Lightweight Heat Shields for Aerospace via AM
Obsah
Introduction: Revolutionizing Thermal Protection with AM Aerospace Heat Shields
The aerospace industry operates at the extremes of performance, temperature, and environmental conditions. From the blistering heat of atmospheric re-entry to the intense thermal loads experienced by engine components and hypersonic vehicles, effective thermal protection is not just a requirement – it’s a mission-critical necessity. Traditionally, manufacturing the complex geometries and robust structures needed for heat shields involved intricate, multi-step processes, often limited by subtractive machining constraints and long lead times. These methods frequently resulted in heavier components than desired, directly impacting payload capacity and fuel efficiency – key metrics in aerospace design.
Enter Additive Manufacturing (AM), commonly known as metal 3D printing. This transformative technology is rapidly reshaping the landscape of aerospace component production, particularly for thermal protection systems (TPS) like heat shields. By building parts layer by layer directly from digital designs using high-performance metal powders, AM offers unprecedented freedom in design complexity, enables significant weight reduction through optimization, and accelerates the prototyping and production cycles. For engineers and procurement managers grappling with the challenges of extreme thermal management, 3D tisk z kovu provides a powerful toolkit to create next-generation lightweight heat shields that push the boundaries of performance and efficiency.
Heat shields, fundamentally, are barriers designed to dissipate, insulate, or ablate extreme heat generated during flight, protecting sensitive structures, payloads, and personnel. Their design demands a careful balance of thermal resistance, structural integrity, low density, and durability under severe mechanical and thermal loads. Additive manufacturing excels in addressing these multifaceted requirements. It allows for the creation of integrated cooling channels, complex lattice structures for weight saving and heat dissipation, and the use of advanced materials specifically chosen for their high-temperature performance and low thermal conductivity.
Companies at the forefront of this technological shift, like Met3dp, are providing the essential building blocks for this revolution. With deep expertise in both advanced metal powder production and industrial metal AM systems, Met3dp empowers aerospace manufacturers to leverage the full potential of additive manufacturing. Their commitment to quality, reflected in their advanced gas atomization and Plasma Rotating Electrode Process (PREP) technologies for powder manufacturing, ensures the high sphericity, flowability, and purity required for mission-critical aerospace parts. As we delve deeper into the applications, advantages, and material choices for AM heat shields, it becomes clear that this technology is not just an alternative manufacturing method; it’s a key enabler for the future of aerospace thermal management, delivering lighter, more efficient, and higher-performing solutions. This shift is crucial for suppliers, distributors, and manufacturers aiming to stay competitive in the demanding aerospace B2B market.
Applications: Where are AM Heat Shields Deployed in Aerospace?
The ability of Additive Manufacturing (AM) to create complex, lightweight, and high-performance components makes it uniquely suited for producing heat shields across a diverse range of aerospace applications. The demand for advanced thermal protection solutions is ubiquitous, driven by increasing flight speeds, more ambitious space missions, and the need for reusable systems. Metal 3D tisk is finding critical use cases in the following areas:
- Atmospheric Re-entry Vehicles (Spacecraft, Capsules):
- Výzva: Protecting spacecraft and crewed capsules from the extreme temperatures (often exceeding 1650°C or 3000°F) generated by atmospheric friction during re-entry.
- AM Solution: AM allows for the creation of heat shields with optimized shapes and potentially integrated transpiration cooling channels or complex internal structures that enhance heat dissipation. Materials like Titanium alloys (e.g., Ti-6Al-4V) offer a good balance of strength-to-weight and high-temperature resistance suitable for certain re-entry profiles or specific shield components, although ceramics and ablative materials remain dominant for the highest heat flux areas. Metal AM parts can serve as structural carriers or attachment points for these primary TPS materials, benefiting from lightweighting and geometric complexity. B2B procurement managers looking for reliable suppliers of structural components for re-entry systems are increasingly turning to AM specialists.
- Hypersonic Vehicles (Missiles, Aircraft):
- Výzva: Sustained flight at Mach 5 and above generates intense, persistent aerodynamic heating on leading edges, nose cones, and engine surfaces. Materials must withstand high temperatures while maintaining structural integrity under significant mechanical stress.
- AM Solution: Metal AM enables the production of conformal heat shields and hot structure components with intricate cooling channels (using liquid or gas coolants) integrated directly into the part. This is extremely difficult or impossible with traditional manufacturing. High-temperature superalloys and potentially refractory metals (processed via specialized AM techniques) can be used. Lightweighting through topology optimization is critical for hypersonics, and AM delivers this capability. Aerospace component distributors require partners who can supply these geometrically complex, high-temperature resistant parts reliably.
- Rocket Engines and Propulsion Systems:
- Výzva: Components like nozzle extensions, combustion chambers, and exhaust vectoring systems experience extreme thermal gradients and high heat flux from combustion gases. Protecting adjacent structures and ensuring component longevity is vital.
- AM Solution: AM can produce lightweight nozzle extensions with internal stiffening features or integrated shielding elements. Heat shields for protecting sensitive engine components (actuators, electronics) can be designed with complex shapes to fit tightly within constrained spaces, often incorporating mounting features directly into the shield. Materials like Ti-6Al-4V and Nickel-based superalloys are frequently employed. The ability to consolidate parts (e.g., integrating a bracket into the shield) reduces assembly time and potential failure points, a key benefit for aerospace manufacturers.
- Satellite Thermal Management:
- Výzva: Satellites experience extreme temperature swings, facing direct solar radiation on one side and the cold vacuum of space on the other. Heat shields or thermal blankets are used to protect sensitive electronics and instruments, maintaining operational temperature ranges.
- AM Solution: While often employing multi-layer insulation (MLI), structural components and shields requiring specific shapes, rigidity, and low mass can benefit from AM. Aluminum alloys like AlSi10Mg offer excellent thermal conductivity (useful for spreading heat) and low density, making them suitable for certain thermal management structures or radiator components that function alongside traditional shielding. AM allows for custom designs tailored to specific satellite geometries and thermal requirements, valuable for wholesale buyers needing bespoke solutions.
- Engine Nacelles and Pylons (Commercial & Military Aircraft):
- Výzva: Areas around aircraft engines are exposed to significant heat radiation and require robust fire protection and thermal insulation to protect the wing structure and fuselage.
- AM Solution: Metal AM can be used to create complex brackets, heat shield segments, and ventilation components with optimized airflow for cooling. Titanium alloys are often preferred for their fire resistance and strength at elevated temperatures. The ability to rapidly prototype and produce replacement parts via AM is also a significant advantage for maintenance, repair, and overhaul (MRO) operations, impacting aerospace suppliers and distributors.
Table: AM Heat Shield Applications & Benefits
Oblast použití | Key Thermal Challenge | AM Advantages | Potential Materials | Target B2B Audience |
---|---|---|---|---|
Re-entry Vehicles | Extreme transient heating (>1650°C) | Complex structural carriers, integrated features, potential for cooling channels | Ti-6Al-4V (structures) | Space agencies, capsule manufacturers, Tier 1 suppliers |
Hypersonic Systems | Sustained high temperatures, thermal stress | Integrated cooling, complex geometries, lightweighting, rapid prototyping | Superalloys, Ti-alloys | Defense contractors, aerospace R&D, hypersonic startups |
Rocket Propulsion | High heat flux, thermal gradients | Part consolidation, conformal designs, lightweight nozzles/shields | Ti-6Al-4V, Superalloys | Launch providers, engine manufacturers, component suppliers |
Satelity | Extreme temperature swings, radiation | Lightweight structures, custom geometries, thermal conductivity (AlSi10Mg) | AlSi10Mg, Ti-alloys | Satellite manufacturers, payload integrators |
Letecké motory | Engine heat radiation, fire resistance | Complex brackets, optimized airflow, MRO replacement parts, fire resistance | Ti-6Al-4V | Aircraft OEMs, engine manufacturers, MRO providers |
Export to Sheets
The versatility of AM allows engineers to rethink heat shield design, moving away from bulky, over-engineered components towards highly optimized, integrated thermal protection solutions tailored for the specific demands of each aerospace application.
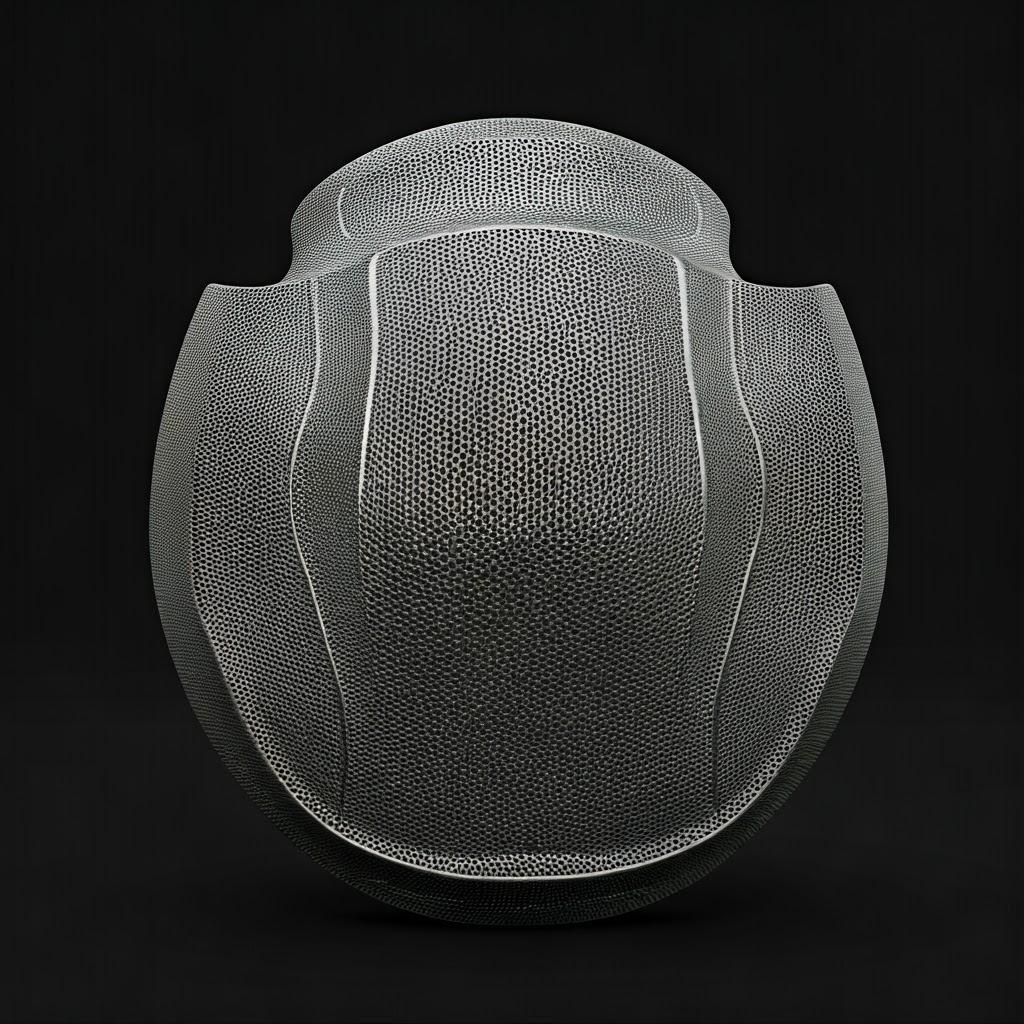
The Additive Advantage: Why Metal 3D Printing for Aerospace Heat Shields?
While traditional manufacturing methods like machining, forming, and casting have long served the aerospace industry, they often present significant limitations when fabricating complex thermal protection components like heat shields. Metal additive manufacturing offers a compelling suite of advantages that directly address these challenges, making it an increasingly preferred method for producing next-generation heat shields. For procurement managers and engineers evaluating manufacturing options, understanding these benefits is crucial for making informed decisions that impact performance, cost, and lead times.
Key advantages of using metal AM for aerospace heat shields include:
- Unparalleled Design Freedom & Complexity:
- Výzva: Traditional methods struggle with intricate internal features, undercuts, and non-uniform shapes required for optimal thermal performance and integration.
- AM Solution: AM builds parts layer-by-layer, enabling the creation of previously impossible geometries. This includes:
- Internal Cooling Channels: Conformal channels that precisely follow the contours of the heat shield surface can be integrated directly into the part, allowing for highly efficient active cooling systems.
- Mřížové struktury: Lightweight, porous structures can be designed within the shield’s volume to reduce mass significantly while maintaining structural rigidity and potentially enhancing thermal insulation or dissipation properties.
- Topology Optimization: Algorithms can determine the most efficient material distribution to withstand specific thermal and mechanical loads, removing unnecessary material and drastically reducing weight.
- B2B Impact: Aerospace suppliers can offer highly differentiated, performance-optimized heat shields that are impossible to produce conventionally, giving them a competitive edge.
- Significant Weight Reduction (Lightweighting):
- Výzva: Every kilogram saved in aerospace translates directly to fuel savings, increased payload capacity, or enhanced maneuverability. Traditional heat shields are often heavier than necessary due to manufacturing constraints.
- AM Solution: Through topology optimization, lattice structures, and the ability to create thin-walled designs with integrated stiffening ribs, AM can reduce the weight of heat shields by 20-50% or more compared to conventionally manufactured counterparts, without compromising performance. Using inherently lighter materials like Titanium (Ti-6Al-4V) and Aluminum (AlSi10Mg) further enhances this benefit.
- B2B Impact: Procurement managers seeking lightweight components to meet stringent aerospace requirements find AM an attractive solution. Weight savings cascade through the entire aircraft or spacecraft design, offering substantial system-level benefits.
- Konsolidace částí:
- Výzva: Complex assemblies involving multiple components (e.g., a shield, brackets, fasteners) increase weight, assembly time, cost, and potential points of failure.
- AM Solution: AM allows multiple functional elements to be combined into a single, monolithic printed part. A heat shield can be printed with integrated mounting bosses, attachment features, or stiffening elements, eliminating the need for separate components and fasteners.
- B2B Impact: Reduces supply chain complexity for buyers, lowers assembly costs for manufacturers, and improves overall component reliability by minimizing joints and interfaces.
- Accelerated Prototyping and Development Cycles:
- Výzva: Creating prototypes and iterating on designs using traditional tooling and machining can be time-consuming and expensive.
- AM Solution: AM enables rapid production of functional prototypes directly from CAD data, often within days. This allows engineers to test different designs, materials, and configurations quickly, significantly shortening the development timeline for new heat shield concepts.
- B2B Impact: Faster time-to-market for new aerospace systems and quicker qualification of components. Suppliers can respond more rapidly to customer design changes.
- Efektivní využití materiálu a snížení množství odpadu:
- Výzva: Subtractive manufacturing (machining) starts with a solid block of material and removes large amounts, generating significant waste (scrap), especially with expensive aerospace alloys like Titanium.
- AM Solution: Additive manufacturing is an inherently near-net-shape process, using only the material necessary to build the part and required supports. While some support material is used and some post-processing may involve minor material removal, the overall material waste is drastically reduced compared to subtractive methods. Companies like Met3dp, specializing in high-quality powder production, also focus on powder recyclability within the AM process, further enhancing sustainability.
- B2B Impact: Lower material costs, especially for high-value alloys, and improved environmental credentials appeal to cost-conscious and sustainability-focused procurement teams.
- Customization and On-Demand Production:
- Výzva: Producing small batches or highly customized heat shields using traditional methods often involves high setup costs and long lead times.
- AM Solution: AM is well-suited for low-to-medium volume production runs and creating bespoke designs without the need for expensive tooling. This facilitates on-demand manufacturing and the production of replacement parts for legacy systems.
- B2B Impact: Enables aerospace component distributors and MRO providers to offer customized solutions and maintain inventories of critical spare parts more effectively.
Table: Traditional vs. AM for Heat Shield Manufacturing
Vlastnosti | Traditional Manufacturing (Machining, Forming) | Výroba aditiv kovů (AM) | Advantage for AM Heat Shields |
---|---|---|---|
Složitost návrhu | Limited by tool access, draft angles | High geometric freedom, internal channels, lattices | Optimal thermal performance, integrated features |
Hmotnost | Often heavier due to constraints | Significant potential for lightweighting | Improved fuel efficiency, payload capacity |
Part Count | Often requires assemblies | Enables part consolidation | Reduced assembly time, cost, and failure points |
Vytváření prototypů | Slow, requires tooling | Rapid prototyping directly from CAD | Faster design iteration and time-to-market |
Materiálový odpad | Vysoká (subtraktivní proces) | Low (near-net-shape process) | Lower material costs, more sustainable |
Nástroje | Required (molds, dies, fixtures) | Tooling-free production | Cost-effective for low volumes and customization |
Doba realizace | Can be long, especially for complex parts | Potentially shorter, particularly for prototypes/low vol | Faster component delivery, agile manufacturing |
Export to Sheets
By leveraging these advantages, metal AM empowers aerospace engineers and manufacturers to overcome the limitations of conventional methods, paving the way for lighter, more complex, and higher-performing heat shields critical for current and future aerospace missions. Choosing a knowledgeable AM partner like Met3dp ensures access to not only the tiskových metod but also the material science expertise required to succeed.
Material Selection: Optimizing Performance with Ti-6Al-4V and AlSi10Mg Powders
Selecting the right material is paramount in designing aerospace heat shields, as the material’s properties directly dictate the component’s performance under extreme thermal and mechanical loads. Additive manufacturing expands the palette of usable materials and allows for their strategic deployment in complex geometries. For many lightweight heat shield applications produced via AM, two metal powders stand out: Titanium alloy Ti-6Al-4V and Aluminum alloy AlSi10Mg. The choice between them (or potentially other advanced alloys) depends heavily on the specific operating conditions, structural requirements, and weight targets.
High-quality metal powders are the foundation of reliable metal AM. Companies like Met3dp, utilizing advanced manufacturing techniques like gas atomization and PREP, produce spherical metal powders with high purity, controlled particle size distribution (PSD), and excellent flowability. These characteristics are crucial for achieving dense, defect-free parts with predictable mechanical properties – a non-negotiable requirement for aerospace components.
1. Titanium Alloy Ti-6Al-4V (Grade 5): The Aerospace Workhorse
- Popis: Ti-6Al-4V is an alpha-beta titanium alloy renowned for its excellent combination of high strength-to-weight ratio, corrosion resistance, and good performance at moderately elevated temperatures (up to around 350-400°C, potentially higher for short durations). It is one of the most widely used titanium alloys in aerospace for structural components, engine parts, and landing gear.
- Advantages for Heat Shields:
- Vysoký poměr pevnosti k hmotnosti: Significantly lighter than steel or nickel alloys for equivalent strength, crucial for lightweighting shields.
- Good High-Temperature Retention: Maintains useful strength at temperatures where aluminum alloys would fail.
- Vynikající odolnost proti korozi: Resists oxidation and attack from aerospace fluids and environments.
- Low Thermal Conductivity: Compared to aluminum, its lower conductivity can be advantageous for insulation purposes, slowing heat transfer through the shield structure (though often active cooling or insulation layers are still needed for very high heat fluxes).
- Biokompatibilita: While less relevant for heat shields, its biocompatibility broadens its use in other aerospace applications (e.g., medical implants for astronauts).
- Fire Resistance: Titanium has relatively good fire-resistant properties compared to aluminum or magnesium alloys.
- AM Considerations: Ti-6Al-4V is readily processable using Laser Powder Bed Fusion (LPBF/SLM) and Electron Beam Melting (EBM) techniques. It requires careful control of the inert atmosphere (Argon) during printing to prevent oxygen pickup, which can embrittle the material. Post-processing often includes stress relief heat treatment and potentially Hot Isostatic Pressing (HIP) to close any residual internal porosity and maximize fatigue life.
- Případy použití: Structural elements of re-entry vehicle TPS, hypersonic leading edge substructures (often combined with other materials/cooling), engine nacelle components, pylon heat shields, exhaust system components.
- B2B Focus: Suppliers offering certified Ti-6Al-4V powder and printing services compliant with aerospace standards (e.g., AMS specifications) are highly sought after by OEMs and Tier 1 manufacturers.
2. Aluminum Alloy AlSi10Mg: Lightweight and Thermally Conductive
- Popis: AlSi10Mg is an aluminum alloy containing silicon and magnesium, known for its good strength, castability (which translates to good printability in AM), and excellent thermal conductivity. It is significantly lighter than titanium.
- Advantages for Heat Shields:
- Extremely Low Density: Offers maximum weight savings where high strength or high-temperature resistance is not the primary driver.
- Vysoká tepelná vodivost: Excellent for applications where the goal is to rapidly spread and dissipate heat across the shield structure, often connecting to a larger heat sink or radiator system.
- Dobrá potiskovatelnost: Processes well using LPBF, allowing for fine features and complex geometries.
- Nižší náklady: Generally less expensive than titanium alloys, both in raw material cost and potentially in printing time/energy.
- AM Considerations: Prints well, but its lower melting point requires careful parameter control. Like titanium, an inert atmosphere is needed. Post-processing typically involves stress relief and potentially a T6 heat treatment (solutionizing and artificial aging) to achieve optimal mechanical properties (strength and hardness).
- Omezení: Its primary limitation is its relatively low operating temperature ceiling (typically below 150-200°C for sustained use), restricting its application to lower-temperature heat shield scenarios or areas further from the primary heat source. It also has lower absolute strength and fatigue resistance compared to Ti-6Al-4V.
- Případy použití: Satellite thermal management components (radiators, structural supports where heat spreading is needed), housings for electronics requiring shielding and heat dissipation, certain components in cooler sections of engine nacelles, prototypes where functional geometry is key but high-temperature performance isn’t needed for initial tests.
- B2B Focus: Distributors and manufacturers looking for cost-effective, lightweight solutions for moderate temperature applications often specify AlSi10Mg. Availability of aerospace-grade powder and certified printing processes is key.
Table: Comparison of Ti-6Al-4V and AlSi10Mg for AM Heat Shields
Vlastnictví | Ti-6Al-4V (třída 5) | AlSi 10Mg | Key Implication for Heat Shields |
---|---|---|---|
Hustota | ~4,43 g/cm³ | ~2.67 g/cm³ | AlSi10Mg offers superior lightweighting potential. |
Max Operating Temp. | ~350-400°C (sustained) | ~150-200°C (sustained) | Ti-6Al-4V suitable for higher temperature environments. |
Strength (Ultimate) | High (~900-1100 MPa, depending on HT) | Moderate (~250-350 MPa, depending on HT) | Ti-6Al-4V provides much higher structural integrity. |
Poměr síly a hmotnosti | Velmi vysoká | Vysoký | Both are excellent, but Ti-6Al-4V often preferred for structure. |
Tepelná vodivost | Low (~7 W/m·K) | High (~130-150 W/m·K) | AlSi10Mg excels at heat spreading; Ti-6Al-4V provides insulation. |
Printability (LPBF) | Good (requires careful control) | Vynikající | Both are well-suited for AM complexity. |
Odolnost proti korozi | Vynikající | Good (can require surface treatment) | Ti-6Al-4V generally more robust in harsh environments. |
Relativní náklady | Vyšší | Dolní | AlSi10Mg offers a more economical solution. |
Export to Sheets
Beyond Ti-6Al-4V and AlSi10Mg:
It’s important to note that the portfolio of metal AM powders is constantly expanding. For even more demanding high-temperature applications, aerospace engineers might consider:
- Nickel-Based Superalloys (e.g., Inconel 718, Inconel 625): Offer excellent strength retention at very high temperatures (up to 700°C or higher) and good corrosion resistance. Often used in engine hot sections and exhaust systems.
- Refractory Metals (e.g., Molybdenum, Tungsten alloys): For extreme temperature applications like hypersonic leading edges or throats of rocket nozzles, though processing these materials via AM presents significant challenges.
- Advanced Titanium Alloys: Met3dp’s portfolio includes innovative alloys like TiNi, TiTa, TiAl, and TiNbZr, which may offer tailored properties for specific thermal or structural challenges beyond standard Ti-6Al-4V. Exploring the full range of produkty available from specialized suppliers is crucial.
Conclusion on Materials:
The choice between Ti-6Al-4V and AlSi10Mg for an AM heat shield hinges on a thorough analysis of the thermal environment, structural loads, weight targets, and cost constraints. Ti-6Al-4V provides the high-temperature strength needed for more demanding applications, while AlSi10Mg offers maximum weight savings and heat spreading capabilities in cooler environments. Partnering with an AM provider like Met3dp, which possesses deep material science knowledge and offers high-quality, aerospace-grade powders, ensures that the selected material translates into a reliable, high-performance heat shield component.
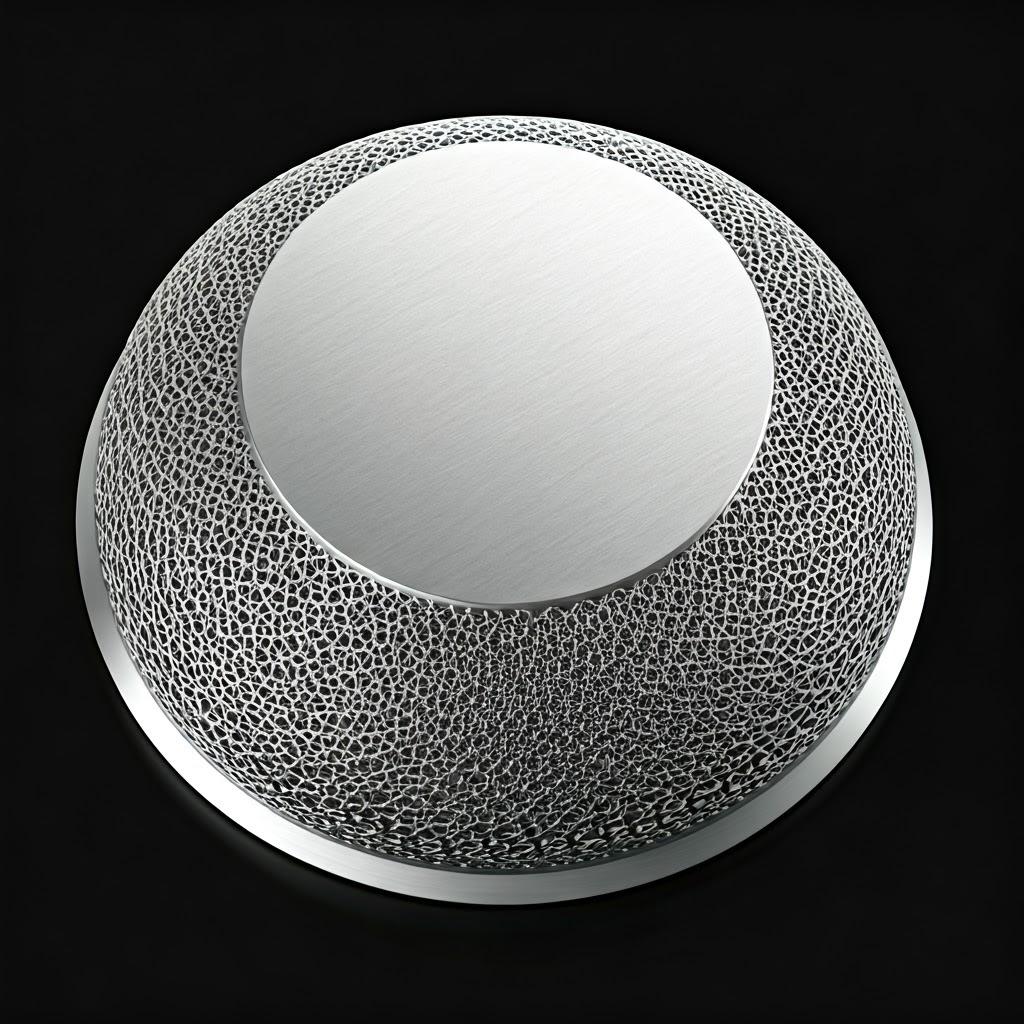
Design for Additive Manufacturing (DfAM): Optimizing Heat Shield Geometry and Performance
Simply replicating a design intended for traditional manufacturing using Additive Manufacturing rarely unlocks the full potential of the technology. To truly leverage the advantages discussed earlier – particularly lightweighting, part consolidation, and enhanced thermal performance – engineers must embrace Design pro aditivní výrobu (DfAM) principles. DfAM is a mindset and methodology focused on designing parts specifically for the layer-by-layer build process, considering both its unique capabilities and its inherent constraints. Applying DfAM is critical for developing optimized AM aerospace heat shields that meet stringent performance targets.
Key DfAM considerations for AM heat shields include:
- Topology Optimization:
- Concept: Using specialized software algorithms to remove material from areas where it is not structurally necessary to withstand defined thermal and mechanical loads, resulting in organic-looking, highly efficient structures.
- Application for Heat Shields: Dramatically reduces weight while ensuring stiffness and strength are maintained where needed. This is ideal for creating lightweight structural carriers for TPS materials or optimizing the shield’s own structural frame. The software considers load cases (thermal gradients, pressure differentials, vibration) to sculpt the part.
- Benefit: Maximizes weight savings, leading to direct improvements in fuel efficiency and payload capacity.
- Lattice and Cellular Structures:
- Concept: Incorporating internal porous structures (like honeycombs, gyroids, or stochastic foams) within the solid volume of a part. These structures can be precisely controlled in terms of cell size, strut thickness, and overall density.
- Application for Heat Shields:
- Odlehčení: Replacing solid material with porous lattices significantly reduces mass.
- Tepelný management: Certain lattice types can impede heat transfer (insulation) or, conversely, increase surface area for heat exchange if integrated with cooling channels. They can also alter vibration damping characteristics.
- Absorpce energie: Can be designed to absorb impact energy, potentially useful for shields protecting against debris.
- Benefit: Provides multi-functional benefits beyond simple weight reduction, enabling tailored thermal and mechanical responses.
- Konsolidace částí:
- Concept: Redesigning assemblies of multiple components to be printed as a single, integrated part.
- Application for Heat Shields: Combining the shield surface with mounting brackets, standoffs, stiffening ribs, or even fluid channel connectors into one monolithic piece.
- Benefit: Reduces part count, eliminates fasteners and joints (potential failure points), simplifies assembly, lowers overall weight, and potentially improves structural integrity.
- Feature Integration:
- Concept: Designing functional features directly into the AM part that would be difficult or impossible to create otherwise.
- Application for Heat Shields:
- Konformní chladicí kanály: Internal channels that precisely follow the complex curvature of the heat shield surface for highly effective, targeted cooling.
- Textured Surfaces: Creating specific surface textures during the printing process to enhance radiative properties or influence aerodynamic boundary layers.
- Integrated Sensor Housings: Designing cavities or mounting points for embedded sensors (temperature, pressure, strain) within the shield structure.
- Benefit: Enhances performance (cooling, sensing) and adds functionality without increasing part count or assembly complexity.
- Build Orientation and Support Structures:
- Concept: Considering how the part will be oriented on the build plate and where temporary support structures will be needed during the printing process (e.g., using LPBF or EBM).
- Application for Heat Shields:
- Povrchová úprava: Critical surfaces requiring high smoothness should ideally be oriented upwards or steeply angled to minimize the “stair-stepping” effect inherent in layer-based manufacturing and reduce support contact points.
- Overhangs and Angles: Shallow angles (typically less than 45 degrees from the horizontal) and large overhangs require support structures to prevent collapse during printing. DfAM aims to minimize the need for supports or design self-supporting angles where possible.
- Odstranění podpory: Supports must be removable after printing, usually through manual breaking or machining. DfAM involves designing access for support removal and minimizing supports on critical or hard-to-reach surfaces.
- Zbytkové napětí: Build orientation influences thermal gradients during printing, affecting residual stress and potential distortion. Strategic orientation can help mitigate these issues.
- Benefit: Optimizes print success, reduces post-processing time and cost, and improves final part quality.
- Minimum Feature Size and Wall Thickness:
- Concept: Understanding the limits of the specific AM process and machine regarding the smallest features (holes, slots, pins) and thinnest walls that can be reliably produced.
- Application for Heat Shields: Ensuring that thin walls designed for lightweighting or fine details like cooling channel inlets/outlets are within the achievable limits of the chosen AM system (e.g., Met3dp’s printers known for accuracy). Wall thickness also impacts heat transfer and structural stability.
- Benefit: Ensures manufacturability and prevents feature failure during printing or in service.
Table: DfAM Strategies for AM Heat Shields
DfAM Strategy | Goal | How it Applies to Heat Shields | Klíčový přínos |
---|---|---|---|
Optimalizace topologie | Maximize stiffness/strength for given mass | Remove non-essential material based on load cases | Maximum weight reduction |
Mřížové struktury | Reduce weight, tailor properties | Replace solid volumes with internal porous structures | Multi-functional: lightweighting, thermal control |
Konsolidace částí | Reduce part count and assembly | Combine shield, brackets, features into one piece | Improved reliability, lower assembly cost & weight |
Feature Integration | Add functionality | Embed cooling channels, sensor mounts, surface textures | Enhanced performance, added capabilities |
Orientation/Supports | Optimize printability, minimize post-pro. | Plan build setup, design self-supporting angles | Reduced print time/cost, better surface finish |
Feature Size Limits | Ensure manufacturability | Adhere to minimum wall thickness, hole size constraints | Guaranteed producibility, prevents feature failure |
Export to Sheets
Implementing DfAM requires close collaboration between design engineers and AM specialists. Partners like Met3dp, with comprehensive knowledge spanning materials, processes, and equipment, can provide invaluable guidance in applying these principles effectively, transforming a standard heat shield design into a highly optimized, additively manufactured component. Engaging with AM experts early in the design phase is crucial for maximizing the benefits and ensuring a successful outcome for aerospace B2B suppliers and their customers.
Precision Matters: Achieving Tight Tolerances and Surface Finish in AM Heat Shields
Aerospace components demand exceptional precision. Heat shields are no exception, requiring accurate dimensions for proper fit and assembly, controlled surface characteristics for predictable thermal and aerodynamic performance, and overall geometric integrity to ensure structural reliability. While Additive Manufacturing offers incredible geometric freedom, achieving the tight tolerances and specific surface finishes required for aerospace applications necessitates careful process control, understanding of the technology’s capabilities, and often, post-processing steps. Procurement managers and engineers must have realistic expectations and clear specifications regarding the achievable precision of AM parts.
1. Dimensional Accuracy and Tolerances:
- Definice: Dimensional accuracy refers to how closely the final printed part conforms to the nominal dimensions specified in the CAD model. Tolerance defines the permissible range of variation for a given dimension.
- AM Capabilities: The achievable accuracy in metal AM depends on several factors:
- Machine Calibration: Precision of the laser or electron beam guidance system, layer thickness consistency. Companies like Met3dp invest heavily in printers with industry-leading accuracy and reliability.
- Vlastnosti materiálu: Shrinkage rates, thermal expansion characteristics of the specific metal powder (e.g., Ti-6Al-4V vs. AlSi10Mg).
- Part Geometry & Size: Larger parts and complex geometries are generally more prone to distortion and deviation.
- Build Orientation: Affects thermal history and potential warping.
- Support Strategy: How well supports constrain the part during printing.
- Následné zpracování: Stress relief heat treatments can cause minor dimensional changes.
- Typical Tolerances: As-built tolerances for metal LPBF/EBM are often in the range of ±0.1 mm to ±0.3 mm for smaller dimensions (e.g., up to 100 mm), with potentially larger deviations (e.g., ±0.2% of the dimension) for larger parts. However, tighter tolerances can often be achieved on specific critical features through careful process optimization and are routinely improved upon with advancements in AM technology.
- Aerospace Requirements: Critical interface dimensions, mounting points, and aerodynamic surfaces often require tolerances tighter than the standard as-built capability of AM. These tighter tolerances are typically achieved through post-process machining.
2. Surface Finish (Roughness):
- Definice: Surface finish, often quantified by Ra (average roughness), describes the texture of the part’s surfaces.
- AM Characteristics: As-built surfaces in metal AM, particularly from powder bed fusion processes, are inherently rougher than machined surfaces. This is due to:
- Layer Lines: The characteristic “stair-stepping” effect, especially on angled or curved surfaces.
- Partially Melted Powder: Powder particles adhering to the surface.
- Support Structure Contact Points: Marks left after support removal.
- Typical Ra Values: As-built Ra values for metal LPBF/EBM typically range from 6 µm to 25 µm (240 µin to 1000 µin), depending on the material, parameters, orientation, and whether the surface faced up, down, or sideways during the build. Downward-facing surfaces tend to be rougher due to support contact.
- Aerospace Requirements: While some internal surfaces might tolerate as-built roughness, critical aerodynamic surfaces, sealing faces, or areas requiring specific radiative properties often demand much smoother finishes (e.g., Ra < 3.2 µm or even < 0.8 µm). These smoother finishes necessitate post-processing methods.
3. Achieving Required Precision:
- Řízení procesu: Utilizing high-end AM systems with precise control over laser/beam parameters, gas flow, and thermal management is fundamental. Met3dp’s focus on high-accuracy printers is a key enabler here.
- Design for Precision: Designing parts with tolerances appropriate for AM where possible, and clearly identifying critical dimensions and surfaces that will require post-processing. Orienting parts optimally to achieve the best possible as-built finish on critical surfaces.
- Následné zpracování: This is often essential for meeting tight aerospace specifications:
- CNC Machining: Used to achieve tight tolerances on specific features (holes, mating faces, critical diameters).
- Povrchové úpravy: Techniques like grinding, polishing, abrasive flow machining (AFM), or chemical etching can significantly improve surface finish.
- Heat Treatment (HIP): While primarily for mechanical properties, Hot Isostatic Pressing can also lead to minor improvements in surface consistency by closing near-surface pores.
Table: Precision Considerations for AM Heat Shields
Parametr | Typical As-Built AM (LPBF/EBM) | Common Aerospace Requirements | Method to Achieve Requirement (if needed) |
---|---|---|---|
Tolerance | ±0.1 to ±0.3 mm (or ±0.2%) | < ±0.1 mm (often tighter) | CNC Machining of critical features |
Surface Finish (Ra) | 6 µm – 25 µm | < 3.2 µm (or smoother) | Grinding, Polishing, AFM, Machining, Coating |
Pórovitost | < 0.5% (often <0.1%) | Near 100% dense (<0.01%) | Process Optimization, Hot Isostatic Pressing (HIP) |
Geometric Integrity | Good (subject to warping) | High (must match CAD intent) | Stress Relief, Fixturing during HT, Machining |
Export to Sheets
Conclusion on Precision:
Engineers and procurement managers must clearly define the dimensional tolerance and surface finish requirements for each heat shield feature early in the process. While metal AM provides good baseline precision, achieving the stringent demands common in aerospace often requires incorporating post-processing steps into the manufacturing plan. Partnering with an AM provider who understands these nuances, possesses high-quality equipment, and has established relationships with post-processing specialists is crucial for obtaining heat shield components that meet all specifications. Discussing these requirements upfront ensures realistic expectations regarding cost, lead time, and final part quality.
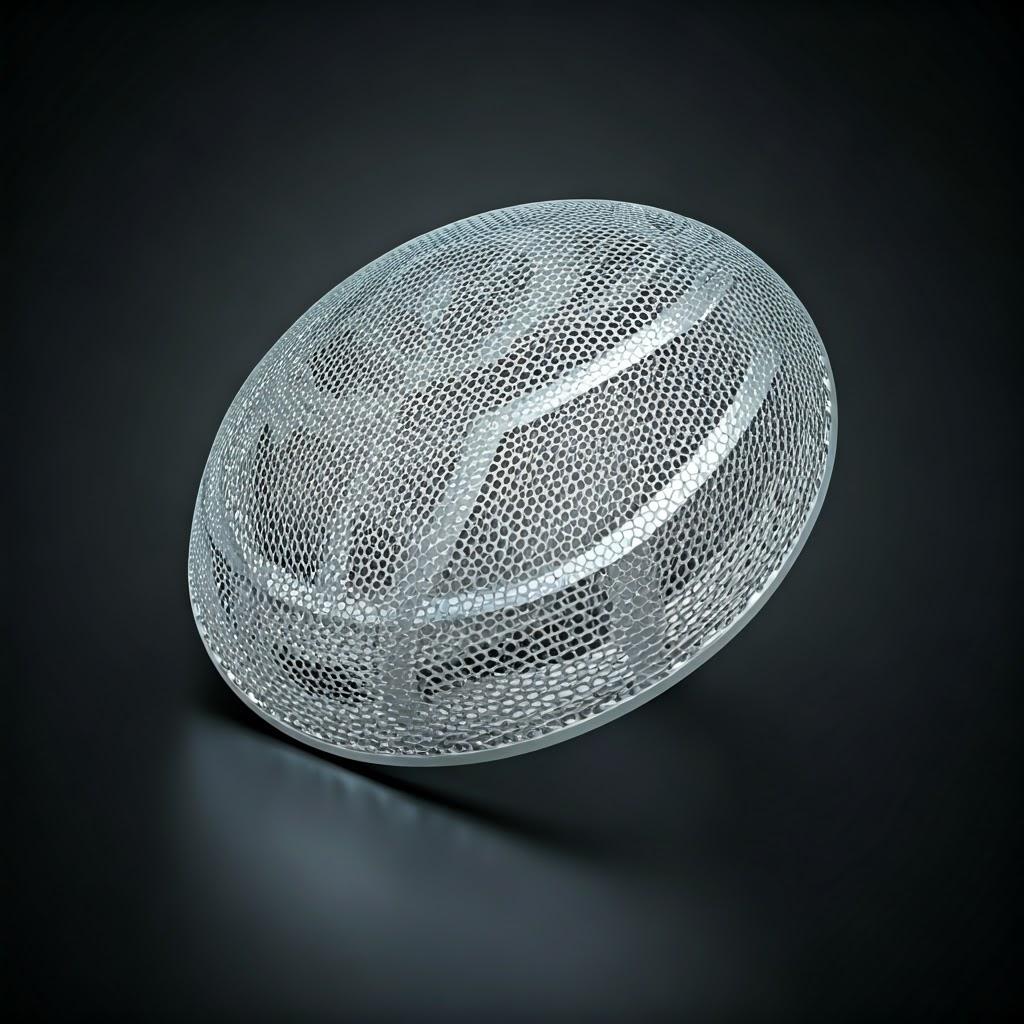
Beyond the Build: Essential Post-Processing for Aerospace Heat Shields
The journey of an additively manufactured metal heat shield doesn’t end when the printer stops. The “as-built” part, fresh off the build plate, typically requires several crucial post-processing steps to transform it into a flight-ready component meeting the rigorous demands of aerospace applications. These steps are essential for ensuring the part possesses the required mechanical properties, dimensional accuracy, surface finish, and overall integrity. Understanding these common post-processing requirements is vital for accurately estimating costs, lead times, and for selecting a capable AM supplier or managing the production workflow.
Common post-processing steps for AM aerospace heat shields include:
- Powder Removal:
- Účel: To remove any loose or partially sintered metal powder trapped within internal channels, lattice structures, or adhered to the surface.
- Metody: Manual brushing, compressed air blowing, ultrasonic cleaning baths, specialized powder recovery systems integrated with the printer. Thorough powder removal is critical, especially from internal cooling channels, to prevent blockages and ensure functionality. For complex internal geometries, this can be challenging.
- Importance: Ensures part cleanliness, prevents contamination in subsequent steps, and allows for accurate inspection.
- Stress Relief Heat Treatment:
- Účel: The rapid heating and cooling cycles inherent in layer-by-layer AM processes (especially LPBF) induce residual stresses within the part. These stresses can cause distortion, cracking, and reduced fatigue life if not relieved.
- Metody: Heating the part in a controlled furnace (often in a vacuum or inert atmosphere to prevent oxidation) to a specific temperature below the alloy’s transformation point, holding it for a set duration, and then cooling it slowly. Parameters vary significantly based on the alloy (e.g., Ti-6Al-4V vs. AlSi10Mg) and part geometry. This is often done while the part is still attached to the build plate to minimize warping.
- Importance: Stabilizes the part dimensionally, reduces the risk of cracking, and improves overall mechanical performance. Mandatory for most aerospace structural components.
- Part Removal from Build Plate:
- Účel: To separate the printed heat shield(s) from the metal build plate they were fused to during the AM process.
- Metody: Typically done using wire Electrical Discharge Machining (EDM) or a band saw. Care must be taken not to damage the part during removal.
- Importance: Frees the part for subsequent processing steps.
- Odstranění podpůrné konstrukce:
- Účel: To remove the temporary support structures required during printing to anchor the part and support overhangs.
- Metody: Supports are often designed with weakened interfaces for easier removal. Methods include manual breaking (pliers, chisels), machining (milling), or sometimes wire EDM. This step can be labor-intensive, especially for complex parts with internal supports.
- Importance: Achieves the final part geometry and reveals the underlying surface.
- Izostatické lisování za tepla (HIP):
- Účel: To eliminate any residual internal porosity (microscopic voids) that might remain after printing, thereby achieving full theoretical density. HIPing significantly improves fatigue life, fracture toughness, and overall mechanical property consistency.
- Metody: Subjecting the part to high temperature (below melting point) and high isostatic pressure (using an inert gas like Argon) simultaneously in a specialized HIP vessel.
- Importance: Often mandatory for critical aerospace components, especially those subject to fatigue loading or requiring maximum material integrity. It enhances the reliability and performance predictability of AM parts.
- Machining (CNC):
- Účel: To achieve tight tolerances on critical dimensions, mating surfaces, sealing faces, or threaded holes that cannot be met by the as-built AM process. It is also used to improve surface finish on specific features.
- Metody: Using conventional CNC milling, turning, or grinding operations. Fixturing AM parts for machining can sometimes be challenging due to their complex or topology-optimized shapes.
- Importance: Ensures precise fit and function within the larger aerospace assembly.
- Povrchová úprava:
- Účel: To achieve the required surface roughness (Ra) for aerodynamic performance, sealing, coating adhesion, or aesthetic reasons.
- Metody: A wide range of techniques can be employed, depending on the required finish and geometry:
- Tumbling/Mass Finishing: Using abrasive media in a vibrating or rotating drum for general smoothing and deburring.
- Abrasive Blasting (Sandblasting, Bead Blasting): Creates a uniform matte finish, cleans surfaces.
- Grinding/Polishing: Mechanical processes for achieving very smooth, mirror-like finishes on accessible surfaces.
- Abrasive Flow Machining (AFM): Forcing an abrasive putty through internal channels or over complex surfaces to smooth them.
- Electropolishing: An electrochemical process that removes a thin surface layer, resulting in a very smooth and clean finish (suitable for certain alloys).
- Importance: Critical for meeting performance specifications related to fluid flow, heat transfer, sealing, or reducing friction.
- Cleaning and Inspection:
- Účel: Final cleaning to remove any residues from previous steps (machining fluids, polishing compounds) and thorough inspection to verify dimensional accuracy, surface finish, and integrity.
- Metody: Aqueous or solvent cleaning, visual inspection, Coordinate Measuring Machine (CMM) for dimensional checks, non-destructive testing (NDT) methods like X-ray or CT scanning to verify internal integrity and detect defects (porosity, cracks).
- Importance: Final quality assurance step before the heat shield is certified for use.
Table: Overview of Post-Processing Steps for AM Heat Shields
Post-Processing Step | Primary Purpose | Typical Method(s) | Key Outcome |
---|---|---|---|
Odstranění prášku | Cleanliness, channel clearing | Brushing, Air-jet, Ultrasonic Cleaning | Part free of loose powder |
Úleva od stresu | Reduce internal stress, prevent distortion | Controlled Furnace Heating/Cooling | Dimensional stability, improved properties |
Part Removal | Separate part from build plate | Wire EDM, Sawing | Part ready for handling |
Odstranění podpory | Achieve final geometry | Manual Breaking, Machining, EDM | Support-free part, surface marks may remain |
HIP | Eliminate internal porosity, improve properties | High Temp & Pressure Furnace | Full density, enhanced fatigue life |
Machining (CNC) | Achieve tight tolerances, smooth surfaces | Milling, Turning, Grinding | Precise dimensions, specific feature finishing |
Povrchová úprava | Achieve required Ra, clean/prepare surfaces | Blasting, Polishing, AFM, Electropolishing | Desired surface texture and smoothness |
Cleaning & Inspection | Final QA, verify specifications | Cleaning Baths, CMM, NDT (X-ray/CT) | Certified, flight-ready component |
Export to Sheets
Procurement managers and engineers must factor these essential post-processing steps into their project planning. Working with a vertically integrated AM provider or one with a well-managed network of post-processing partners ensures a streamlined workflow and guarantees that the final AM heat shield meets all stringent aerospace requirements.
Navigating Challenges: Overcoming Hurdles in AM Heat Shield Production
While metal additive manufacturing offers significant advantages for producing lightweight and complex aerospace heat shields, the technology is not without its challenges. Successfully implementing AM requires a deep understanding of the potential pitfalls and the strategies needed to mitigate them. Awareness of these challenges allows engineers and procurement teams to work proactively with their AM suppliers to ensure robust and reliable production. Met3dp, through its focus on high-quality powders and reliable printing systems, actively works to minimize many of these common issues.
Key challenges in AM heat shield production include:
- Residual Stress, Warping, and Distortion:
- Problem: The rapid, localized heating and cooling inherent in AM processes create significant thermal gradients, leading to the buildup of internal residual stresses. These stresses can cause parts to warp or distort during or after the build, especially for large, thin, or complex geometries typical of heat shields. In severe cases, stresses can cause cracking.
- Mitigation Strategies:
- Process Simulation: Using software to predict thermal behavior and stress accumulation, allowing for optimization of build orientation and support strategies before printing.
- Optimized Scan Strategies: Using specific laser/beam scanning patterns (e.g., island scanning, sectoring) to manage heat distribution.
- Platform Heating: Maintaining an elevated temperature of the build plate and chamber environment reduces thermal gradients.
- Robust Support Structures: Strategically placed supports anchor the part securely to the build plate, resisting distortion forces.
- Stress Relief Heat Treatment: Essential post-processing step performed promptly after the build (often before part removal) to relax internal stresses.
- DfAM: Designing parts with features that inherently minimize stress concentration and warping potential.
- Porosity Control:
- Problem: Small voids or pores can form within the printed material due to trapped gas, incomplete fusion between layers, or powder contamination. Porosity degrades mechanical properties, particularly fatigue strength, which is critical for aerospace components.
- Mitigation Strategies:
- High-Quality Powder: Using highly spherical powder with low internal gas content and controlled particle size distribution, like those produced by Met3dp’s advanced atomization techniques. Proper powder handling and storage are crucial to prevent moisture pickup and contamination.
- Optimized Process Parameters: Precisely controlling laser/beam power, scan speed, layer thickness, and chamber atmosphere (inert gas purity) to ensure complete melting and fusion.
- Izostatické lisování za tepla (HIP): An effective post-processing step to close internal pores and achieve near-full density.
- Process Monitoring: In-situ monitoring systems can sometimes detect porosity formation during the build, allowing for adjustments.
- Support Structure Removal and Surface Quality:
- Problem: Support structures are necessary but can be difficult and time-consuming to remove, especially from complex internal channels or delicate lattice structures. Removal processes can leave witness marks or rough patches on the part surface, impacting finish and potentially requiring further treatment.
- Mitigation Strategies:
- DfAM for Support Minimization: Designing parts with self-supporting angles (typically >45°) and optimizing build orientation to reduce the need for supports.
- Optimized Support Design: Using support structures with minimal contact points or easily breakable interfaces. Software tools can generate optimized support geometries.
- Advanced Removal Techniques: Utilizing methods like electrochemical machining or specialized tooling for difficult-to-reach supports.
- Planning for Finishing: Anticipating that surfaces where supports were attached will likely require additional machining or finishing steps.
- Achieving Tight Tolerances and Surface Finish:
- Problem: As discussed previously, the as-built accuracy and surface finish of AM parts may not meet the stringent requirements for all heat shield features. Achieving aerospace-grade precision often necessitates additional steps.
- Mitigation Strategies:
- High-Precision AM Systems: Utilizing machines known for their accuracy and repeatability.
- Calibration and Process Control: Rigorous machine calibration and parameter optimization.
- Integrating Post-Processing: Planning for and incorporating necessary machining and surface finishing steps into the production workflow and budget. Collaboration between AM provider and machining/finishing specialists is key.
- Material Property Consistency and Qualification:
- Problem: Ensuring that the mechanical properties (strength, ductility, fatigue life) of the final AM part are consistent from build-to-build and meet aerospace specifications requires rigorous process control and validation. Qualifying new AM parts or materials for flight applications involves extensive testing and documentation.
- Mitigation Strategies:
- Standardized Procedures: Adhering to strict operating procedures for powder handling, machine operation, and post-processing.
- Certifikace materiálu: Using certified aerospace-grade powders from reputable suppliers.
- Process Monitoring & Data Logging: Tracking key parameters during the build for traceability and quality control.
- Comprehensive Testing: Performing tensile tests, fatigue tests, metallographic analysis, and NDT on witness coupons built alongside the parts and/or on the parts themselves.
- Collaboration with Certification Bodies: Working closely with regulatory agencies (FAA, EASA) and prime contractors to meet qualification requirements.
- Cost and Lead Time Management:
- Problem: While AM can accelerate prototyping, the overall cost and lead time for production parts can be influenced by factors like machine time, powder cost, labor-intensive post-processing (especially support removal and finishing), and qualification requirements.
- Mitigation Strategies:
- DfAM for Efficiency: Optimizing designs not just for performance but also for printability (e.g., minimizing supports, reducing build height).
- Nesting and Build Optimization: Printing multiple parts simultaneously on a single build plate to maximize machine utilization.
- Realistic Planning: Accurately estimating time and costs associated with all steps, including post-processing and inspection.
- Supplier Expertise: Working with experienced AM providers who can optimize the entire workflow.
Table: Common AM Challenges & Mitigation Approaches
Výzva | Primary Cause(s) | Key Mitigation Strategies | Importance for Heat Shields |
---|---|---|---|
Warping/Distortion | Residual Stress from Thermal Gradients | Simulation, Scan Strategy, Supports, Stress Relief HT, DfAM | High (Ensures proper fit/assembly) |
Pórovitost | Trapped Gas, Incomplete Fusion | High-Quality Powder, Optimized Parameters, HIP | Critical (Impacts fatigue life) |
Support Removal/Finish | Need for Supports, Removal Process | DfAM (Minimize Supports), Optimized Support Design, Advanced Removal, Plan for Finishing | High (Affects cost, surface quality) |
Tolerance/Accuracy | Process Limitations, Thermal Effects | High-Precision Machines, Calibration, Post-Process Machining | Critical (Ensures fit and function) |
Property Consistency | Process Variability, Powder Quality | Standard Procedures, Certified Powder, Testing, Monitoring, Qualification | Mandatory (Ensures reliability) |
Cost/Lead Time | Machine Time, Powder Cost, Post-Processing | DfAM for Efficiency, Build Optimization, Realistic Planning, Experienced Supplier | High (Affects project viability) |
Export to Sheets
Successfully navigating these challenges requires expertise, rigorous process control, and a collaborative approach between designers, AM specialists, and quality assurance teams. By acknowledging these potential hurdles and implementing appropriate mitigation strategies, manufacturers can confidently leverage metal AM to produce high-performance, reliable aerospace heat shields.
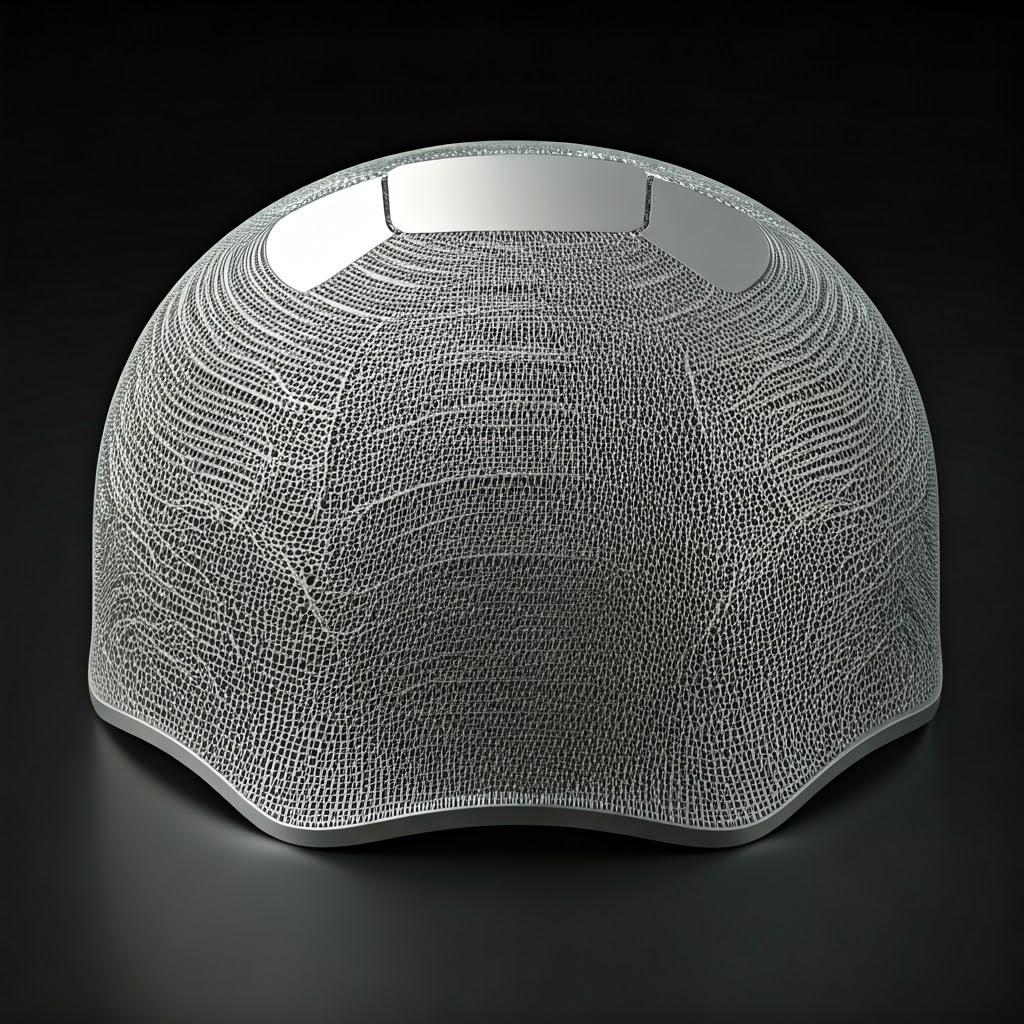
Supplier Selection: Choosing the Right Metal AM Partner for Aerospace Components
Selecting the right additive manufacturing service provider is as critical as the design and material selection for producing high-quality, flight-ready aerospace heat shields. The unique demands of the aerospace industry – stringent quality requirements, complex geometries, advanced materials, and the need for absolute reliability – mean that not all AM service bureaus are created equal. Procurement managers and engineering teams must conduct thorough due diligence to identify a partner with the necessary expertise, capabilities, and certifications.
Key criteria for evaluating and selecting a metal AM supplier for aerospace heat shields include:
- Aerospace Expertise and Experience:
- Requirement: Demonstrated track record of successfully producing components for the aerospace or defense industries. Understanding of aerospace materials, specifications (e.g., AMS, MIL-STD), and quality expectations.
- Evaluation: Case studies, customer references within aerospace, familiarity with heat shield applications or similar high-temperature, structural components. Does the supplier understand the criticality and specific challenges of thermal protection systems?
- Relevant Technology and Equipment:
- Requirement: Possession of industrial-grade metal AM systems (LPBF, EBM) suitable for the chosen material (Ti-6Al-4V, AlSi10Mg, superalloys) and required part size/volume. State-of-the-art equipment often translates to better process control, accuracy, and reliability.
- Evaluation: Specific printer models used, build envelope size, beam type (laser/electron beam), process monitoring capabilities. Suppliers like Met3dp, who manufacture their own advanced printers, often have deep technical knowledge of their systems’ capabilities and limitations. Assess their maintenance and calibration procedures.
- Material Capabilities and Quality:
- Requirement: Ability to process the specified aerospace alloys (e.g., Ti-6Al-4V, AlSi10Mg) with validated parameters. Crucially, they must use high-quality, aerospace-grade metal powders with documented traceability and certification.
- Evaluation: Range of materials offered, powder sourcing (in-house production like Met3dp’s advanced atomization vs. external suppliers), powder handling protocols, material testing capabilities, availability of material certifications (e.g., Certificate of Conformance).
- Quality Management System (QMS) and Certifications:
- Requirement: A robust QMS is non-negotiable for aerospace. Look for certifications like AS9100 (specific to aerospace), ISO 9001, and potentially NADCAP accreditation for special processes like heat treatment or NDT if performed in-house.
- Evaluation: Request copies of certifications, audit reports (if possible), and inquire about their quality control procedures, traceability systems (from powder batch to final part), and non-conformance handling processes.
- Post-Processing Capabilities and Network:
- Requirement: As discussed, post-processing is essential. The ideal supplier either has in-house capabilities (stress relief, machining, finishing, HIP, NDT) or a well-managed network of qualified partners for these services.
- Evaluation: List of in-house vs. outsourced post-processing steps. If outsourced, how are partners qualified and managed? Can they provide a complete, finished part? Assess their experience managing the entire workflow.
- Technical Expertise and Support:
- Requirement: Access to experienced AM engineers and metallurgists who can provide DfAM support, advise on material selection, optimize build strategies, and troubleshoot potential issues.
- Evaluation: Qualifications and experience of the engineering team, availability for design reviews, responsiveness to technical queries, willingness to collaborate on development projects.
- Capacity and Lead Time:
- Requirement: Sufficient machine capacity to meet project timelines, from prototyping to potential production volumes. Ability to provide realistic and reliable lead time estimates.
- Evaluation: Number of relevant machines, current workload, historical performance on lead time commitments. Ask about their production planning and scheduling processes.
- Cost Competitiveness:
- Requirement: Transparent and competitive pricing structure. While cost is always a factor, it should be balanced against quality, reliability, and expertise, especially for critical aerospace components.
- Evaluation: Request detailed quotes outlining costs for printing, material, supports, post-processing, NDT, and any NRE (Non-Recurring Engineering) charges. Compare quotes on a like-for-like basis, considering the entire scope of supply.
Table: Supplier Selection Checklist for AM Heat Shields
Kritéria | Key Questions to Ask | Importance Rating |
---|---|---|
Aerospace Experience | Can you provide aerospace case studies/references? Do you understand TPS challenges? | ★★★★★ |
Technologie a vybavení | What specific printers/systems do you use? What is the build volume? How new is the equipment? | ★★★★☆ |
Material Capability/Quality | Can you process [Alloy]? How do you ensure powder quality/traceability? Certs available? | ★★★★★ |
QMS & Certifications | Are you AS9100 certified? Can we see your quality manual/certs? How is traceability managed? | ★★★★★ |
Následné zpracování | What steps are in-house vs. outsourced? How do you manage partners? Can you deliver finished parts? | ★★★★★ |
Technická podpora | What DfAM support do you offer? Who are the technical contacts? | ★★★★☆ |
Capacity & Lead Time | What is your capacity for this project? What are realistic lead times? | ★★★★☆ |
Nákladová konkurenceschopnost | Can you provide a detailed cost breakdown? What are payment terms? | ★★★☆☆ |
Export to Sheets
Choosing the right AM partner is an investment in quality and reliability. Companies like Met3dp, which offer comprehensive solutions encompassing advanced printers, high-quality metal powders, and application development services, represent the type of integrated provider that can significantly de-risk the adoption of AM for critical components like aerospace heat shields. Taking the time to thoroughly vet potential suppliers against these criteria will ensure a successful partnership and high-quality results for demanding B2B aerospace projects.
Understanding Costs and Lead Times for AM Aerospace Heat Shields
For procurement managers and project engineers, accurately estimating the cost and lead time associated with producing aerospace heat shields via additive manufacturing is crucial for budgeting, planning, and project viability. Unlike high-volume traditional manufacturing, AM costs and timelines are influenced by a different set of factors. Understanding these drivers allows for more realistic expectations and better communication with AM service providers.
Factors Influencing Cost:
- Part Volume and Mass:
- Dopad: Larger or heavier parts consume more material and take longer to print, directly increasing costs. DfAM techniques like topology optimization and lattice structures are key to minimizing volume while meeting requirements.
- Consideration: Material cost per kilogram (e.g., Ti-6Al-4V is significantly more expensive than AlSi10Mg) is a major driver. Efficient nesting of multiple parts within a single build can reduce per-part cost but requires careful planning.
- Part Complexity and Geometry:
- Dopad: Highly complex geometries with intricate features, thin walls, or extensive internal channels may require longer setup times, slower printing speeds for accuracy, and significantly more effort in post-processing (especially powder removal and support removal).
- Consideration: While AM enables complexity, overly complex designs can inflate costs unnecessarily. DfAM should balance performance gains with manufacturability and post-processing effort.
- Typ materiálu:
- Dopad: The raw material powder cost varies significantly between alloys. Titanium alloys and nickel superalloys are substantially more expensive than aluminum or steel alloys. Powder quality (sphericity, purity, PSD) also influences cost – high-quality powders command a premium but yield better results. Met3dp’s investment in advanced powder production aims to provide high-quality materials efficiently.
- Consideration: Ensure the material selected is truly necessary for the application’s demands.
- Build Height (Z-Height):
- Dopad: Print time is heavily dependent on the number of layers, which is determined by the part’s height in the build orientation. Taller parts take longer to print, increasing machine time costs.
- Consideration: Optimizing part orientation to minimize build height (while considering support needs and surface finish) can reduce print time and cost.
- Support Structure Requirements:
- Dopad: Parts requiring extensive support structures consume more material (supports are often made from the same expensive alloy) and significantly increase post-processing labor time for removal and subsequent surface finishing.
- Consideration: DfAM efforts to minimize supports are crucial for cost reduction.
- Post-Processing Intensity:
- Dopad: This is often a major cost driver. Requirements for HIP, extensive CNC machining for tight tolerances, high-level surface finishing (polishing, AFM), and rigorous NDT add significant cost and time.
- Consideration: Clearly define which features truly require tight tolerances or specialized finishing to avoid unnecessary costs.
- Quality Assurance and Certification:
- Dopad: Aerospace requires stringent QA, including material certifications, process documentation, dimensional inspection (CMM), NDT (X-ray/CT), and potentially qualification testing. These activities add cost.
- Consideration: Factor in the cost of compliance and certification early in the project planning.
- Objem objednávky:
- Dopad: Like most manufacturing, economies of scale apply to AM, though perhaps less dramatically than traditional tooling-based methods. Setup costs and programming time can be amortized over larger batches, reducing the per-part cost.
- Consideration: Discuss potential volume discounts for batch production or recurring orders.
Factors Influencing Lead Time:
- Engineering and Setup Time: Initial file preparation, DfAM review, build simulation, support generation, and machine setup contribute to the initial lead time.
- Machine Availability and Print Queue: Lead time depends heavily on the supplier’s current workload and the availability of the specific machine required.
- Actual Print Time: Determined primarily by part volume, height, and complexity. Can range from hours for small parts to many days for large, complex components or full build plates.
- Cooling Time: Parts need to cool down within the machine under an inert atmosphere after printing, which can take several hours.
- Post-Processing Sequence: Each step (stress relief, part removal, support removal, HIP, machining, finishing, inspection) adds time. The total post-processing time can often exceed the print time, especially if multiple steps are involved or require transport between different facilities (if outsourced).
- Quality Assurance and Inspection: Thorough inspection and documentation take time.
- Shipping and Logistics: Standard shipping times apply.
Typical Lead Time Estimates:
- Prototypy: Often 1-3 weeks, depending on complexity and post-processing needs.
- Low Volume Production: Typically 3-8 weeks, heavily dependent on post-processing intensity and qualification requirements.
- Poznámka: These are rough estimates; specific project timelines require detailed quoting from the supplier.
Table: Key Cost & Lead Time Drivers for AM Heat Shields
Řidič | Dopad na náklady | Impact on Lead Time | Mitigation / Consideration |
---|---|---|---|
Part Volume/Mass | High (Material, Print Time) | High (Print Time) | DfAM (Lightweighting), Efficient Nesting |
Složitost části | Moderate/High (Setup, Post-Pro. Effort) | Moderate/High (Setup, Print, Post-Pro.) | Balance complexity with manufacturability, DfAM |
Typ materiálu | High (Powder Price) | Minimální | Justify material choice, Ensure quality |
Build Height (Z) | Moderate (Print Time) | High (Print Time) | Optimize orientation |
Podpůrné struktury | Moderate (Material, Removal Labor) | Moderate (Removal Time) | DfAM (Minimize Supports) |
Následné zpracování | Very High (HIP, Machining, Finishing, NDT) | Very High (Multiple sequential steps) | Specify only necessary steps, Integrated supplier |
QA / Certification | Moderate/High (Testing, Documentation) | Moderate (Testing, Reporting) | Plan for compliance requirements |
Objem objednávky | Moderate (Amortization of Setup) | Moderate (Efficient Scheduling) | Discuss batch production benefits |
Export to Sheets
Understanding these factors enables more productive discussions with AM suppliers and helps set realistic expectations for bringing advanced, lightweight AM heat shields into aerospace applications. Engaging with experienced providers early allows for optimization strategies that can positively impact both cost and lead time.
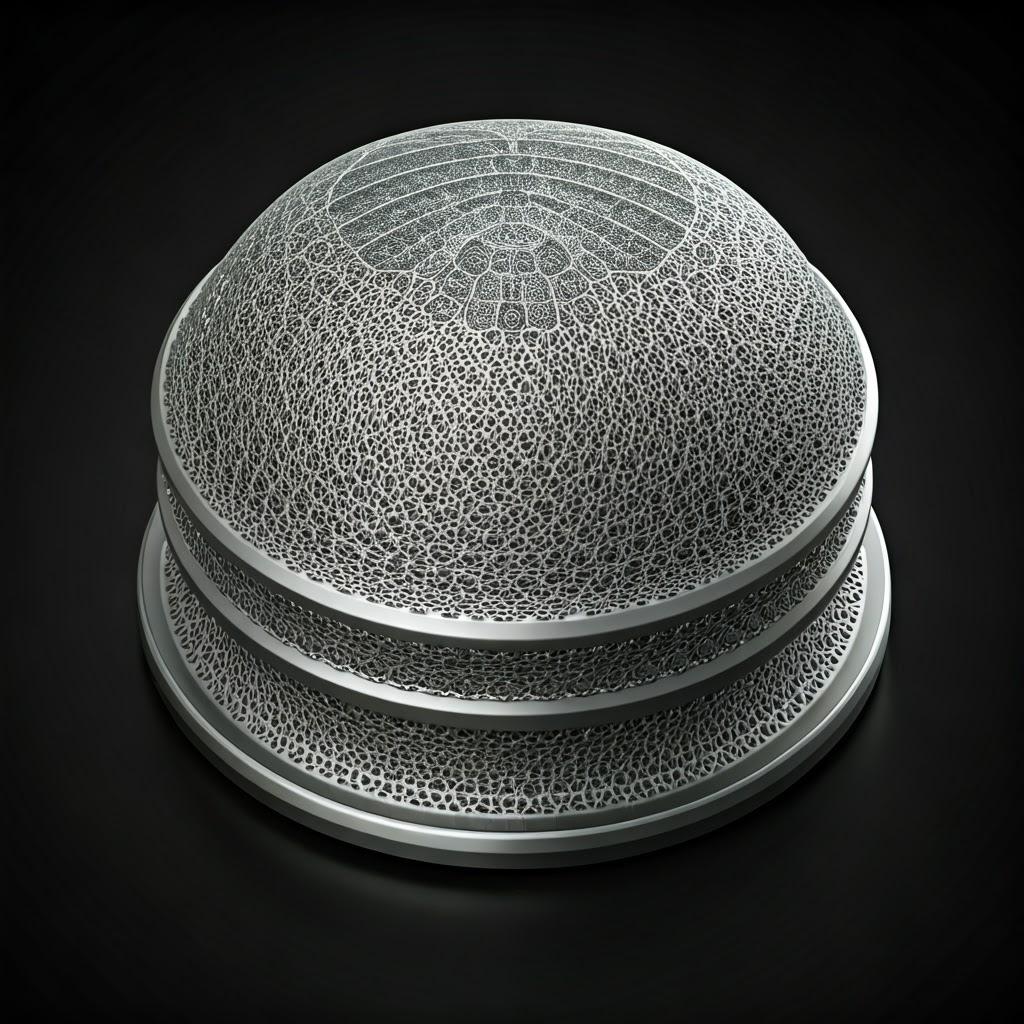
Frequently Asked Questions (FAQ) about AM Heat Shields
Here are some common questions engineers and procurement managers have about using metal additive manufacturing for aerospace heat shields:
- Q: Can AM produce heat shields that match the performance of traditionally manufactured ones?
- A: Yes, and often exceed it. AM allows for optimized designs (topology optimization, internal cooling channels, lattice structures) and part consolidation that are impossible with traditional methods. This leads to lighter weight components with potentially superior thermal management capabilities. Achieving equivalent or better performance requires proper DfAM, validated process parameters, high-quality materials (like Ti-6Al-4V or AlSi10Mg), and necessary post-processing (like HIP for optimal material properties). The key is leveraging AM’s unique strengths, not just replicating old designs.
- Q: What are the main challenges in ensuring the quality and reliability of AM aerospace heat shields?
- A: Key challenges include managing residual stress and distortion during the build, minimizing internal porosity to ensure material integrity (especially fatigue life), achieving tight dimensional tolerances and required surface finishes (often through post-processing), consistent removal of support structures without damaging the part, and ensuring repeatable mechanical properties build after build. Overcoming these requires rigorous process control, high-quality feedstock powder, appropriate post-processing (stress relief, HIP), comprehensive NDT inspection (like CT scanning), and adherence to strict aerospace quality management systems (like AS9100).
- Q: Is metal AM cost-effective for producing heat shields compared to traditional methods?
- A: It depends on several factors. For highly complex designs, low-to-medium production volumes, or components where significant lightweighting offers substantial system-level benefits (fuel savings, increased payload), AM can be very cost-effective, especially when considering total lifecycle cost. Part consolidation via AM also reduces assembly costs. However, for simpler geometries produced in high volumes, traditional methods like stamping or machining might still be cheaper on a per-part basis. The “buy-to-fly” ratio (material used vs. final part weight) is much better in AM for expensive materials like Titanium, reducing waste compared to machining. A detailed cost analysis considering design complexity, volume, material, and post-processing is needed for each specific case.
Conclusion: The Future of Aerospace Thermal Protection is Additive
The demands placed on aerospace thermal protection systems continue to escalate, driven by ambitions for hypersonic flight, more efficient aircraft engines, reusable spacecraft, and advanced satellite technology. Traditional manufacturing methods, while reliable, often struggle to meet the simultaneous requirements for extreme temperature resistance, complex geometries, structural integrity, and aggressive lightweighting demanded by these next-generation applications.
Metal Additive Manufacturing has emerged as a powerful, enabling technology capable of overcoming these limitations. As we’ve explored, AM offers unparalleled design freedom, allowing engineers to create heat shields with integrated cooling channels, optimized topologies, and lightweight lattice structures – features previously unattainable. The ability to consolidate multiple components into a single printed part reduces weight, complexity, and potential failure points. Utilizing advanced aerospace alloys like Ti-6Al-4V and AlSi10Mg, AM delivers high-performance materials precisely where needed, minimizing waste and maximizing efficiency.
While challenges related to process control, post-processing, and qualification exist, they are being systematically addressed through advancements in DfAM principles, machine technology, process monitoring, material science, and standardization efforts within the industry. Choosing the right partner – one with deep expertise in aerospace applications, robust quality systems (AS9100), advanced equipment, high-quality material supply, and comprehensive post-processing capabilities – is paramount to successfully leveraging AM for critical components like heat shields.
Companies like Met3dp are at the vanguard of this manufacturing transformation, providing not just the advanced metal powders and industry-leading printers but also the application development expertise needed to help aerospace organizations implement AM effectively. By embracing additive manufacturing, the aerospace industry can unlock new levels of performance, efficiency, and innovation in thermal management. The lightweight, complex, and highly optimized heat shields produced via AM are not just components; they are key enablers for the future of flight and space exploration.
Sdílet na
Facebook
Cvrlikání
LinkedIn
WhatsApp
E-mailem
MET3DP Technology Co., LTD je předním poskytovatelem řešení aditivní výroby se sídlem v Qingdao v Číně. Naše společnost se specializuje na zařízení pro 3D tisk a vysoce výkonné kovové prášky pro průmyslové aplikace.
Dotaz k získání nejlepší ceny a přizpůsobeného řešení pro vaše podnikání!
Související články
O Met3DP
Nedávná aktualizace
Náš produkt
KONTAKTUJTE NÁS
Nějaké otázky? Pošlete nám zprávu hned teď! Po obdržení vaší zprávy obsloužíme vaši žádost s celým týmem.
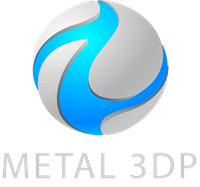
Kovové prášky pro 3D tisk a aditivní výrobu
SPOLEČNOST
PRODUKT
kontaktní informace
- Město Qingdao, Shandong, Čína
- [email protected]
- [email protected]
- +86 19116340731