3D Printed Turbocharger Housings for High Performance Vehicles
Inhaltsübersicht
Revolutionizing Performance with 3D Printed Turbocharger Housings
In the relentless pursuit of automotive performance, engineers continually push the boundaries of engine technology. Central to forced induction systems, the turbocharger is a critical component, and its housing plays a far more significant role than merely containing the compressor and turbine wheels. The turbocharger housing—comprising the cool-side compressor housing and the hot-side turbine housing—must endure extreme temperatures, high pressures, significant vibrations, and corrosive exhaust gases while precisely guiding airflow to maximize efficiency and engine power. Traditionally, manufacturing these complex components involved casting or extensive machining, methods often entailing compromises in design, material properties, or lead times, especially for specialized applications.
Eintritt in die Ära der Additive Fertigung (AM), allgemein bekannt als 3D-Druck. Konkret, 3D-Druck von Metall is rapidly emerging as a transformative force in the production of high-performance automotive components, including turbocharger housings. This technology allows for the layer-by-layer construction of intricate metal parts directly from digital designs, unlocking unprecedented levels of design freedom, enabling the use of advanced materials, and significantly accelerating development cycles. For engineers striving for optimal aerodynamic efficiency, lightweighting, and thermal management, and for procurement managers seeking flexible, high-value manufacturing solutions for custom turbocharger components, metal AM offers a compelling alternative to conventional methods.
This shift is particularly relevant in demanding sectors like motorsport, high-performance aftermarket tuning, and niche vehicle manufacturing, where off-the-shelf components often fall short. The ability to create bespoke high-performance turbochargers with optimized geometries tailored to specific engine requirements provides a tangible competitive edge. Companies like Met3dp are at the forefront of this revolution, providing not only industry-leading metal AM printers known for their accuracy and reliability but also high-performance metal powders engineered for the demanding conditions within metal 3D printing powertrain applications. As we delve deeper, we will explore precisely how this technology is reshaping turbocharger design and manufacturing, offering significant advantages for businesses operating in the high-performance automotive landscape.
Core Applications: Where are 3D Printed Turbocharger Housings Making an Impact?
The unique capabilities of metal additive manufacturing are driving the adoption of 3D printed turbocharger housings across several key segments within the automotive industry. While not yet mainstream for mass-produced commuter vehicles (where traditional casting efficiencies still dominate high volumes), AM shines brightly where customization, peak performance, and rapid development are paramount. Procurement specialists and engineers sourcing motorsport components manufacturing solutions or seeking suppliers for performance vehicle upgrades are increasingly turning to AM.
Here’s a breakdown of the core applications:
- Motorsport and Racing:
- Competitive Edge: In Formula 1, endurance racing (like WEC), rally championships, and other top-tier series, every gram of weight and every fraction of aerodynamic efficiency counts. AM allows teams to create highly optimized, lightweight housings with complex internal volutes and passages tailored precisely to their engine’s operating characteristics and packaging constraints.
- Schnelle Iteration: Design changes can be implemented and tested in days or weeks, rather than the months required for new casting tooling. This agility is crucial for staying competitive throughout a racing season.
- Material Superiority: Racing environments push materials to their absolute limits. AM enables the use of high-temperature superalloys like Inconel 625 or 718, ensuring durability under extreme thermal loads that might challenge conventional materials or manufacturing methods for complex shapes.
- Low Volume, High Value: Race teams typically require only a small number of highly specialized components, making the tooling-free nature of AM economically viable and strategically advantageous.
- High-Performance Aftermarket:
- Verbesserte Leistung: Aftermarket turbocharger suppliers and tuning companies cater to enthusiasts seeking significant power gains. 3D printing allows them to design and offer housings with superior airflow characteristics, improved wastegate designs, and potentially integrated features not found in OEM parts.
- Unique Geometries: AM enables the creation of housings with complex shapes, such as twin-scroll turbine housings with highly optimized, separated passages, or compressor covers with advanced anti-surge features, which can be difficult or impossible to achieve efficiently with casting.
- Anpassungen: For specific engine swaps or unique builds, AM allows for the creation of bespoke housings that perfectly fit the available space and interface correctly with other engine components.
- Brand Differentiation: Offering advanced, 3D printed components can serve as a significant differentiator in the competitive aftermarket landscape.
- OEM Prototyping and Niche Vehicles:
- Beschleunigte Entwicklung: During the OEM turbocharger development phase for new engines, AM enables engineers to rapidly prototype and functionally test multiple housing designs. This drastically cuts down R&D timelines and costs associated with traditional prototyping methods. Design flaws can be identified and corrected quickly before committing to expensive mass-production tooling.
- Niche Vehicle Production: For hypercars, supercars, and other specialty vehicles produced in limited numbers (low-volume automotive production), the high cost of casting tooling is often prohibitive. Metal AM provides a cost-effective manufacturing solution for producing the final, functional turbocharger housings for these exclusive vehicles without requiring investment in dedicated molds.
- Teil Konsolidierung: AM allows designers to integrate features like brackets, sensor bosses, or heat shields directly into the housing design, potentially reducing part count, assembly time, and points of failure.
Functional Benefits Realized Through AM:
Across these applications, the functional advantages translate directly into measurable performance gains:
- Optimized Aerodynamics: Precisely controlled internal geometries (volutes, diffusers) lead to higher compressor and turbine efficiency, resulting in more power and faster throttle response (reduced turbo lag).
- Improved Thermal Management: Integration of sophisticated internal cooling channels, particularly in the turbine housing, helps manage extreme temperatures, improving material durability and maintaining performance consistency under load.
- Gewichtsreduzierung: Topology optimization algorithms, facilitated by AM, remove material from non-critical areas, leading to lighter components. This is vital in motorsport and contributes to overall vehicle efficiency.
- Enhanced Packaging: The design freedom allows housings to be shaped to fit tightly constrained engine bays, crucial in modern vehicles packed with technology.
For B2B buyers, sourcing 3D printed turbocharger housings means accessing cutting-edge technology that delivers tangible performance benefits, faster development cycles for custom projects, and a flexible manufacturing solution for specialized, high-value components.
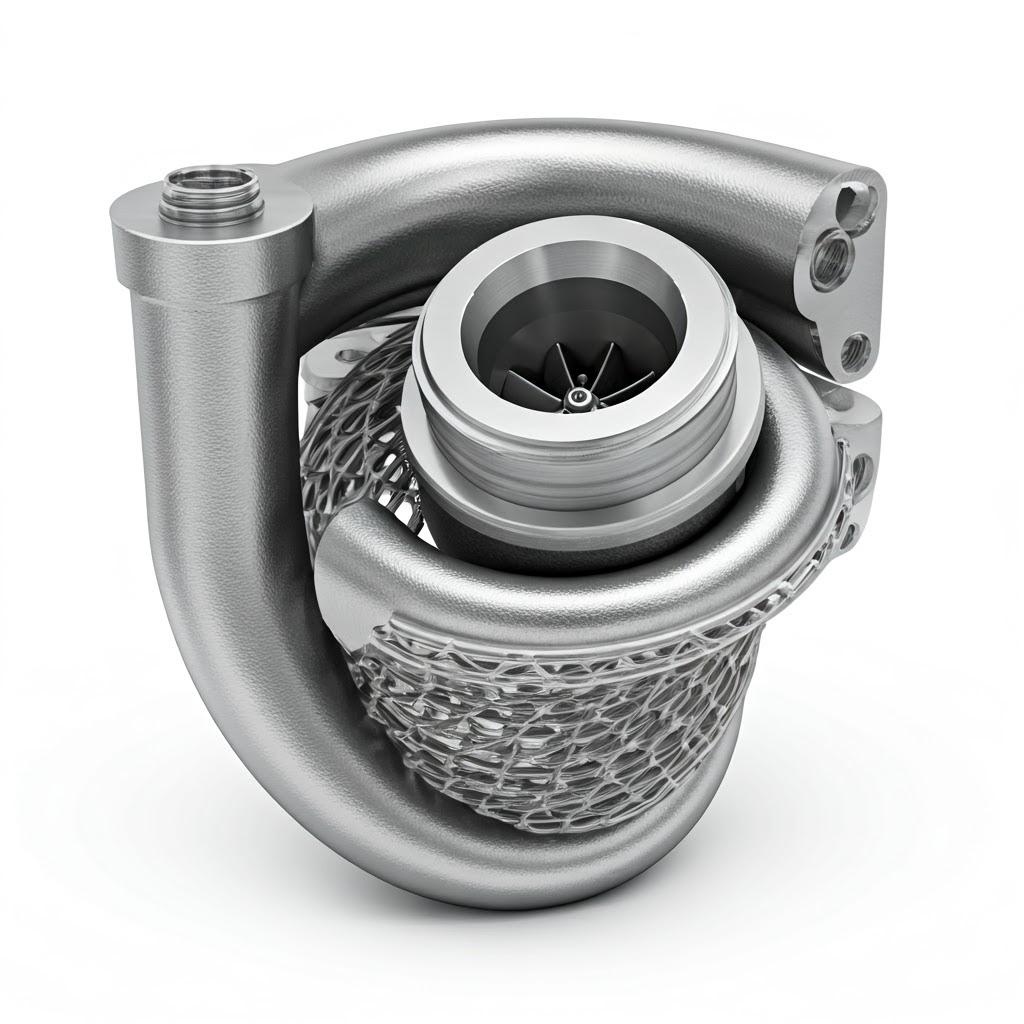
The Additive Advantage: Why Choose Metal 3D Printing for Turbocharger Housings?
While traditional manufacturing methods like casting and machining have served the automotive industry well for decades, metal additive manufacturing presents a paradigm shift, offering distinct advantages, particularly for complex, performance-critical components like turbocharger housings. Understanding these benefits is crucial for engineers and procurement managers evaluating production methods for next-generation powertrain components. Let’s compare AM against conventional techniques and highlight the benefits metal AM automotive applications bring.
AM vs. Traditional Methods:
- Feinguss:
- Vorteile: Excellent surface finish, good dimensional accuracy, suitable for complex shapes, cost-effective at high volumes.
- Nachteile: Requires expensive and time-consuming tooling (wax patterns, ceramic shells), significant design constraints (e.g., draft angles, uniform wall thickness), limited internal complexity (cores can be fragile), long lead times for initial parts. Difficult to iterate designs quickly.
- Sand Casting:
- Vorteile: Lower tooling cost than investment casting, suitable for larger parts.
- Nachteile: Poorer surface finish and dimensional accuracy, requires secondary machining, significant design limitations, less suitable for very thin walls or highly intricate details.
- Machining from Billet:
- Vorteile: High precision, excellent material properties (wrought stock), no tooling cost.
- Nachteile: Extremely high material waste (buy-to-fly ratio), very difficult or impossible to create complex internal features (like volutes), long machining times for complex external shapes, potentially high cost per part due to waste and machine time.
- Metal Additive Manufacturing (LPBF/SEBM):
- Vorteile: Tooling-free productionermöglicht rapid prototyping turbo parts and low-volume manufacturing cost-effectively. Unmatched complex geometry manufacturing automotive capability, including intricate internal channels and organic shapes. Facilitates lightweight turbocharger design through topology optimization. Enables part consolidation. Wide range of materials, including high-performance superalloys. Significant lead time reduction automotive development cycles.
- Nachteile: Higher cost per part compared to casting at very high volumes. Typically requires post-processing (heat treatment, support removal, surface finishing, final machining). Build size limitations depending on the machine. Requires expertise in Design for Additive Manufacturing (DfAM).
Key Advantages of AM for Turbocharger Housings:
- Beispiellose Designfreiheit: This is arguably the most significant advantage. AM liberates designers from the constraints of traditional methods.
- Beispiel: Engineers can design compressor and turbine volutes with continuously varying cross-sections optimized purely for fluid dynamics, maximizing efficiency across the operating range. Internal cooling channels can follow complex paths directly adjacent to the hottest areas of the turbine housing, something virtually impossible with casting. Wastegate passages can be integrated seamlessly with optimal flow paths.
- Lightweighting through Topology Optimization: AM works synergistically with computational design tools. Software can analyze stress distributions and remove material from areas where it’s not structurally required, creating strong yet lightweight organic shapes.
- Beispiel: A 3D printed turbine housing might feature an intricate lattice structure externally, maintaining rigidity while significantly reducing mass compared to a solid cast counterpart. This reduces overall vehicle weight and can potentially improve transient response.
- Enhanced Thermal Management: The ability to incorporate complex internal cooling channels directly within the housing walls is a game-changer, especially for the turbine side enduring extreme exhaust temperatures.
- Beispiel: Liquid or air-cooling channels can be printed just millimeters away from the hottest gas passages, drastically lowering material temperatures, improving durability, preventing heat soak, and allowing for more aggressive engine tuning.
- Rapid Prototyping und Iteration: The tooling-free nature of AM means design modifications can be implemented and tested physically within days.
- Beispiel: An engineering team can test three different volute designs back-to-back on a dynamometer in a week, gathering empirical data to select the optimal configuration. With casting, this process could take many months and incur substantial tooling costs for each iteration.
- Advanced Material Utilization: AM processes like Selective Electron Beam Melting (SEBM) and Laser Powder Bed Fusion (LPBF) excel at processing high-performance materials essential for turbochargers.
- Beispiel: Nickel-based superalloys like IN718 and IN625, known for their high-temperature strength and corrosion resistance, can be readily processed via AM into dense, near-net-shape parts. These materials are often challenging or expensive to work with using traditional methods, especially for complex geometries.
- Teil Konsolidierung: Components that were previously separate and required assembly (e.g., housing, mounting brackets, heat shields) can potentially be integrated into a single printed part.
- Beispiel: A turbocharger housing could be printed with integrated mounting points and structural reinforcements, reducing part count, assembly labor, weight, and potential leak paths or failure points.
For B2B customers, these advantages translate into tangible value: faster time-to-market for innovative products, superior component performance leading to a competitive edge, simplified logistics for low-volume parts, and the ability to create solutions previously deemed impossible. Partnering with an experienced AM provider ensures these benefits are fully realized through optimized design, material selection, and process control.
Material Focus: IN718 & IN625 for Demanding Turbocharger Environments
Selecting the right material is paramount for turbocharger housings, given the incredibly harsh operating conditions they face: temperatures potentially exceeding 1000°C (1832°F) on the turbine side, high pressures, cyclic thermal loading, vibrations, and exposure to corrosive exhaust byproducts. While various materials are used in mass-produced turbochargers (like cast irons or stainless steels), high-performance and demanding applications necessitate the use of nickel-based superalloys. Among these, Inconel 718 (IN718) and Inconel 625 (IN625) stand out as prime candidates for metal additive manufacturing, offering an exceptional combination of properties readily processable via AM. Understanding their characteristics helps in selecting the optimal material for specific housing requirements.
Why Superalloys?
Nickel-based superalloys are engineered to maintain excellent mechanical strength, surface stability, and corrosion/oxidation resistance at extremely high temperatures where conventional steels would fail. This makes them ideal for the punishing environment within a turbocharger, particularly the turbine housing directly exposed to hot exhaust gases.
Inconel 718 (IN718 / Alloy 718)
- Zusammensetzung: Nickel-Chromium alloy containing significant amounts of Iron, Niobium, and Molybdenum, with additions of Aluminum and Titanium.
- Wichtige Eigenschaften:
- High Strength & Creep Resistance: Maintains excellent tensile, fatigue, and creep-rupture strength up to approximately 700°C (1300°F).
- Age-Hardenable: Its strength is derived primarily from precipitation hardening (aging heat treatment) involving Ni3(Nb, Ti, Al) phases. This allows for very high strength levels.
- Gute Korrosionsbeständigkeit: Offers good resistance to oxidation and corrosion in typical engine environments.
- Excellent Printability/Weldability: Compared to other precipitation-hardened superalloys, IN718 exhibits very good processability in both welding and additive manufacturing, making it a popular choice for complex AM components.
- Turbocharger Relevance: Often used for compressor housings (where temperatures are lower) and potentially for turbine housings in applications where peak exhaust temperatures are moderately high. Its excellent strength-to-weight ratio after heat treatment is highly beneficial. It’s generally slightly less expensive than IN625.
Inconel 625 (IN625 / Alloy 625)
- Zusammensetzung: Nickel-Chromium-Molybdenum-Niobium alloy.
- Wichtige Eigenschaften:
- Superior High-Temperature Strength: Relies on solid-solution strengthening from Molybdenum and Niobium, providing excellent strength and toughness at temperatures up to 815°C (1500°F) and even higher for short excursions. It retains strength better than IN718 at the highest temperatures seen in aggressive turbo applications.
- Hervorragende Korrosionsbeständigkeit: The high Chromium and Molybdenum content imparts superb resistance to a wide range of corrosive environments, including pitting, crevice corrosion, and acidic condensates found in exhaust streams. It generally outperforms IN718 in corrosion resistance.
- Excellent Fabricability & Printability: Known for its ease of fabrication and excellent weldability/printability, making it highly suitable for complex AM geometries.
- Zähigkeit: Exhibits high toughness and fatigue strength across a broad temperature range.
- Turbocharger Relevance: The preferred choice for high-performance turbine housings, especially those subjected to the most extreme temperatures and potentially corrosive fuel mixtures (e.g., high ethanol content). Its superior resistance to heat and corrosion provides a greater margin of safety and durability in demanding motorsport or highly tuned aftermarket applications.
IN718 vs. IN625 for 3D Printed Turbo Housings:
Merkmal | Inconel 718 (IN718) | Inconel 625 (IN625) | Relevance to Turbo Housings |
---|---|---|---|
Max Service Temp. | ~700°C (1300°F) | ~815°C+ (1500°F+) | IN625 better suited for extreme exhaust temps (turbine side) |
Strength Mechanism | Precipitation Hardening (Aging Req.) | Solid-Solution Strengthening | IN718 potentially higher strength <700°C, requires heat treat |
Strength at >700°C | Decreases more rapidly | Maintains strength better | IN625 superior for hottest parts of turbine housing |
Korrosionsbeständigkeit | Gut | Ausgezeichnet | IN625 offers more robustness against aggressive exhaust gases |
Printability/Weldability | Sehr gut | Ausgezeichnet | Both are well-established materials for metal AM |
Kosten | Im Allgemeinen niedriger | Im Allgemeinen höher | Cost must be weighed against performance/durability needs |
Typische Anwendung | Compressor Housing, Moderate Turbine Side | Turbine Housing, Highly Demanding Applications | Match material choice to the specific operational environment |
In Blätter exportieren
Die Rolle von Met3dp bei der Materialexzellenz:
Successfully 3D printing turbocharger housings from demanding materials like IN718 and IN625 requires not only advanced printing equipment but also exceptionally high-quality metal powder. This is where Met3dp excels. Leveraging industry-leading powder manufacturing techniques like Vacuum Gas Atomization (VIGA) and Plasma Rotating Electrode Process (PREP), Met3dp produces Metallpulver with characteristics critical for successful additive manufacturing:
- Hohe Sphärizität: Ensures excellent powder flowability and high packing density in the powder bed, leading to denser, stronger final parts.
- Niedriger Satellitengehalt: Minimizes fine particles attached to larger spheres, further improving flowability and reducing potential defects.
- Kontrollierte Partikelgrößenverteilung (PSD): Optimized PSD for specific AM processes (like LPBF or SEBM) ensures consistent melting and layer formation.
- Hohe Reinheit: Minimizes contaminants that could compromise material properties.
Met3dp manufactures a wide range of high-quality metal powders, including innovative alloys and standard superalloys like IN718 and IN625, optimized for laser and electron beam powder bed fusion processes. Their deep expertise in both powder production and AM printing systems ensures that customers receive materials perfectly suited for manufacturing mission-critical components like high-performance turbocharger housings. Choosing a supplier like Met3dp, with control over both the material science and the printing process, provides confidence in achieving the desired material properties and component reliability.
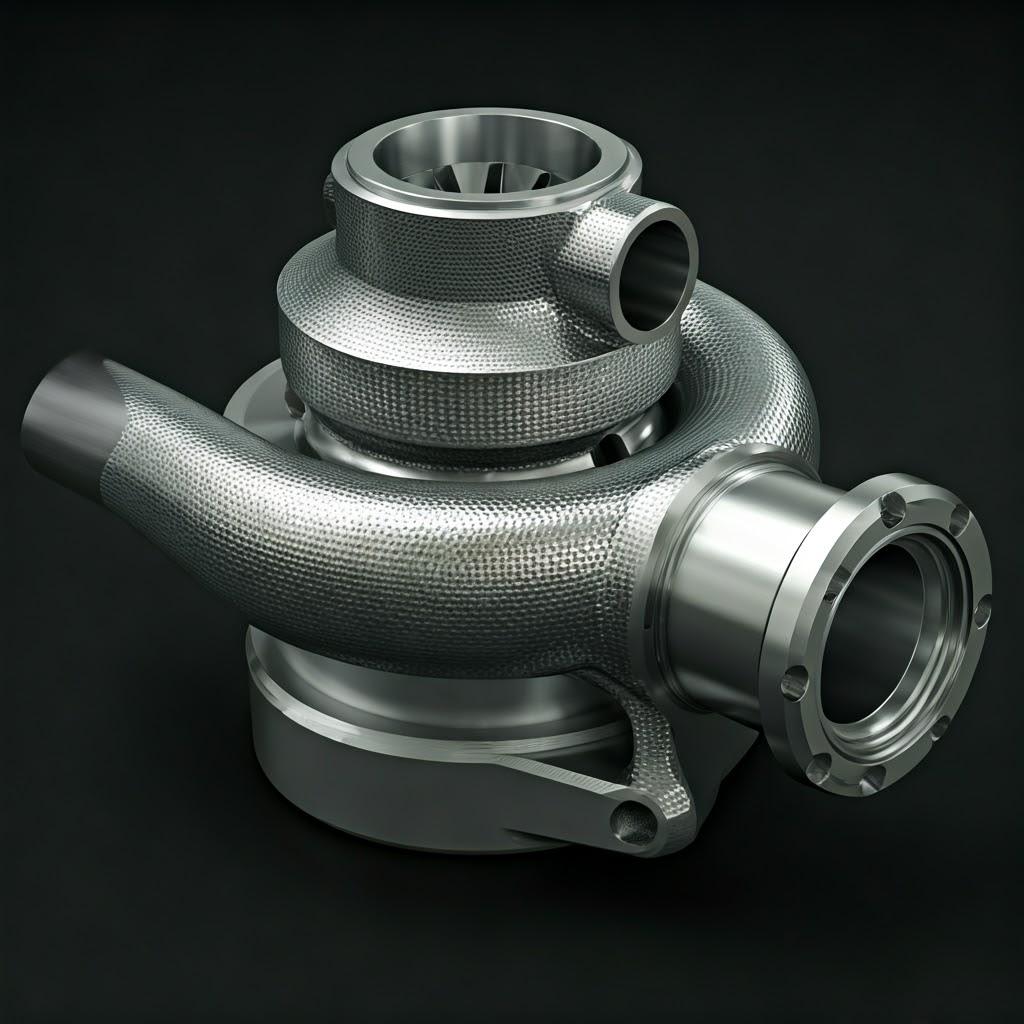
Design for Additive Manufacturing (DfAM): Optimizing Turbocharger Housings for 3D Printing
A common misconception when adopting metal additive manufacturing is that existing designs, originally intended for casting or machining, can simply be sent to the printer. While technically possible, this approach fails to leverage the unique strengths of AM and often leads to suboptimal results, increased costs, or even print failures. To truly unlock the potential of 3D printed turbocharger housings, Design für additive Fertigung (DfAM) is not just recommended—it’s essential. DfAM involves rethinking the design process from the ground up, considering both the freedoms and the constraints of the layer-by-layer building process. Engineers and designers involved in DfAM automotive parts development focus on maximizing performance while ensuring manufacturability.
Key DfAM Principles for Turbocharger Housings:
- Optimierung der Fließwege: This is where AM truly shines for turbochargers. Forget the limitations of casting cores. Design smooth, continuously varying volutes and passages that precisely match the aerodynamic requirements of the compressor and turbine wheels. Utilize Computational Fluid Dynamics (CFD) extensively during the design phase to iterate and perfect airflow paths, minimize pressure drops, and optimize wastegate flow for better boost control. Consider integrating features like bellmouth entries or optimized diffusers directly into the housing.
- Integrated Complex Features: Leverage AM’s ability to create intricate internal structures.
- Internal Cooling Channels: Design conformal cooling channels that follow the contours of the hottest sections of the turbine housing (e.g., near the volute tongue or turbine inlet). These channels, impossible to create with traditional methods, allow for targeted cooling (liquid or air), drastically improving thermal management, material durability, and performance consistency under load. Ensure channels are designed for printability (self-supporting angles where possible) and complete powder removal.
- Sensor Integration: Bosses and ports for pressure, temperature, or speed sensors can be seamlessly integrated into the design, potentially in optimized locations not previously accessible.
- Minimierung und Optimierung der Stützstruktur: Support structures are often necessary in metal AM (especially LPBF) to anchor the part, manage thermal stress, and support overhanging features. However, they consume extra material, add print time, and require removal in post-processing.
- Strategische Ausrichtung: The way a part is oriented on the build plate dramatically impacts support needs, surface finish, and potential distortion. Analyze different orientations to find the best balance.
- Selbsttragende Winkel: Design overhangs to be self-supporting whenever possible. Angles greater than approximately 45 degrees from the horizontal plane often print successfully without supports (though this depends on the specific process and material). Use chamfers or fillets instead of sharp horizontal overhangs.
- DfAM for Support Removal: Ensure supports, especially internal ones, are accessible for removal. Design access ports if necessary or consider processes like SEBM which may require fewer supports due to higher build chamber temperatures.
- Wall Thickness and Lightweighting:
- Topologie-Optimierung: Use specialized software to identify and remove material from low-stress areas, creating organic, load-path-optimized structures. This is key for topology optimization turbocharger designs aimed at motorsport or maximum performance, reducing rotational inertia (if applied to rotating components) and overall vehicle weight.
- Mindestwanddicke: AM allows for relatively thin walls (e.g., 0.4mm – 1.0mm depending on process and unsupported span), but ensure walls are thick enough for structural integrity, handling, and printability. Avoid large, unsupported thin horizontal sections.
- Avoid Thick Sections: Very thick, solid sections can accumulate excessive thermal stress, increasing the risk of cracking or warping. Consider designing internal lattice structures or hollow sections instead of solid bulk where appropriate.
- Wärmemanagement beim Drucken: The intense energy input during metal AM creates significant thermal gradients. Design features to mitigate this: add sacrificial ribs or heat sinks in critical areas to draw heat away; ensure smooth transitions between thick and thin sections; orient the part to minimize large, flat surfaces parallel to the build plate. Build simulation software is invaluable here.
- Teil Konsolidierung: Examine the turbocharger assembly and identify opportunities to integrate adjacent components (brackets, mounts, heat shields) directly into the housing design. This reduces part count, assembly time, weight, and potential failure points (like bolted joints or welds).
- Design der Löcher: Vertical holes generally print with better roundness. Horizontal or angled holes often print slightly out-of-round (elliptical or teardrop-shaped) due to the layered construction. If tight tolerance is needed, design holes undersized for later machining or incorporate specific shapes (like teardrops) to improve as-built accuracy.
Engaging with an experienced additive Fertigung provider like Met3dp early in the design process can be highly beneficial. Their engineers possess deep knowledge of DfAM principles and can provide valuable feedback to optimize your turbocharger housing design for successful printing, optimal performance, and cost-effectiveness, leveraging advanced CAD for AM automotive Arbeitsabläufe.
Achieving Precision: Tolerance, Surface Finish, and Dimensional Accuracy in AM Housings
While metal additive manufacturing offers unparalleled design freedom, it’s crucial for engineers and procurement managers to have realistic expectations regarding the achievable precision directly from the printer. As-built AM parts generally do not possess the same tight tolerances and fine surface finish across all features as components fully machined from billet using multi-axis CNC centers. However, through careful process control, appropriate post-processing, and advanced quality assurance, precision metal 3D printing automotive components like turbocharger housings can meet demanding specifications.
Toleranzen:
- As-Built Tolerances: The typical dimensional accuracy achievable directly from Laser Powder Bed Fusion (LPBF) or Selective Electron Beam Melting (SEBM) processes often falls within the range of ±0.1mm to ±0.3mm oder ±0.1% to 0.2% of the nominal dimension, whichever value is larger. These values are general guidelines and can vary significantly based on the specific machine, material, part geometry, size, orientation, and process parameters used. Large, complex parts are inherently more challenging to keep within tight tolerances due to thermal effects.
- Erzielung engerer Toleranzen: For critical interfaces—such as turbine and compressor housing flanges, mating surfaces for the center housing rotating assembly (CHRA), V-band connections, or mounting points—as-built tolerances are often insufficient. These features typically require post-print CNC machining to achieve the necessary precision (e.g., ±0.025mm to ±0.05mm) for proper sealing, alignment, and assembly. DfAM plays a role here by including sufficient machining stock only on these critical features.
Surface Finish (Roughness Average – Ra):
- As-Built Oberflächenbeschaffenheit: The texture of an AM part’s surface is influenced by several factors:
- Pulver Partikelgröße: Finer powders generally yield smoother finishes.
- Schichtdicke: Thinner layers typically result in smoother surfaces, especially on sloped features.
- Orientierung: Vertical walls tend to be smoother than up-facing or down-facing surfaces. Down-facing surfaces, which rely on supports or the powder bed itself, are usually the roughest due to partially melted powder adhesion.
- Prozess: LPBF generally produces smoother as-built surfaces (e.g., Ra 6-20 µm) compared to SEBM (which might be Ra 20-35 µm or higher) due to differences in beam energy and powder size.
- Nachbearbeitetes Oberflächenfinish: As-built finishes are often too rough for sealing surfaces or optimal airflow in critical areas. Post-processing is used to improve the finish:
- Media Blasting: (e.g., bead, sand, grit) provides a uniform matte finish and removes loose powder (Ra might improve slightly or change texture).
- Taumeln/Gleitschleifen: Can smooth external surfaces but is less effective for internal channels.
- Bearbeitungen: Provides the best surface finish on specific features (Ra < 1.6 µm or better is achievable).
- Polieren: Can achieve very smooth, mirror-like finishes if required for specific aerodynamic or aesthetic reasons, but is labor-intensive.
Maßgenauigkeit und Qualitätskontrolle:
- Faktoren, die die Genauigkeit beeinflussen: Achieving consistent dimensional accuracy turbocharger components requires control over the entire workflow: machine calibration, optimized and validated process parameters, thermal management strategies (orientation, supports), minimizing residual stress, and accounting for minor distortions during post-print heat treatment.
- Importance of Quality Control (QC): Rigoros quality control metal additive manufacturing is essential. This involves:
- In-process Monitoring: Some advanced AM systems offer real-time monitoring of the melt pool and layer consistency.
- Inspektion nach der Fertigstellung: Hochauflösend 3D-Scannen is commonly used to compare the final part geometry against the original CAD model, generating detailed deviation maps.
- Coordinate Measuring Machine (CMM) Inspection: Used for precise measurement of critical dimensions, tolerances, and geometric dimensioning and tolerancing (GD&T) features, especially after final machining.
- Überprüfung der Materialeigenschaften: Testing tensile bars printed alongside the main part to confirm material properties meet specifications after heat treatment.
Met3dp’s Contribution to Precision: Met3dp understands the criticality of precision for demanding automotive applications. Their printers are engineered for branchenführendes Druckvolumen, Genauigkeit und Zuverlässigkeit. By combining robust machine design, advanced process control software, high-quality spherical metal powders, and stringent quality management systems, Met3dp enables customers to reliably produce dense, accurate turbocharger housings that meet the exacting standards required for mission-critical parts in high-performance vehicles.
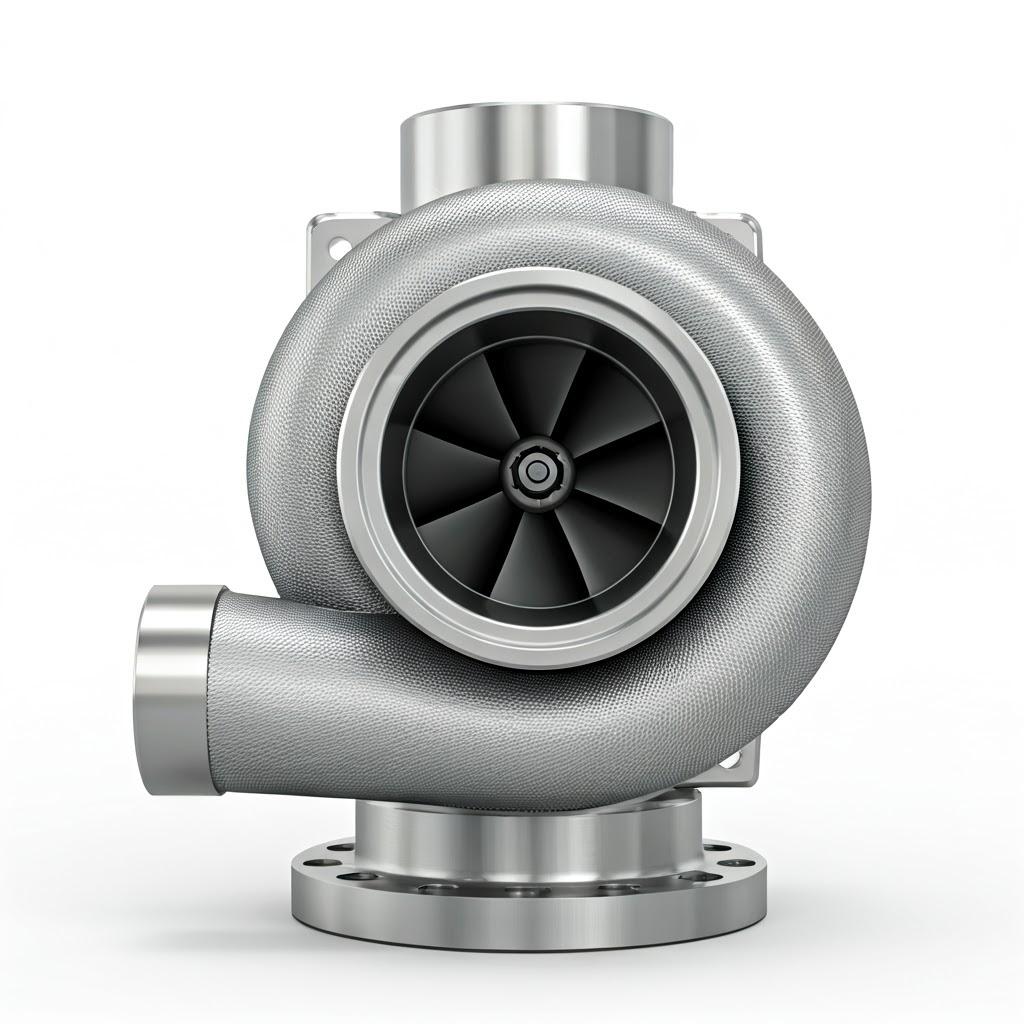
Beyond the Print: Essential Post-Processing for Turbocharger Housings
A common oversight in planning for metal additive manufacturing is underestimating the importance and extent of post-processing. Receiving the turbocharger housing off the 3D printer is a significant milestone, but it’s far from the final step. A series of essential post-processing operations are required to transform the raw printed part into a functional, reliable component ready for turbocharger housing assembly. These steps are critical for achieving the desired material properties, dimensional accuracy, surface finish, and overall integrity.
Typical Post-Processing Workflow for AM Turbo Housings (IN718/IN625):
- Stressabbau Wärmebehandlung:
- Warum? The rapid heating and cooling cycles inherent in AM create significant residual stresses within the printed part. These stresses can cause distortion or cracking during subsequent steps like support removal or machining, or even later in service. Stress relieving is typically the very first step after the build completes and the part cools.
- Wie? The part (often while still attached to the build plate) is heated in a controlled atmosphere furnace (vacuum or inert gas like Argon) to a specific temperature below the aging or annealing temperature, held for a period, and then slowly cooled. This allows internal stresses to relax without significantly altering the microstructure.
- Entfernen des Teils von der Bauplatte:
- Warum? Das Teil wird während des Drucks mit einer dicken Metallplatte verschmolzen.
- Wie? Typically done using wire Electrical Discharge Machining (EDM) or a band saw. Careful planning of the part’s connection to the plate (e.g., using minimal contact points or supports) can simplify this step.
- Entfernung der Stützstruktur:
- Warum? Supports are needed during the build but must be removed afterward.
- Wie? This can range from simple manual breaking/clipping for accessible supports to more involved Abstützung Metall AM techniques like grinding, milling, or EDM for tenacious or internal supports. The difficulty depends heavily on the material (Inconel supports are strong) and the effectiveness of DfAM in minimizing and optimizing support placement. Internal passages require careful inspection to ensure all support material and loose powder are removed.
- Final Heat Treatment (Solution Annealing / Aging):
- Warum? This step develops the final, desired mechanical properties of the superalloy. The required treatment depends on the specific alloy:
- Heat Treatment IN718 Parts: IN718 is typically precipitation-hardened. This involves solution annealing (heating to a high temperature to dissolve precipitates) followed by controlled cooling and then one or two aging steps at lower temperatures to precipitate the strengthening phases (gamma prime and gamma double-prime). Specific cycles (e.g., per AMS 5662/5663) are followed to achieve target hardness and strength.
- IN625: Is typically used in the solution-annealed condition for the best balance of properties in high-temperature corrosive environments. This involves heating to a high temperature (e.g., ~1150°C) and rapidly cooling to retain the strengthening elements (Mo, Nb) in solid solution. While not ‘aged’ like IN718, this annealing step (often combined with stress relief) is crucial.
- Wie? Performed in calibrated, atmosphere-controlled furnaces following precise time-temperature profiles defined by material specifications or aerospace/automotive standards.
- Warum? This step develops the final, desired mechanical properties of the superalloy. The required treatment depends on the specific alloy:
- CNC-Bearbeitung:
- Warum? To achieve the tight tolerances, specific surface finishes, and precise geometric features required for sealing and assembly interfaces.
- Wie? Using multi-axis CNC machining 3D printed components requires careful fixture design and programming. Key areas machined typically include:
- Turbine inlet and outlet flanges (flatness, Ra finish for gaskets/V-bands).
- Compressor inlet and outlet connections.
- Mating face for the Center Housing Rotating Assembly (CHRA).
- Wastegate valve seat or actuator interface.
- Mounting points and threaded holes.
- Any critical internal bore diameters.
- Machining stock should have been added in the DfAM phase specifically for these features.
- Oberflächenveredelung:
- Warum? To achieve a uniform appearance, remove contaminants, potentially improve fatigue life (shot peening), or achieve specific roughness targets on non-machined surfaces.
- Wie? Zu den gängigen Methoden gehören:
- Media Blasting: Bead blasting or grit blasting for a clean, matte finish (surface finishing AM automotive standard practice).
- Taumeln/Gleitschleifen: Can smooth external edges and surfaces.
- Manuelles Schleifen/Polieren: For specific requirements on localized areas.
- Reinigung und Inspektion:
- Warum? Ensure the part is free from debris, cutting fluids, and meets all specifications before assembly.
- Wie? Thorough cleaning processes. Final dimensional inspection (CMM/scanning), visual inspection, and potentially Non-Destructive Testing (NDT) like Fluorescent Penetrant Inspection (FPI) to check for surface cracks or Computed Tomography (CT) scanning to verify internal integrity and check for trapped powder, especially for critical applications.
Each of these post-processing steps adds time and cost but is indispensable for ensuring the 3D printed turbocharger housing performs reliably and meets the stringent requirements of the high-performance automotive sector. Partnering with a service provider offering integrated post-processing capabilities is often advantageous.
Navigating Challenges: Common Issues in 3D Printing Turbo Housings and Solutions
While metal additive manufacturing unlocks incredible possibilities for turbocharger housings, it’s an advanced process that comes with its own set of potential challenges. Awareness of these common issues, coupled with robust mitigation strategies, is key to successfully producing high-quality, reliable components. Experience and process expertise, such as that offered by Met3dp, are invaluable in navigating these complexities.
Gemeinsame Herausforderungen und Abhilfestrategien:
- Verformung und Verzerrung:
- Ausgabe: Significant thermal gradients during printing generate internal stresses (residual stress management AM). As layers cool and contract, these stresses can cause the part to warp, lift from the build plate, or distort from its intended geometry. This is particularly prevalent in warping metal AM parts with large flat areas or significant asymmetry.
- Lösungen:
- Build Simulation: Use process simulation software to predict stress accumulation and distortion patterns vor printing. Adjust orientation or support structures based on results.
- Optimierte Ausrichtung: Orient the part to minimize large cross-sections parallel to the build plate and balance thermal mass distribution.
- Robuste Unterstützungsstrategie: Use strategically placed supports (thermal and mechanical) to anchor the part firmly and conduct heat away effectively.
- Kontrolle der Prozessparameter: Optimize scan strategies (e.g., island scanning, alternating hatch directions) to distribute heat more evenly.
- Unmittelbarer Stressabbau: Perform stress relief heat treatment immediately after the build, often before removing the part from the build plate.
- Residual Stress and Cracking:
- Ausgabe: High residual stresses, if not properly managed, can exceed the material’s strength, leading to cracking during the print, during cooling, support removal, or even later in service life. Nickel-based superalloys, especially precipitation-hardenable ones like IN718 (cracking in Inconel printing), can be susceptible if process parameters and heat treatments are not carefully controlled. Thick sections and sharp internal corners exacerbate this risk.
- Lösungen:
- Wärmemanagement: Strategies mentioned above for warping also help manage residual stress.
- Optimized Heat Treatments: Implement scientifically validated stress relief and final heat treatment cycles specific to the alloy and AM process.
- DfAM: Design with generous fillet radii instead of sharp corners. Avoid abrupt changes in section thickness.
- Optimierung der Prozessparameter: Ensure stable melt pool dynamics and avoid excessive energy input in localized areas.
- Unterstützung bei der Beseitigung von Schwierigkeiten:
- Ausgabe: Supports made from high-strength superalloys can be very difficult and time-consuming to remove, especially from complex internal channels or intricate external features. Aggressive removal can damage the part surface. Inaccessible internal supports may be impossible to remove completely (support removal difficulties).
- Lösungen:
- DfAM: The most effective solution! Design for self-supporting angles (>45°). Orient the part to minimize the need for supports in critical or inaccessible areas. Design supports for easier removal (e.g., with specific contact points or structures).
- Specialized Support Structures: Utilize software features for easily removable support types (e.g., tree supports, block supports with perforation).
- Auswahl des Prozesses: SEBM often requires fewer supports than LPBF due to the higher process temperature maintaining parts above stress relaxation temperatures longer.
- Nachbearbeitungsplanung: Factor support removal methods (manual, machining, EDM) into the overall production plan.
- Kontrolle der Porosität:
- Ausgabe: Small voids or pores (porosity control 3D printing) can form within the printed material due to trapped gas (e.g., Argon used in LPBF chambers), incomplete fusion between layers, or keyholing (vapor depression collapse) caused by excessive energy density. Porosity can reduce mechanical properties, particularly fatigue strength.
- Lösungen:
- Qualität des Pulvers: Use high-quality, dry, spherical powder with optimized PSD (like Met3dp’s powders) to ensure good flowability and packing density.
- Optimierung der Parameter: Develop and validate robust process parameters (laser/beam power, speed, hatch spacing, layer thickness) to ensure complete melting and fusion.
- Atmosphärenkontrolle: Maintain high purity inert gas atmosphere (LPBF) or high vacuum (SEBM) to minimize gas entrapment.
- Heiß-Isostatisches Pressen (HIP): An optional post-processing step where parts are subjected to high temperature and isostatic pressure. This can effectively close internal pores, significantly improving density and fatigue properties, but adds cost and time.
- Surface Finish Roughness:
- Ausgabe: As-built surfaces, especially downward-facing slopes and surfaces directly supported by powder, can be rougher than desired for sealing or optimal flow dynamics.
- Lösungen:
- Orientierung: Prioritize critical surfaces for upward-facing or vertical orientations where possible.
- Einstellung der Parameter: Finer powders and thinner layers can improve finish but may increase print time.
- Nachbearbeiten: Plan for appropriate surface finishing steps (blasting, machining, polishing) as described in the previous section.
Successfully navigating these challenges requires a combination of robust DfAM practices, optimized and validated process parameters, high-quality materials, meticulous post-processing, and rigorous quality control. Partnering with an experienced AM service provider with deep expertise in processing superalloys for demanding applications is critical for achieving reliable, high-performance 3D printed turbocharger housings.
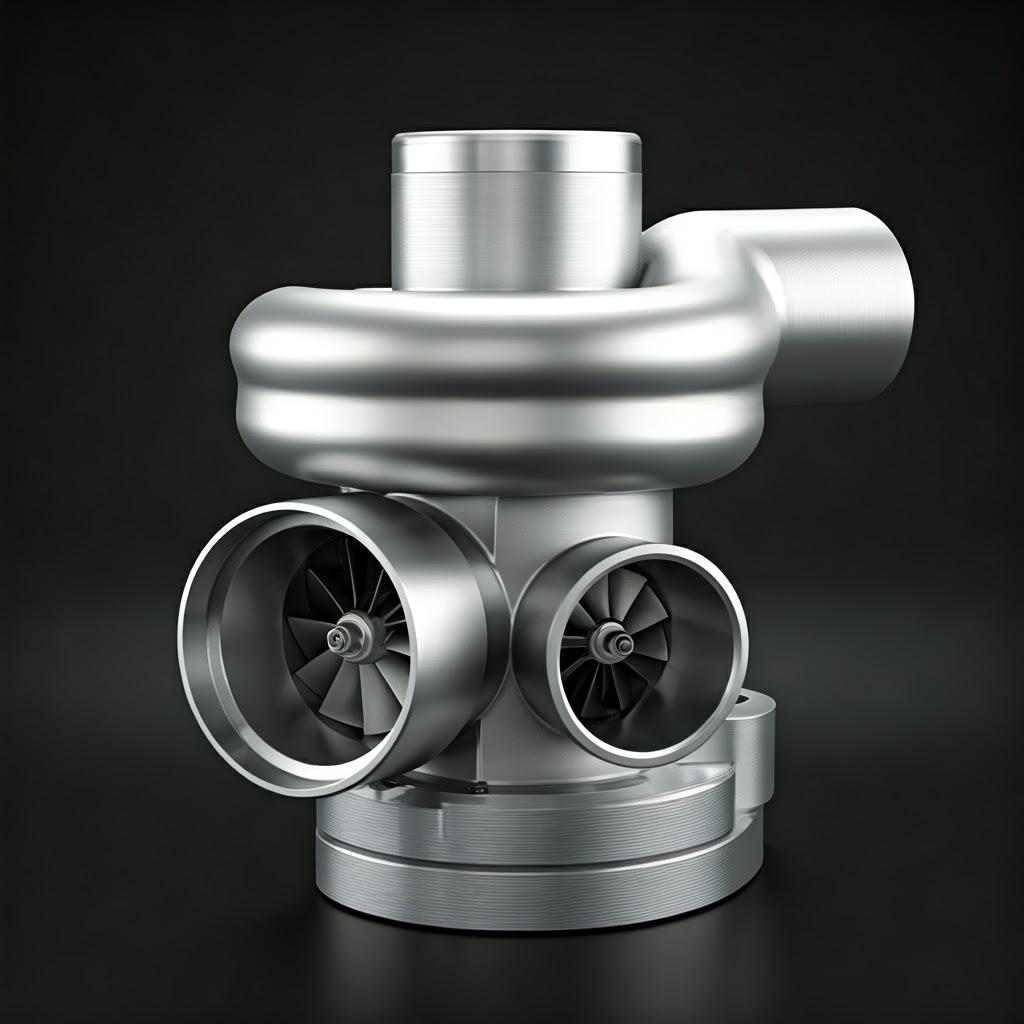
Supplier Selection: Choosing the Right Metal AM Partner for Automotive Components
Selecting a manufacturing partner is always a critical decision, but when dealing with advanced processes like metal additive manufacturing for high-performance automotive components, the choice demands extra diligence. The quality, reliability, and performance of your 3D printed turbocharger housing are directly linked to the capabilities and expertise of your chosen supplier. For engineers and procurement managers sourcing metal AM service provider automotive solutions, evaluating potential partners based on a comprehensive set of criteria is essential for mitigating risks and ensuring project success.
Key Criteria for Evaluating Metal AM Suppliers:
- Proven Industry & Application Experience: Does the supplier have demonstrable experience in the automotive sector, specifically with powertrain components or parts subjected to similar harsh environments (high temperature, high stress)? Ask for case studies, examples of past projects, or references related to high-performance turbochargers or similar demanding applications. Generic AM experience may not be sufficient.
- Material Expertise (IN718/IN625): Deep knowledge of processing nickel-based superalloys via AM is non-negotiable. Inquire about their experience with IN718 and IN625, their process parameters, how they validate material properties (e.g., tensile testing of witness coupons), and their powder handling procedures. Suppliers with material expertise additive manufacturing are crucial. Understanding their powder sourcing is also key – do they manufacture powder in-house, ensuring quality control, or rely solely on external suppliers?
- Technological Capabilities & Capacity: Assess their equipment portfolio. Do they operate well-maintained, industrial-grade LPBF or SEBM machines suitable for Inconel alloys? What are the build envelope sizes? Can they achieve the required accuracy and repeatability? Do they invest in keeping their technology current? Equally important is their capacity – can they meet your required Vorlaufzeit Metall-AM-Teile for prototypes and potential low-volume production runs?
- Qualitätsmanagementsystem und Zertifizierungen: This is paramount in automotive. Look for robust Quality Management Systems (QMS).
- ISO 9001: A baseline requirement for demonstrating quality processes.
- AS9100: While an aerospace standard, its rigorous focus on process control, traceability, and risk management is highly relevant and often adopted by suppliers serving high-performance automotive/motorsport.
- IATF 16949: The global automotive standard. While full certification might still be emerging among specialized AM providers, demonstrating adherence to its principles (e.g., process control, APQP, PPAP elements where applicable) is a significant advantage. Ask potential suppliers about their quality certifications AM.
- Integrierte Post-Processing-Funktionen: A turbocharger housing requires extensive post-processing. Can the supplier manage this entire workflow (stress relief, heat treatment to specification, precision CNC machining, surface finishing, NDT) either in-house or through a tightly controlled network of qualified partners? A single point of contact managing the entire process chain simplifies logistics and accountability.
- Engineering & DfAM Support: The best results come from collaboration. Does the supplier offer expert DfAM consultation? Can they help optimize your design for printability, performance, and cost-effectiveness? This partnership approach is often more valuable than a purely transactional relationship.
- Transparenz und Kommunikation: Evaluate their quoting process – is it clear and detailed? How do they handle project communication and updates? Are they responsive to technical queries? A transparent and communicative partner is easier to work with.
Warum sollte man Met3dp in Betracht ziehen?
Wenn evaluating 3D printing suppliers, Met3dp emerges as a compelling partner for demanding automotive applications. Headquartered in Qingdao, China, Met3dp offers a unique combination of strengths:
- Integrated Solutions Provider: Met3dp is not just a service bureau; they design and manufacture their own industry-leading printers known for accuracy, reliability, and suitability for mission-critical parts. Crucially, they also possess advanced in-house capabilities for producing high-quality spherical metal powders (including IN718, IN625, and innovative alloys) using Gas Atomization and PREP technologies. This vertical integration provides exceptional control over the core elements influencing final part quality.
- Umfassendes Fachwissen: With decades of collective expertise in metal additive manufacturing, Met3dp understands the nuances of processing challenging materials and complex geometries.
- Comprehensive Partnership: Beyond printing services, Met3dp provides comprehensive solutions including Anwendungsentwicklungsdienste, helping organizations implement AM effectively and accelerate their manufacturing transformations. They partner with clients to achieve specific engineering goals.
- Nachgewiesene Erfolgsbilanz: Met3dp focuses on delivering cutting-edge systems and powders for demanding fields like aerospace, medical, and automotive, demonstrating their capability in handling mission-critical components.
Choosing a supplier with proven Met3dp-Fähigkeiten means partnering with a company invested in the entire AM ecosystem, from material science to final part realization.
Understanding Investment: Cost Factors and Lead Times for AM Turbocharger Housings
For procurement managers and engineers planning projects involving 3D printed turbocharger housings, understanding the factors that drive cost and lead time is crucial for budgeting, planning, and comparing quotations effectively. While AM offers significant advantages, it involves different cost structures compared to traditional manufacturing. Providing clear information for B2B AM quoting ist unerlässlich.
Key Cost Drivers for AM Turbocharger Housings:
- Materialverbrauch:
- Teilband: The net volume of the final housing design.
- Unterstützungsstruktur Volumen: Supports consume material and must be factored in. Efficient DfAM minimizes this.
- Pulverkosten: High-performance superalloys like IN718 and IN625 are inherently expensive raw materials compared to common steels or aluminum alloys. Powder quality and production method also influence cost.
- Powder Refresh/Recycling: While unused powder can often be recycled, multiple uses can slightly alter its properties, requiring specific refresh strategies that impact overall material cost.
- Maschinenzeit: This is often the most significant cost factor.
- Bauhöhe: Print time is primarily determined by the number of layers (Z-height), not just the part’s volume or complexity within each layer. Taller parts take longer.
- Scan Time: The time taken by the laser or electron beam to scan each layer. Highly complex geometries might slightly increase this per-layer time.
- Maschinentarif: The hourly operating cost of the industrial metal AM system (includes energy, inert gas consumption, maintenance, depreciation).
- Build Plate Utilization (Density): Printing multiple parts simultaneously on a single build plate amortizes the fixed setup, heating, cooling, and part removal times across more units, reducing the cost per part. This is key for optimizing low-volume production runs.
- Support Structure Complexity: Intricate or dense supports not only consume more material but also require significantly more time and effort for removal during post-processing, adding labor costs.
- Nachbearbeitungsintensität: This is a major contributor to the final metal 3D printing cost automotive components. Each step adds cost:
- Stress relief and final heat treatments (furnace time, atmosphere control).
- Support removal labor/machining time.
- CNC machining (setup time, programming, machine time, tooling – potentially complex 5-axis machining).
- Surface finishing (blasting, tumbling, manual polishing).
- NDT and detailed inspections.
- Qualitätssicherungsanforderungen: The level of inspection specified (e.g., standard dimensional checks vs. full CMM report, surface FPI, internal CT scanning) directly impacts cost.
- Auftragsvolumen: While AM avoids tooling costs, there are still setup and programming efficiencies with larger batches. The price per part generally decreases for quantities moving from single prototypes to tens or low hundreds of units, though the curve is much flatter than for casting.
- Engineering/DfAM Support: If significant design optimization or consultation is required from the supplier, this engineering time may be factored into the initial project cost.
Überlegungen zur Vorlaufzeit:
Die lead time additive manufacturing parts involves more than just the print time:
- Vorverarbeitung: CAD file preparation, build simulation, slicing, build job setup (can take hours to days).
- Drucken: Highly variable based on part height and density (can range from 12 hours to several days for complex housings).
- Kühlung: Parts need to cool sufficiently within the machine before removal (several hours).
- Maschinenwarteschlange: Availability of the appropriate AM system at the supplier.
- Nachbearbeiten: Often the longest portion of the lead time. Heat treatments take hours/days per cycle; machining can take days depending on complexity and shop scheduling; finishing and inspection add more time (can collectively take 1-4+ weeks).
- Versand: Transitzeit zu Ihrer Einrichtung.
Typische Vorlaufzeitspannen:
- Prototypen: Often 1-4 weeks, depending on complexity and post-processing needs.
- Low-Volume Production (e.g., 10-50 units): Typically 4-8+ weeks, heavily influenced by post-processing requirements and batching efficiency.
Optimizing Cost & Lead Time:
- Leverage DfAM aggressively to reduce material usage and minimize support structures.
- Clearly define necessary tolerances and surface finishes only where critical to avoid unnecessary machining or polishing.
- Consolidate orders where possible to improve build plate density.
- Provide clear and complete technical specifications upfront to avoid delays.
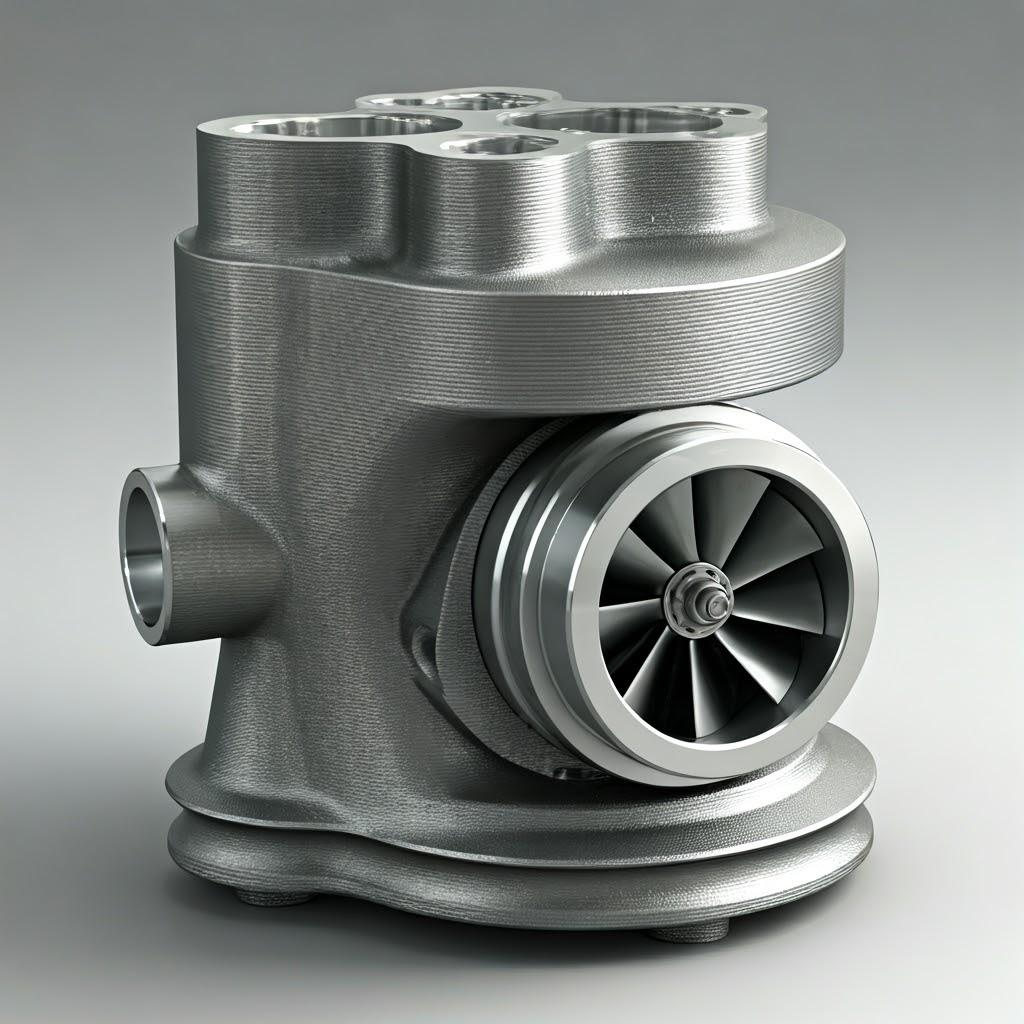
Frequently Asked Questions (FAQ) about 3D Printed Turbocharger Housings
As metal additive manufacturing gains traction in the automotive industry, engineers and procurement managers often have pertinent questions about its application for components like turbocharger housings. Here are answers to some common queries:
Q1: Are 3D printed turbocharger housings as reliable as traditionally manufactured ones?
A: Yes, absolutely, provided they are engineered and manufactured correctly. When designed using robust DfAM principles specifically for additive manufacturing, printed using validated processes on industrial-grade machines with high-quality superalloys like IN718 or IN625, and subjected to appropriate post-processing (especially heat treatment and machining of critical features) and rigorous quality control, 3D printed housings demonstrate excellent 3D printed turbocharger reliability. In many cases, due to optimized geometries (e.g., integrated cooling, superior flow paths) and the use of advanced materials, AM parts can offer superior performance and durability compared to their conventional counterparts, particularly under extreme operating conditions. Reliability is contingent on expertise throughout the entire design, manufacturing, and validation chain.
Q2: How does the cost of a 3D printed housing compare to casting for low volumes?
A: This is a crucial cost comparison AM automotive parts question. For prototypes, one-off custom parts, and low-volume production runs (typically ranging from single units up to perhaps 50-100 units, though the exact crossover point depends heavily on part complexity and casting method), metal AM is generally more cost-effective than traditional investment casting. This is primarily because AM eliminates the substantial upfront investment and long lead times associated with creating casting patterns and molds (tooling). As production volume increases into the hundreds or thousands, the per-part cost of casting drops significantly due to amortization of tooling, eventually making it cheaper than AM. However, it’s vital to consider the total value proposition: AM often enables performance levels, lightweighting, or design features unattainable with casting, which can justify a higher per-part cost in performance-driven applications. Furthermore, AM’s speed in delivering initial parts accelerates development cycles.
Q3: What kind of performance improvements can be expected from an optimized AM turbo housing?
A: Optimized AM housings can deliver tangible performance gains:
- Improved Aerodynamic Efficiency: Precisely crafted volutes and flow paths, designed using CFD and unconstrained by casting limitations, can improve both compressor and turbine efficiency. This translates to potentially faster spool-up (reduced lag), higher boost pressure capability, and increased engine power/torque across the operating range.
- Enhanced Thermal Management: Integrated cooling channels within the turbine housing walls can significantly lower material temperatures, improving durability, preventing heat soak, reducing the need for excessive exhaust heat shielding, and allowing for more consistent performance during prolonged high-load operation (e.g., track use).
- Gewichtsreduzierung: Topology optimization can reduce housing mass considerably compared to bulky cast designs, contributing to overall vehicle weight reduction – a critical factor in motorsport and performance vehicles.
- Optimized Packaging: Design freedom allows housings to be shaped to fit into tightly constrained engine bays, enabling more compact or efficient engine layouts.
Q4: Can existing turbocharger housing designs be directly 3D printed?
A: While you dürfen technically send a CAD file of a cast housing design to a 3D printer, it is strongly discouraged and generally yields poor results. Designs optimized for casting often feature uniform wall thicknesses, draft angles, and large radii suited for mold filling and part ejection – features that are unnecessary or even detrimental in AM. Directly printing such a design typically results in:
- Excessively heavy and bulky parts (missing lightweighting opportunities).
- Requirement for extensive internal and external support structures, increasing print time, material cost, and post-processing difficulty.
- Potential for higher residual stress and warping due to non-optimized geometry.
- Failure to leverage AM’s key advantages (e.g., internal channels, complex flow paths). To achieve the benefits discussed, redesigning the housing using DfAM principles is essential. Comparing AM vs cast turbo housing requires designing for each process’s strengths.
Conclusion: Driving Automotive Innovation with Additive Manufacturing
The landscape of high-performance automotive engineering is continually evolving, and metal additive manufacturing stands out as a powerful catalyst for innovation, particularly in the realm of forced induction. As we’ve explored, 3D printed turbocharger housings, fabricated from advanced superalloys like IN718 and IN625, offer a compelling package of benefits that directly address the extreme demands of motorsport, aftermarket tuning, and specialty vehicle applications.
Die unvergleichliche Gestaltungsfreiheit afforded by AM enables the creation of housings with optimized aerodynamics and integrated thermal management features previously impossible to achieve. This translates into tangible performance gains – faster response, higher efficiency, and enhanced durability under load. The ability to leverage Topologieoptimierung results in significantly lighter components, crucial for competitive advantage. Furthermore, AM facilitates schneller Prototypenbau and tooling-free Kleinserienfertigung, accelerating development cycles and providing a flexible manufacturing route for customized or niche components.
However, unlocking this potential requires more than just access to a 3D printer. Success hinges on a holistic approach encompassing expert Design für additive Fertigung (DfAM), meticulous process control, comprehensive Nachbearbeitung, rigorous qualitätssicherung, and crucially, partnering with the right metal AM solutions provider. Navigating the challenges of residual stress, support structures, and achieving precise tolerances necessitates deep expertise and experience.
Die future of turbocharger manufacturing for performance applications will undoubtedly see increasing adoption of additive manufacturing. For engineers seeking to push performance boundaries and procurement managers looking for cutting-edge, flexible manufacturing solutions for advanced automotive components, metal AM presents a strategic imperative.
If your organization is ready to explore the transformative potential of metal 3D printing for turbocharger housings or other demanding automotive components, consider partnering with a leader in the field. We invite you to Contact Met3dp to discuss your specific requirements. Leverage their comprehensive solutions—spanning industry-leading SEBM printers, advanced gas-atomized and PREP metal powders, and expert application development services—to power your organization’s additive manufacturing goals and accelerate your path to next-generation automotive innovation.
Teilen auf
Facebook
Twitter
LinkedIn
WhatsApp
E-Mail
MET3DP Technology Co., LTD ist ein führender Anbieter von additiven Fertigungslösungen mit Hauptsitz in Qingdao, China. Unser Unternehmen ist spezialisiert auf 3D-Druckgeräte und Hochleistungsmetallpulver für industrielle Anwendungen.
Fragen Sie an, um den besten Preis und eine maßgeschneiderte Lösung für Ihr Unternehmen zu erhalten!
Verwandte Artikel
Über Met3DP
Aktuelles Update
Unser Produkt
KONTAKT US
Haben Sie Fragen? Senden Sie uns jetzt eine Nachricht! Wir werden Ihre Anfrage mit einem ganzen Team nach Erhalt Ihrer Nachricht bearbeiten.
Holen Sie sich Metal3DP's
Produkt-Broschüre
Erhalten Sie die neuesten Produkte und Preislisten
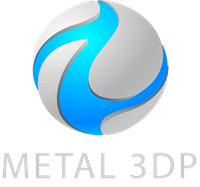
Metallpulver für 3D-Druck und additive Fertigung
UNTERNEHMEN
PRODUKT
cONTACT INFO
- Qingdao Stadt, Shandong, China
- [email protected]
- [email protected]
- +86 19116340731