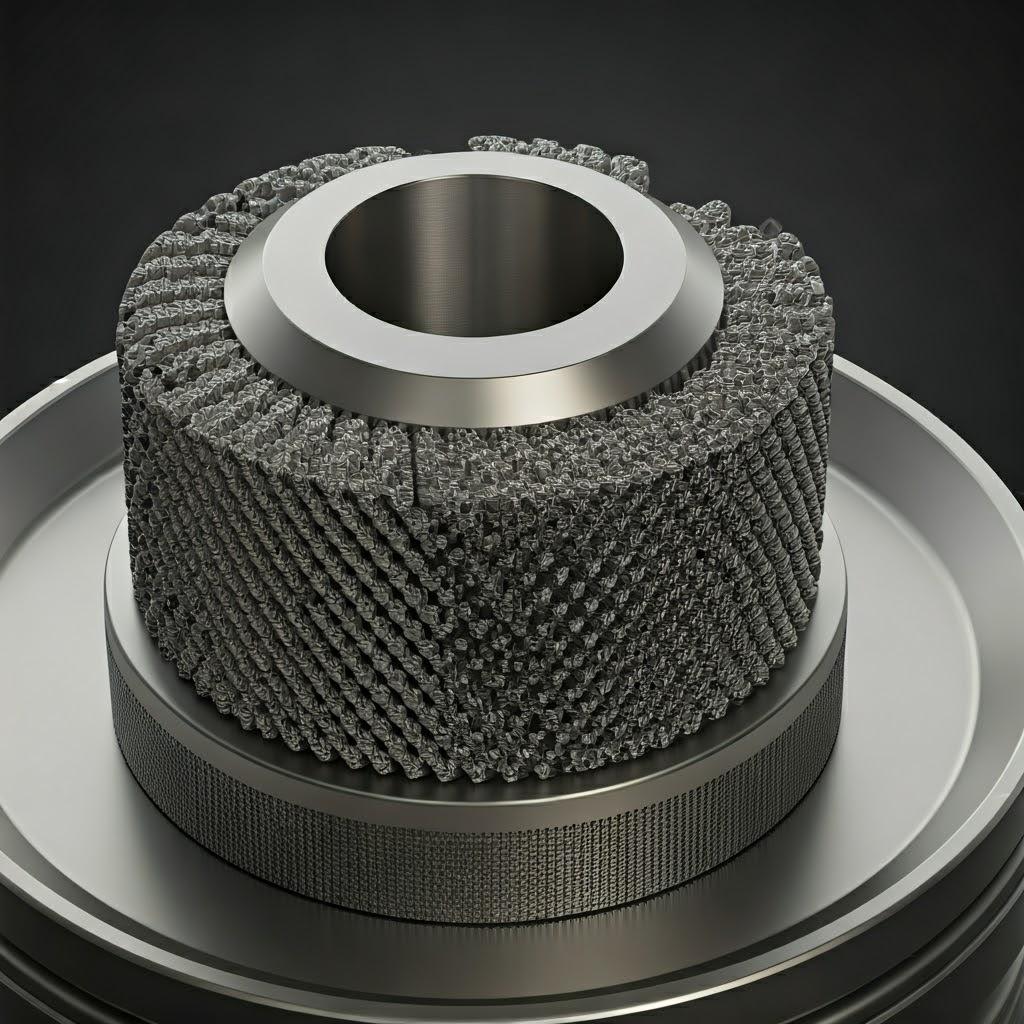
Cost Analysis and Turnaround: Factors Influencing Price and Lead Time for 3D Printed Tooling
One of the most pressing questions for engineers and procurement managers considering metal AM for custom tooling revolves around cost and lead time. While often promoted for speed, understanding the nuances of what drives the 3D printing cost estimation tooling a additive manufacturing lead time is crucial for accurate project planning, budgeting, and comparing AM against traditional methods or other suppliers. Pricing is not arbitrary; it’s influenced by a combination of material consumption, machine time, labor, and the complexity of both printing and post-processing. Similarly, lead time is more than just the print duration; it encompasses the entire workflow from order placement to final delivery.
Key Factors Influencing Cost:
- Material Type and Consumption:
- Powder Cost: The raw material cost varies significantly between different metal powders. Maraging steels or specialized alloys are typically more expensive than standard tool steels like H13 or stainless steels.
- Part Volume: The actual volume of the final part directly impacts the amount of powder consumed.
- Podpůrné struktury: Material used for support structures also contributes to cost. Optimized designs (DfAM) that minimize supports can lead to savings.
- Waste: While AM is additive, some powder waste occurs during handling, printing, and recycling processes.
- Part Geometry, Size, and Complexity:
- Bounding Box / Build Height: Larger parts or taller parts occupy the machine for longer periods and consume more material. The overall size relative to the machine’s build volume impacts how many parts can be nested together in one build, affecting efficiency.
- Složitost: While AM handles complexity well, highly intricate designs might require more extensive support structures, potentially increasing print time and post-processing labor for removal. Features like complex internal conformal cooling channels add design and verification time.
- Wall Thickness/Density: Thicker sections take longer to print. Designs utilizing lightweight lattice structures can reduce material consumption and print time compared to solid bodies.
- Machine Time (Printing):
- Hourly Rate: AM machines represent significant capital investment, and providers typically factor in an hourly operational rate covering depreciation, energy, maintenance, inert gas, and consumables.
- Print Duration: Determined by the part’s volume, height, complexity (number of contours to scan), chosen layer thickness, and scan strategy. Longer print times directly increase cost.
- Požadavky na následné zpracování: This is often a major cost driver for tooling.
- Stress Relief & Heat Treatment: Furnace time, energy consumption, and labor. Complex cycles for tool steels are more involved than simple aging for maraging steels.
- Odstranění podpory: Labor-intensive, especially for complex parts or difficult-to-access supports. Use of specialized methods like wire EDM adds cost.
- CNC Machining: Required for critical tolerances and finishes. Cost depends on the amount of machining, complexity of setups, and desired precision. Machining hardened tool steel is slower and more costly.
- Povrchová úprava: Blasting is relatively inexpensive, but manual polishing for high-quality mold finishes can be very time-consuming and requires skilled labor, significantly adding to the cost. Coating adds further expense.
- Quality Assurance and Inspection:
- Basic QA: Standard dimensional checks and visual inspection are typically included.
- Advanced NDT: Requirements like CT scanning for internal porosity detection or detailed CMM reports for dimensional verification add significant cost due to equipment and expertise required.
- certifikace: Projects requiring specific certifications or extensive documentation may incur additional administrative costs.
- Práce a odbornost:
- DfAM & Engineering Support: Consultation and design optimization services may be costed separately or bundled.
- Machine Setup & Operation: Skilled technicians are required to prepare builds, operate machines, and perform initial part cleanup.
- Post-Processing Labor: As mentioned, support removal, machining, and polishing require skilled labor.
- Order Volume and Urgency:
- Množství: While setup costs are relatively low compared to traditional tooling, some economies of scale may be possible for larger batches (e.g., multiple identical mold inserts) through optimized build nesting and streamlined post-processing. Enquire about wholesale 3D printing tooling options if applicable.
- Expedited Service: Rush orders that require interrupting schedules or dedicated machine time will typically incur premium charges.
Understanding Lead Time Components:
Lead time is the total duration from order confirmation to final part delivery. It includes:
- Quoting & Order Processing: Reviewing design, confirming requirements, generating quote (hours to days).
- Queue Time: Waiting for machine availability (can vary significantly based on provider’s backlog – potentially days to weeks).
- Build Preparation: Slicing the model, planning supports, preparing the machine (hours).
- Doba tisku: Actual machine runtime (hours to several days, depending on part size/complexity).
- Cooling Time: Allowing the build chamber and part to cool sufficiently before removal (hours).
- Následné zpracování:
- Stress Relief/Heat Treatment: Furnace cycles (hours to days, including ramp/soak/cool).
- Support Removal & Basic Finishing: (hours to days).
- Machining: (hours to days, depending on complexity and shop scheduling).
- Polishing/Coating: (hours to days or even weeks for complex molds).
- Kontrola kvality: (hours to days).
- Doprava: (days, depending on location and method).
Typical Turnaround: For moderately complex metal AM tooling requiring standard post-processing (stress relief, heat treatment, basic machining), lead times often range from 1 to 4 weeks. However, highly complex tools, those requiring extensive polishing (like injection molds), or orders placed during peak capacity periods can extend beyond this. Always confirm lead times with your provider based on your specific project. Exploring the diverse applications and processes within 3D tisk z kovu can provide further context on timelines.
By understanding the factors influencing metal AM pricing factors and the various stages contributing to the overall additive manufacturing lead time, manufacturers can better plan projects, manage budgets, and set realistic expectations when incorporating 3D printed custom tooling into their production workflows. Transparent communication with your AM partner is key to navigating these aspects successfully.
Frequently Asked Questions (FAQ) about Metal 3D Printed Custom Tooling
As metal additive manufacturing gains traction for tooling applications, engineers, designers, and procurement specialists often have questions about its capabilities, limitations, and practical implications. Here are answers to some frequently asked questions regarding 3D printed tooling FAQ:
1. How does the durability and lifespan of 3D printed tooling compare to traditionally manufactured tooling?
This is a critical question, and the answer depends heavily on several factors: material selection, print quality (density), design optimization (DfAM), and proper post-processing (especially heat treatment).
- Material Equivalence: When using equivalent materials (e.g., H13 tool steel printed vs. wrought H13) and achieving high density (>99.5%) with proper heat treatment, the core mechanical properties (hardness, strength) of the AM tool can be comparable, and sometimes even slightly better due to the fine-grained microstructure often achieved in AM.
- Performance Enhancements: Metal AM offers unique advantages that can extend tool life beyond traditional capabilities. The most significant is conformal cooling in molds and dies. By enabling more efficient and uniform heat extraction, conformal cooling reduces thermal stress, minimizes wear associated with high temperatures, and can lead to significantly longer tool life in demanding cycle applications.
- Potential Challenges: If the AM process is not properly controlled, internal defects like porosity or lack of fusion can act as initiation points for cracks, potentially reducing fatigue life compared to defect-free wrought material. Residual stresses, if not adequately relieved, can also negatively impact durability. Surface finish might also influence wear characteristics if not appropriately post-processed for the application.
- Závěr: Properly designed, printed (high density), and post-processed (correct heat treatment and finishing) metal AM tooling, especially when leveraging unique AM advantages like conformal cooling, can meet or even exceed the lifespan of traditional tooling in many applications. However, achieving this requires expertise and rigorous process control from the AM provider. It’s less about the process itself and more about achieving the required material integrity and final properties. Consulting metal AM tooling questions with experienced providers like Met3dp is recommended.
2. Is metal 3D printing cost-effective for high-volume tooling production?
Generally, metal AM finds its sweet spot in applications where its unique advantages outweigh potentially higher per-part printing costs compared to highly optimized traditional methods for very simple, high-volume tools.
- AM Strengths: Metal 3D printing is typically most cost-effective for:
- Prototypes and Bridge Tooling: Quickly producing functional tools for testing or initial low-volume production runs while conventional hard tooling is made.
- Složité geometrie: Tools with intricate features that are very difficult or expensive to machine traditionally. AM complexity comes at little to no extra cost.
- Conformal Cooling: The performance benefits (cycle time reduction, quality improvement) often justify the investment in AM for molds and dies, even for moderate volumes, based on Total Cost of Ownership (TCO).
- Custom/Low-Volume Tooling: Jigs, fixtures, or specialized tools needed in small quantities where traditional setup costs are prohibitive.
- Tool Repair/Modification: AM can sometimes be used to repair worn or damaged tools or add features.
- Traditional Strengths: For simple tooling geometries required in very high volumes (e.g., thousands of identical simple inserts), traditional methods like CNC machining from stock material may still offer a lower piece price due to established infrastructure and economies of scale.
- Evolving Landscape: As AM technology matures, printing speeds increase, machine costs decrease, and automation improves, the cost-effectiveness of AM is continually improving and expanding into higher volume applications.
- Závěr: While not typically the go-to for extremely high-volume, simple tooling based purely on initial piece price, metal AM offers compelling cost-effectiveness for prototypes, complex designs, performance-critical tools (conformal cooling), and low-to-mid volume production when considering TCO, speed-to-market, and design freedom. Evaluate the cost of 3D printed molds or fixtures based on the specific application’s value drivers.
3. What information do I need to provide to get an accurate quote for a 3D printed tool?
To receive a timely and accurate quote from a metal AM service provider like Met3dp, providing comprehensive information upfront is essential. Missing details can lead to delays or inaccurate pricing. Key information includes:
- CAD Model: A 3D CAD model in a standard neutral format (e.g., STEP (.stp/.step), Parasolid (.x_t)) is mandatory. Avoid mesh files (like STL) if possible for initial quoting unless specifically requested, as solid models contain more precise geometric information.
- Material Specification: Clearly state the desired metal alloy (e.g., H13 Tool Steel, Maraging Steel M300/1.2709, Stainless Steel 17-4PH). If unsure, describe the application requirements (hardness, temperature resistance, wear needs) so the provider can recommend a suitable material.
- Critical Dimensions and Tolerances: Clearly indicate (e.g., on a 2D drawing accompanying the 3D model) any critical dimensions and their required tolerances. Specify if these tolerances are as-built or require post-machining.
- Požadavky na povrchovou úpravu: Specify the required surface roughness (e.g., Ra value) for critical surfaces (e.g., mold cavity, fixture contact points) and non-critical surfaces. Indicate if specific finishing processes like polishing or blasting are needed.
- Požadavky na následné zpracování: Detail any mandatory heat treatment (including target hardness range, e.g., HRC 48-52 for H13), stress relief cycles, or specific machining operations needed beyond achieving tolerances (e.g., threading, final grinding).
- Application Description: Briefly explain the tool’s function (e.g., injection mold insert for ABS plastic, assembly fixture for automotive component, stamping die for sheet aluminum) and operating conditions (temperature, load, expected lifetime). This context helps the provider understand the requirements better.
- Množství: Specify the number of identical or similar parts required.
- Lead Time Requirements: Indicate if there are specific delivery deadlines.
- Confidentiality: If the design is sensitive, ensure an NDA (Non-Disclosure Agreement) is in place before sharing files.
Providing this level of detail enables the AM service provider to accurately assess the printability, complexity, material consumption, required post-processing, and associated costs and lead times, resulting in a reliable quote for your custom tooling durability and manufacturing needs.
Conclusion: The Future of Efficient Manufacturing is Forged with 3D Printed Custom Tooling
The landscape of custom tooling production is undergoing a significant transformation, driven by the capabilities of metal additive manufacturing. As we’ve explored, moving beyond the limitations of traditional subtractive methods, metal 3D printing offers a powerful combination of speed, geometric complexity, and performance enhancement that directly addresses critical manufacturing challenges. From rapidly producing intricate jigs and fixtures to creating high-performance injection molds with integrated conformal cooling channels... benefits of 3D printed tooling are tangible and impactful across diverse industries like aerospace, automotive, medical, and general manufacturing.
The ability to slash lead times from months to weeks or days accelerates product development cycles and enables unprecedented agility in responding to market demands. The design freedom inherent in AM allows engineers to create tools optimized for function – lightweight, ergonomic, and featuring complex internal structures like conformal cooling that dramatically boost productivity and final part quality. Utilizing high-performance materials like H13 tool steel and M300/1.2709 maraging steel ensures that these advantages don’t come at the expense of durability or longevity, provided that Design for Additive Manufacturing (DfAM) principles are embraced and rigorous post-processing steps, particularly heat treatment and finishing, are correctly implemented.
However, realizing the full potential of this technology requires navigating its nuances, including understanding tolerances, surface finishes, potential challenges like residual stress, and the critical importance of post-processing. Success hinges not only on the technology itself but also on choosing the right partner. An ideal metal 3D printing service B2B provider offers more than just printing capacity; they provide expertise in materials science, DfAM consultation, robust process controls, comprehensive in-house post-processing capabilities, and a strong commitment to quality assurance.
Met3dp exemplifies such a partner. As a leader in metal AM, headquartered in Qingdao, China, we provide comprehensive solutions encompassing industry-leading SEBM printers renowned for accuracy and reliability, advanced metal powders manufactured in-house using state-of-the-art atomization techniques, and deep application development expertise. We partner with organizations globally to implement 3D tisk z kovu effectively, accelerating their digital manufacturing transformations and enabling the production of next-generation tooling.
The future of efficient, agile, and high-performance manufacturing is inextricably linked with the advancements in additive manufacturing. For custom tooling, metal 3D printing is no longer a niche novelty but a strategic capability that empowers manufacturers to innovate faster, reduce costs, improve quality, and gain a significant competitive edge. By embracing this technology and collaborating with knowledgeable partners like Met3dp, companies can forge tooling solutions that unlock new levels of productivity and drive manufacturing excellence.
Ready to revolutionize your custom tooling strategy? Contact Met3dp today to explore how our cutting-edge systems, advanced metal powders, and comprehensive expertise can power your organization’s additive manufacturing goals.
Sdílet na
Facebook
Cvrlikání
LinkedIn
WhatsApp
E-mailem
MET3DP Technology Co., LTD je předním poskytovatelem řešení aditivní výroby se sídlem v Qingdao v Číně. Naše společnost se specializuje na zařízení pro 3D tisk a vysoce výkonné kovové prášky pro průmyslové aplikace.
Dotaz k získání nejlepší ceny a přizpůsobeného řešení pro vaše podnikání!
Související články
O Met3DP
Nedávná aktualizace
Náš produkt
KONTAKTUJTE NÁS
Nějaké otázky? Pošlete nám zprávu hned teď! Po obdržení vaší zprávy obsloužíme vaši žádost s celým týmem.
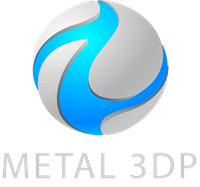
Kovové prášky pro 3D tisk a aditivní výrobu
SPOLEČNOST
PRODUKT
kontaktní informace
- Město Qingdao, Shandong, Čína
- [email protected]
- [email protected]
- +86 19116340731