3D Printed Enclosures for Heat Pipes in Space Systems
Inhaltsübersicht
Introduction: Revolutionizing Spacecraft Thermal Management with 3D Printed Heat Pipe Enclosures
The final frontier presents unparalleled challenges, not least of which is managing extreme temperatures. Spacecraft, whether orbiting Earth, exploring distant planets, or carrying human crews, operate in environments where temperatures can swing hundreds of degrees Celsius between direct sunlight and shadow. Effective thermal management isn’t just desirable; it’s mission-critical. Sensitive electronics, optical instruments, propulsion systems, and life support all depend on maintaining stable operating temperatures. Failure to do so can lead to performance degradation, component failure, and ultimately, mission loss. Among the sophisticated tools employed in spacecraft Thermal Control Systems (TCS), heat pipes stand out for their efficiency and reliability in passively transferring large amounts of heat over significant distances with minimal temperature drop. However, integrating these heat pipes effectively often requires complex enclosures or housings that provide structural support, protection, and optimized thermal interfaces. Traditionally, manufacturing these enclosures involved complex multi-part assemblies, extensive machining from billet materials, or intricate brazing processes – methods often limited by geometric constraints, long lead times, and significant material waste.
Der Einstieg in die additive Fertigung von Metall (AM), allgemein bekannt als Metall 3D-Druck. This transformative technology is rapidly changing how engineers design and manufacture components for the most demanding applications, including aerospace. By building parts layer by layer directly from digital models using high-performance metal powders, AM unlocks unprecedented design freedom. This allows for the creation of highly complex, lightweight, and functionally integrated heat pipe enclosures that were previously impossible or prohibitively expensive to produce. Metal 3D printing enables features like conformal cooling channels, intricate internal structures for optimized fluid flow (in loop heat pipes or CPLs often housed within enclosures), thin walls for mass reduction, and consolidated designs that combine multiple functions into a single component. This revolutionizes not only the component itself but also the entire system design, integration, and performance.
The benefits extend beyond mere geometry. Additive manufacturing facilitates rapid prototyping and design iteration, significantly shortening development cycles – a crucial advantage in the fast-paced aerospace sector. It allows engineers to test multiple design variations quickly, optimizing thermal performance and structural integrity based on simulation and empirical data. Furthermore, AM can utilize advanced materials specifically chosen for their thermal conductivity, strength-to-weight ratio, and resilience in the harsh space environment. This introduction explores the critical role of heat pipe enclosures in spacecraft thermal management and highlights how metal 3D printing is becoming an indispensable tool for creating next-generation, high-performance thermal solutions, paving the way for more capable and ambitious space missions. Companies like Met3dp, with their deep expertise in metal additive manufacturing technologies and high-quality material production, are at the forefront of this evolution, providing the capabilities needed to realize these advanced components.
Applications: Where are 3D Printed Heat Pipe Enclosures Deployed in Space Systems?
The application scope for 3D printed heat pipe enclosures within space systems is broad and continuously expanding as the technology matures and gains flight heritage. Their ability to offer tailored thermal performance, structural integration, and mass reduction makes them invaluable across various spacecraft platforms and subsystems. Procurement managers and engineers working for spacecraft thermal control system suppliers, satellite manufacturers, and aerospace component procurement departments are increasingly turning to AM for solutions that conventional methods cannot deliver efficiently.
Key Application Areas:
- Satellite Platforms (LEO, MEO, GEO):
- Electronics Cooling: Satellites are packed with high-power density electronics for communication payloads, data processing units, and power distribution systems. Heat pipe enclosures manufactured via AM can be designed to conform precisely to electronic component layouts, efficiently drawing heat away and radiating it to space via attached radiator panels. 3D printing allows for integrated mounting features and optimized thermal pathways within the enclosure itself, minimizing thermal resistance.
- Battery Thermal Management: Battery modules, especially during charge/discharge cycles, generate significant heat. Maintaining them within a narrow optimal temperature range is crucial for performance and lifespan. AM enclosures can incorporate complex internal structures or channels (for loop heat pipes) to uniformly cool battery arrays.
- Optical Payload Thermal Stability: Telescopes and sensitive optical instruments require exceptional thermal stability to maintain focus and alignment. 3D printed enclosures can house heat pipes that precisely control the temperature of detector arrays, lenses, and structural mounts, often using materials with low coefficients of thermal expansion (CTE) or tailored thermal conductivity.
- Structural Integration: In small satellites (CubeSats, SmallSats), where volume and mass are severely constrained, AM allows enclosures to serve dual purposes – providing thermal management via heat pipes und acting as primary or secondary structural elements, mounting points, or radiation shielding.
- Deep Space Probes and Rovers:
- Extreme Temperature Survival: Missions venturing into deep space or landing on celestial bodies face extreme hot and cold cycles. Heat pipes within robust AM enclosures help manage these temperature swings, keeping critical systems operational during long transits or harsh surface conditions (e.g., keeping electronics warm during lunar nights or Martian winters).
- Radiator Deployment Mechanisms: Enclosures might integrate with mechanisms that deploy larger radiator surfaces, using heat pipes to transport waste heat efficiently to these deployable elements. AM enables complex, lightweight hinges and structural interfaces within the enclosure.
- Instrument Thermal Control: Scientific instruments often have very specific and stable temperature requirements. Custom-designed AM enclosures housing heat pipes ensure precise thermal control for spectrometers, cameras, and sensors, enabling accurate data collection.
- Crewed Spacecraft and Space Stations:
- Life Support Systems: Environmental Control and Life Support Systems (ECLSS) involve managing heat generated by equipment and occupants. Heat pipe assemblies with AM enclosures contribute to efficient heat rejection, maintaining a habitable environment.
- Avionics Cooling: Complex avionics suites controlling navigation, guidance, and communication systems require robust thermal management. Conformal AM enclosures integrated with heat pipes provide efficient cooling solutions within tightly packed avionics bays.
- Experiment Modules: Scientific experiments conducted in microgravity often require precise temperature control. AM enclosures can be custom-built for specific experiment hardware, integrating heat pipes for stable thermal conditions.
B2B Context and Keywords:
For businesses operating in the aerospace supply chain, understanding these applications is key. Keywords relevant to B2B interactions include:
- Aerospace thermal management solutions supplier
- Spacecraft component manufacturer
- Satellite thermal control systems procurement
- Custom heat pipe assembly fabrication
- Additive manufacturing for aerospace structures
- High-performance thermal enclosures B2B
- Space-qualified component sourcing
- Metal 3D printing services for satellites
- Lightweight aerospace component supplier
The trend is clear: as spacecraft become more powerful and missions more ambitious, the demands on thermal control systems intensify. Traditional manufacturing approaches often struggle to meet the requirements for complexity, performance, and weight optimization. Metal 3D printing provides a powerful alternative, enabling the design and production of heat pipe enclosures that are specifically tailored to the unique challenges of each space mission. This capability positions AM as a critical enabler for future space exploration and commercialization, making partners proficient in this technology highly sought after by aerospace prime contractors and system integrators.
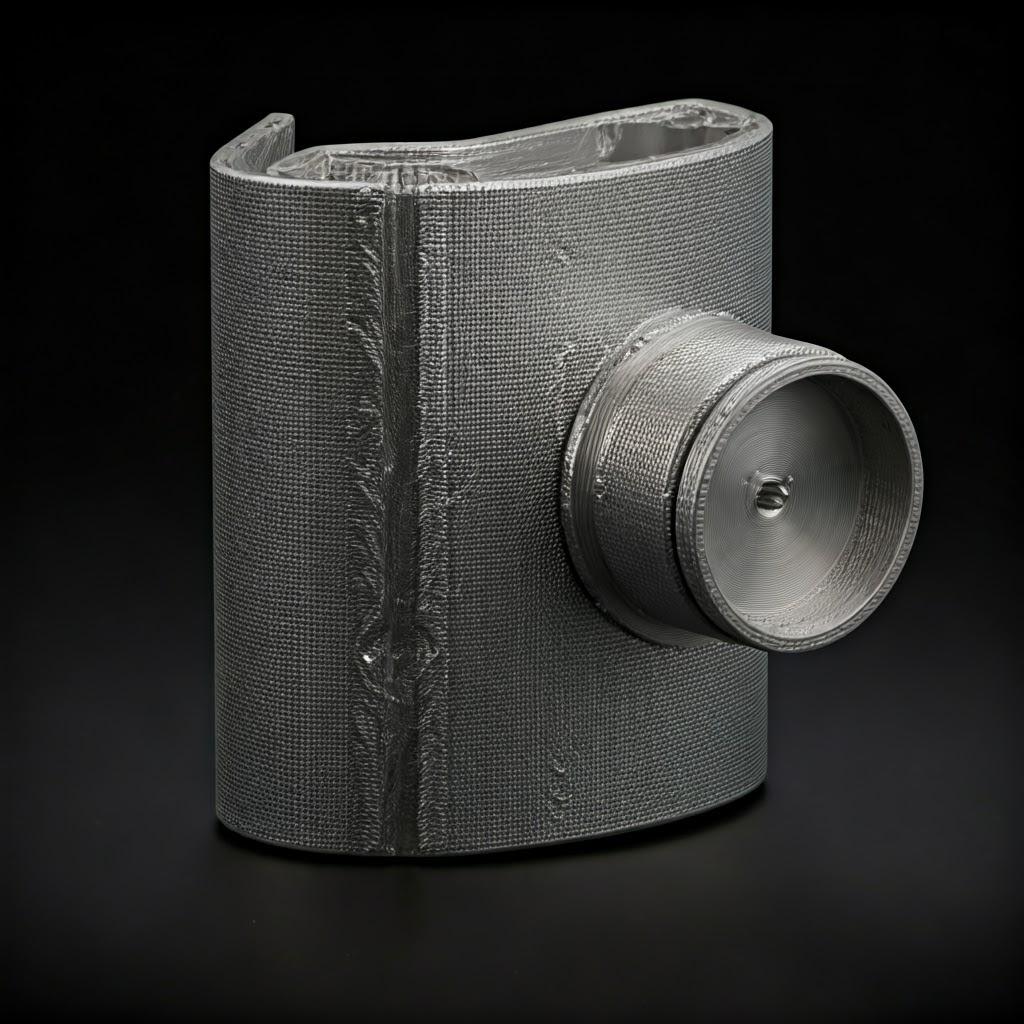
Why Metal Additive Manufacturing for Heat Pipe Enclosures?
The decision to utilize metal additive manufacturing for producing heat pipe enclosures in space systems stems from a confluence of compelling advantages that directly address the limitations of traditional manufacturing methods like machining, casting, or brazing/welding complex assemblies. These benefits translate into tangible improvements in performance, weight, cost, and development time, making AM an increasingly preferred choice for aerospace manufacturing solutions and advanced component fabrication.
Comparison: Metal AM vs. Traditional Manufacturing for Heat Pipe Enclosures
Merkmal | Traditional Manufacturing (Machining, Brazing, Casting) | Metal Additive Manufacturing (LPBF, SEBM) | Advantage for Heat Pipe Enclosures (Space) |
---|---|---|---|
Entwurfskomplexität | Limited by tool access, draft angles, joining methods. Complex internal features difficult/impossible. | Highly complex geometries, intricate internal channels, conformal shapes, organic forms easily achievable. | Enables optimized thermal pathways, integrated fluid channels (LHP/CPL), conformal designs fitting tight spaces, enhanced thermal performance. |
Teil Konsolidierung | Often requires multiple components assembled (brazing, welding, fasteners), increasing part count, weight, and potential failure points. | Ability to print complex, multi-functional components as a single piece. | Reduced part count, lower assembly labor, decreased weight, improved reliability (fewer joints), simplified supply chain. |
Gewichtsreduzierung | Material removal processes can be wasteful. Wall thickness limited by machining constraints. Achieving optimal topology is hard. | Material added only where needed. Enables topology optimization, lattice structures, thin walls, reducing mass significantly. | Critical for space applications where launch costs are high ($ thousands per kg). Improved payload capacity or reduced launch vehicle requirements. |
Materialnutzung | Subtractive methods (machining) generate significant material waste (swarf). Casting has gating/riser waste. | Additive process uses material primarily for the part itself. Powder reuse minimizes waste (though some support material is used). | More sustainable, reduced raw material costs, especially important for expensive aerospace-grade alloys. |
Lead Time (Prototyping) | Requires tooling, fixtures, multi-step processes. Iteration is slow and costly. | Direct digital manufacturing (CAD-to-part). Rapid prototyping and design iteration possible within days/weeks. | Accelerates development cycles, allows for faster testing and optimization of thermal designs, quicker response to design changes. |
Lead Time (Production) | Can be efficient for high volumes if tooling exists. Setup times can be long for complex parts. | Potentially slower per-part build time, but requires no tooling. Scalable by adding machines. Faster for low-to-mid volumes or highly complex parts. | Faster time-to-flight for unique or low-volume space components. Reduced inventory needs (on-demand manufacturing). |
Personalisierung | High cost and long lead times for custom or low-volume parts. | Ideal for producing unique or highly customized parts cost-effectively. | Enables mission-specific enclosure designs tailored to unique thermal loads, interfaces, and volume constraints without prohibitive tooling costs. |
Thermische Leistung | Limited by achievable geometries and joint resistances (in assemblies). | Complex internal features (fins, channels) and conformal designs optimize heat transfer. Reduced thermal resistance through part consolidation. | Enhanced heat dissipation, improved temperature uniformity, better overall thermal control system efficiency. |
Interne Merkmale | Extremely difficult or impossible to machine complex internal channels or structures. | Relatively easy to incorporate intricate internal cooling channels, porous structures (for wicks), or flow paths. | Essential for advanced heat pipe designs (e.g., Loop Heat Pipes, Capillary Pumped Loops) often integrated within enclosures. Improved wick structures. |
In Blätter exportieren
Specific Advantages Elaborated:
- Unerreichte Designfreiheit: AM liberates engineers from the constraints of traditional manufacturing. For heat pipe enclosures, this means designing complex internal passages that precisely follow heat sources, creating optimized fin structures within the enclosure for heat dissipation, or integrating mounting brackets, sensor ports, and structural reinforcements directly into the single printed part. This allows for enclosures that are perfectly conformal to the components they cool, minimizing thermal interface resistance and maximizing volumetric efficiency – crucial in tightly packed spacecraft.
- Signifikante Gewichtsreduzierung: Using techniques like topology optimization (removing material from non-critical areas while maintaining structural integrity) and incorporating internal lattice structures, AM can dramatically reduce the mass of heat pipe enclosures compared to machined counterparts. Every kilogram saved translates directly into lower launch costs or increased capacity for scientific instruments or fuel.
- Part Count Reduction: A complex heat pipe enclosure assembly might traditionally consist of a machined body, brazed or welded end caps, mounting flanges, and internal support structures. Metal AM can often consolidate these multiple parts into a single, monolithic component. This simplifies the supply chain, reduces assembly time and cost, eliminates potential leak paths or failure points at joints, and inherently improves structural integrity and thermal continuity.
- Beschleunigte Entwicklung: The ability to go directly from a CAD model to a physical metal part enables rapid iteration. Engineers can design an enclosure, print a prototype, test its thermal and structural performance, and quickly incorporate modifications into the next design iteration. This drastically shortens the development cycle compared to waiting for traditional tooling or machining setups, allowing for faster optimization and qualification of space hardware. Companies specializing in 3D-Druck von Metall services can further expedite this process with streamlined workflows.
- Enabling Advanced Thermal Designs: Certain advanced thermal management concepts, like Loop Heat Pipes (LHPs) or Capillary Pumped Loops (CPLs), rely on intricate internal evaporator and condenser structures with fine capillary wicks. Metal AM is uniquely capable of producing these complex internal geometries and porous wick structures directly within the enclosure body, something virtually impossible with conventional methods.
In summary, metal additive manufacturing offers a paradigm shift for designing and producing heat pipe enclosures for space systems. It moves beyond the limitations of subtractive and formative techniques, enabling the creation of lighter, more complex, better-performing, and rapidly developed thermal management solutions essential for the success of modern and future space missions.
Material Focus: AlSi10Mg and CuCrZr Powders for Optimal Performance
The choice of material is paramount when designing components for the demanding environment of space, and 3D printed heat pipe enclosures are no exception. The material must possess a suitable combination of thermal conductivity, mechanical strength, low density, resistance to the space environment (vacuum, radiation, thermal cycling), and compatibility with the chosen additive manufacturing process. For heat pipe enclosures, two metal powders stand out as particularly well-suited and are frequently recommended: Aluminum alloy AlSi10Mg und Copper alloy CuCrZr. The quality and characteristics of the metal powder used are fundamental to achieving the desired final part properties, making partnerships with expert powder manufacturers like Met3dp crucial. Met3dp utilizes industry-leading gas atomization and Plasma Rotating Electrode Process (PREP) technologies to produce high-sphericity, high-flowability metal powders optimized for AM processes like Laser Powder Bed Fusion (LPBF) and Selective Electron Beam Melting (SEBM).
1. Aluminum Alloy AlSi10Mg:
AlSi10Mg is a widely used aluminum alloy in additive manufacturing, known for its excellent combination of low density, good mechanical properties, good thermal conductivity (compared to other AM alloys like titanium or stainless steel), and excellent printability.
- Properties and Benefits:
- Geringe Dichte: ~2.67g/cm3. This is a primary driver for its use in aerospace, directly contributing to lightweighting and reduced launch costs.
- Gute Wärmeleitfähigkeit: Typically around 120−150W/(m⋅K) after appropriate heat treatment. While not as high as pure aluminum or copper, it’s sufficient for many heat transfer applications, especially where structural performance is also key.
- Good Mechanical Strength: Offers a good balance of tensile strength (up to 450MPa) and yield strength (up to 280MPa) after heat treatment (e.g., T6), providing necessary structural integrity for the enclosure.
- Ausgezeichnete Druckbarkeit: Melts and solidifies well during LPBF processes, allowing for the creation of complex geometries with fine features and relatively low residual stress compared to some other alloys.
- Korrosionsbeständigkeit: Exhibits good resistance to corrosion.
- Kosten-Nutzen-Verhältnis: Generally more cost-effective than titanium or high-performance copper alloys.
- Suitability for Heat Pipe Enclosures:
- Ideal für Anwendungen, bei denen lightweighting is the primary driver, and moderate thermal conductivity is acceptable.
- Suitable for structurally integrated enclosures that must bear mechanical loads in addition to housing the heat pipe.
- Often used for enclosures operating in moderate temperature ranges where the thermal loads are not extreme.
- Can be post-processed (machined, coated) to meet specific interface or surface requirements.
- Erwägungen:
- Thermal conductivity is lower than copper alloys.
- Mechanical properties decrease at elevated temperatures (above ~150-200°C).
- Requires appropriate heat treatment (solutionizing and aging) to achieve optimal mechanical properties.
2. Copper Alloy CuCrZr (Chromium Zirconium Copper):
CuCrZr is a high-performance copper alloy specifically designed to offer significantly higher thermal and electrical conductivity compared to aluminum alloys, while retaining good mechanical strength, especially at elevated temperatures.
- Properties and Benefits:
- Ausgezeichnete Wärmeleitfähigkeit: Typically exceeds 300W/(m⋅K) after heat treatment, making it vastly superior to AlSi10Mg for efficient heat transfer. This is crucial for minimizing temperature gradients and maximizing heat pipe performance.
- Hohe elektrische Leitfähigkeit: Also exhibits excellent electrical conductivity, which can be beneficial if the enclosure needs to provide electrical grounding or shielding.
- Good Mechanical Strength: Maintains good strength and hardness, particularly at elevated temperatures where aluminum alloys might soften. Tensile strength can reach 450−550MPa with appropriate heat treatment.
- Good High-Temperature Performance: Retains strength and resists softening at temperatures up to ~450-500°C.
- Gute Abriebfestigkeit: Offers better wear resistance compared to aluminum alloys.
- Suitability for Heat Pipe Enclosures:
- Die preferred choice for applications demanding maximum thermal performance and efficient heat dissipation, such as cooling high-power electronics or managing large thermal loads.
- Suitable for enclosures operating at higher temperatures where AlSi10Mg properties would degrade.
- Ideal for heat sinks or thermal spreaders integrated within the enclosure design.
- Erwägungen:
- Höhere Dichte: ~8.8−8.9g/cm3. Significantly heavier than AlSi10Mg, which impacts lightweighting efforts. The performance gain must justify the mass penalty.
- More Challenging Printability: Copper alloys have high reflectivity and thermal conductivity, making them more challenging to process reliably using LPBF compared to aluminum or steel. Requires optimized laser parameters, specific machine setups, and careful process control. Expertise from providers like Met3dp, experienced with diverse materials, becomes critical.
- Höhere Kosten: Copper alloy powders are generally more expensive than AlSi10Mg.
- Oxidation: Copper alloys can be more susceptible to oxidation, potentially requiring protective coatings or careful handling, although CuCrZr has reasonable resistance.
Selection Criteria & Powder Quality:
Choosing between AlSi10Mg and CuCrZr depends on the specific mission requirements, balancing thermal performance needs against mass constraints and budget.
- Prioritize AlSi10Mg if: Lightweighting is paramount, thermal loads are moderate, structural performance is key, and cost is a major factor.
- Prioritize CuCrZr if: Maximum heat transfer efficiency is critical, operating temperatures are high, and the mass penalty is acceptable or mitigated through optimized design.
Regardless of the alloy chosen, the quality of the metal powder is fundamental. Key powder characteristics influencing the final part quality include:
- Partikelgrößenverteilung (PSD): Affects powder bed density, flowability, and final part resolution and density.
- Sphärizität: Highly spherical powders flow better, leading to uniform powder layers and denser, more consistent parts.
- Fließfähigkeit: Crucial for uniform spreading of powder layers during the printing process.
- Purity/Chemistry: Strict control over alloy composition and minimizing impurities (like oxygen, nitrogen) is vital for achieving desired mechanical and thermal properties and ensuring process stability.
- Abwesenheit von Satelliten: Small particles attached to larger ones can hinder flowability and packing density.
Met3dp’s commitment to quality, utilizing advanced manufacturing techniques like vacuum induction melting gas atomization (VIGA) and PREP, ensures their metal powders meet the stringent requirements for aerospace applications. Their portfolio includes not only standard alloys but also capabilities for developing innovative materials tailored to specific customer needs. Ensuring a reliable supply of high-quality, aerospace-grade powder is the first critical step in successfully manufacturing demanding components like heat pipe enclosures. When exploring material options, consulting the detailed specifications available on resources like Met3dp’s product pages can provide valuable insights into the available powders and their certified properties.
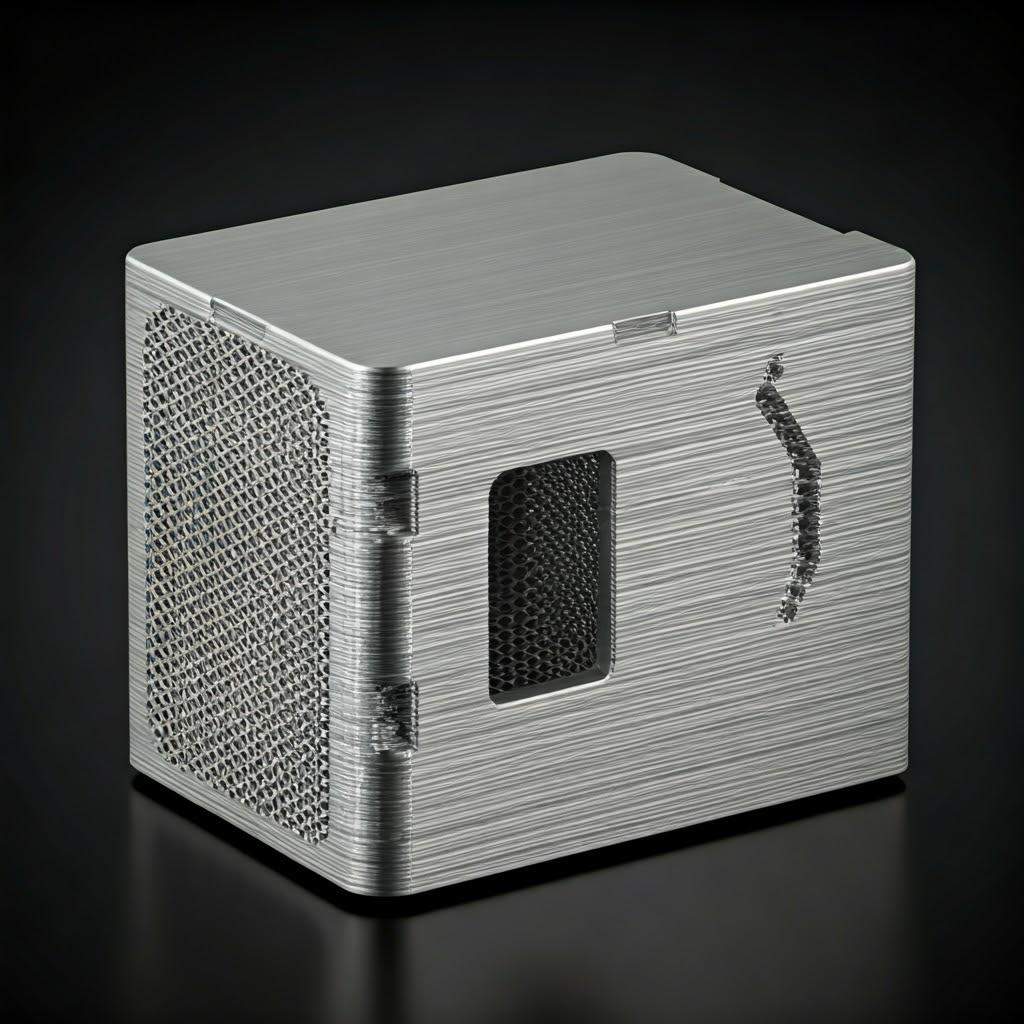
Design for Additive Manufacturing (DfAM): Optimizing Heat Pipe Enclosures for 3D Printing
Simply replicating a design intended for traditional manufacturing using additive manufacturing rarely yields optimal results. To truly harness the power of metal 3D printing for heat pipe enclosures in space systems, engineers must embrace Design für additive Fertigung (DfAM) principles. DfAM is not just a set of rules but a mindset shift, focusing on leveraging AM’s unique capabilities – complexity for free, part consolidation, lightweighting potential – while respecting its inherent constraints, such as support structures, build orientation, and minimum feature sizes. Optimizing a heat pipe enclosure using DfAM can lead to significant improvements in thermal performance, structural efficiency, mass reduction, and overall system integration.
Key DfAM Principles for Heat Pipe Enclosures:
- Topologie-Optimierung:
- Konzept: Software-driven process that mathematically optimizes material distribution within a defined design space, subject to given loads, boundary conditions, and constraints (e.g., maximizing stiffness for a given mass).
- Anwendung: For heat pipe enclosures, topology optimization can identify non-essential material that can be removed, resulting in organic, load-path-following structures that are significantly lighter than conventionally designed parts while meeting or exceeding structural requirements. This is paramount for reducing launch costs.
- Nutzen: Maximizes strength-to-weight ratio, creates highly efficient structures, ideal for generating initial lightweight concepts.
- Erwägung: Optimized shapes can be complex and may require careful consideration of manufacturability (e.g., overhangs needing support). Often needs some smoothing or interpretation for practical printing.
- Lattice Structures and Infill:
- Konzept: Replacing solid volumes with internal lattice structures (e.g., honeycomb, gyroid, truss-based) or patterned infill.
- Anwendung: Reduces mass while maintaining reasonable structural support. Certain lattice types (like TPMS – Triply Periodic Minimal Surfaces, e.g., gyroids) can also potentially enhance thermal performance by increasing surface area for heat transfer or influencing fluid flow within integrated channels (if applicable).
- Nutzen: Significant weight reduction, potential for tailored mechanical properties (e.g., energy absorption), possible thermal benefits.
- Erwägung: Complex lattices can be challenging to print without defects, may trap powder requiring careful design for drainage, and analysis can be computationally intensive. Surface roughness within lattices can be high.
- Conformal Design and Channel Optimization:
- Konzept: Designing features, especially internal channels or thermal pathways, to closely follow the contours of the components they interface with or the optimal path for heat/fluid flow.
- Anwendung: For heat pipe enclosures cooling electronics, internal passages or the external shape can conform precisely to the heat-generating components, minimizing thermal interface resistance. If housing loop heat pipes, the evaporator and condenser channels can be optimized for fluid dynamics and heat transfer, incorporating smooth bends and variable cross-sections impossible with drilling or milling.
- Nutzen: Improved thermal efficiency, reduced pressure drop (in fluid systems), better packaging density.
- Erwägung: Requires accurate modeling of interfacing components; complex internal channels necessitate robust powder removal strategies.
- Teil Konsolidierung:
- Konzept: Redesigning an assembly of multiple traditionally manufactured parts into a single, integrated component printable via AM.
- Anwendung: Combining the main enclosure body, mounting flanges, brackets, internal supports, and potentially even elements of the heat pipe wick structure (using porous AM techniques) into one monolithic part.
- Nutzen: Reduces part count, assembly time, weight, and potential failure points (joints, fasteners, welds, brazes). Improves structural and thermal continuity.
- Erwägung: Increases complexity of the single part; failure of the single part requires replacing the entire integrated component. Requires careful design to ensure all functional requirements of the original assembly are met.
- Wanddicke und Größe der Merkmale:
- Konzept: Designing walls and features appropriate for the specific AM process (e.g., LPBF, SEBM) and material being used.
- Anwendung: Ensuring walls are thick enough for structural integrity and printability (typically >0.4-0.5 mm for many processes, though specifics vary) but minimizing thickness where possible for weight reduction. Considering minimum resolvable feature sizes for small details like pins or thin fins.
- Nutzen: Balances structural performance, weight, and manufacturability. Prevents print failures due to features being too small or walls too thin.
- Erwägung: Optimal thickness depends on material, load case, thermal requirements, and printer capabilities. Very thin walls can be prone to distortion.
- Support Strategy and Build Orientation:
- Konzept: Designing the part and choosing its orientation in the build chamber to minimize the need for support structures, especially in critical or hard-to-reach areas, while ensuring printability. Overhanging features (typically below 45 degrees from horizontal) require support.
- Anwendung: Orienting the enclosure to minimize downward-facing surfaces or designing features to be self-supporting (e.g., using chamfers instead of sharp overhangs). Designing support structures that are easy to remove without damaging the part and minimizing contact points on critical surfaces.
- Nutzen: Reduces post-processing time and cost, improves surface finish on supported surfaces, lowers risk of part damage during removal.
- Erwägung: Build orientation affects residual stress, anisotropy (directional properties), and surface roughness on different faces. Internal supports within complex channels can be very difficult or impossible to remove, requiring careful DfAM planning (e.g., designing drainage holes, using soluble supports if available, or orienting to avoid internal overhangs).
- Integration des Wärmemanagements:
- Konzept: Directly integrating thermal management features like fins, pins, or optimized conductive pathways into the enclosure design.
- Anwendung: Designing external fins directly onto the enclosure surface to increase surface area for radiation to space. Creating internal structures or optimized solid pathways to efficiently conduct heat from the source to the heat pipe evaporator section or from the condenser section to a radiator interface.
- Nutzen: Enhanced thermal performance, reduced thermal resistance, potential elimination of separate heat sinks or spreaders.
- Erwägung: Complex fins increase print time and potentially the need for supports. Thermal performance needs to be validated through simulation and testing.
DfAM Workflow:
A typical DfAM workflow involves:
- Defining Requirements: Clearly specifying thermal loads, structural constraints, interfaces, mass targets, environmental conditions.
- Conceptual Design: Utilizing topology optimization, generative design, or expert knowledge to create initial AM-centric concepts.
- Detaillierter Entwurf und Simulation: Refining the geometry using CAD tools, incorporating DfAM principles (wall thickness, supports, etc.). Performing thermal and structural Finite Element Analysis (FEA) to validate performance.
- Manufacturability Checks: Using specialized software or checklists to ensure the design is printable, supports are feasible, and powder can be removed. Consulting with the AM service provider (like Met3dp) early in the design phase is highly recommended.
- Iteration: Refining the design based on simulation results and manufacturability feedback.
By thoughtfully applying DfAM, engineers can unlock the full potential of metal 3D printing, creating heat pipe enclosures that are not just manufactured differently, but are fundamentally better – lighter, more efficient, and perfectly tailored to the extreme demands of space.
Achieving Precision: Tolerance, Surface Finish, and Dimensional Accuracy in AM Enclosures
While metal additive manufacturing enables incredible geometric complexity, understanding and controlling the achievable precision – encompassing dimensional accuracy, tolerances, and surface finish – is critical for functional space hardware like heat pipe enclosures. Interfaces must align perfectly, seals must function correctly, and thermal contact resistance must be minimized. The capabilities of the AM process itself, the chosen material, the part’s design, and subsequent post-processing steps all influence the final precision.
Maßgenauigkeit und Toleranzen:
- As-Built-Genauigkeit: Metal AM processes like Laser Powder Bed Fusion (LPBF) and Selective Electron Beam Melting (SEBM) typically achieve dimensional accuracies in the range of ±0.1 mm to ±0.2 mm for smaller features (e.g., up to 100 mm), or ±0.1% to ±0.2% of the nominal dimension for larger parts. However, this can be influenced by factors like:
- Thermische Effekte: Residual stress accumulation during printing can cause warping or distortion, impacting overall accuracy. Part design, support strategy, and build parameters play a crucial role.
- Material: Different materials exhibit varying degrees of shrinkage and stress accumulation (e.g., CuCrZr can be more challenging than AlSi10Mg).
- Kalibrierung der Maschine: The accuracy and calibration of the specific AM system are fundamental. High-end industrial printers, like those potentially used by providers such as Met3dp known for industry-leading accuracy and reliability, generally offer better consistency.
- Teilegeometrie und -ausrichtung: Large flat sections or unsupported overhangs are more prone to deviation.
- Achievable Tolerances: While the general accuracy provides a baseline, tighter tolerances on specific critical features (e.g., mounting holes, interface surfaces, sealing grooves) are often required.
- Wie gedruckt: Tolerances tighter than the general accuracy often require careful process control and potentially feature compensation in the design file. Achieving tolerances below ±0.1 mm directly from printing can be challenging and inconsistent across the entire part.
- Post-Machined: For features demanding high precision (e.g., ±0.01 mm to ±0.05 mm), post-process machining (CNC milling, turning, grinding) is typically necessary. Critical interfaces, sealing surfaces, and precise hole diameters/locations are commonly finished using subtractive methods after printing.
Oberflächengüte (Rauhigkeit):
Surface finish, often quantified by the average roughness (Ra), is another key aspect of precision, particularly important for thermal contact resistance and sealing.
- As-Built Surface Roughness:
- Obere Oberflächen: Surfaces facing upwards during the build generally have the lowest roughness, often Ra 5-15 µm, depending on powder size and layer thickness.
- Vertikale Mauern: Side walls tend to have slightly higher roughness due to the layer-wise nature, typically Ra 10-20 µm.
- Supported Surfaces: Downward-facing surfaces that required support structures exhibit the highest roughness, often Ra 20-50 µm or more, depending on the support type and removal process. The points where supports attach leave witness marks that often need further finishing.
- Interne Kanäle: Roughness inside complex internal channels can be high and difficult to improve significantly without specialized techniques (e.g., abrasive flow machining).
- Impact on Performance:
- Thermal Contact Resistance (TCR): Rough surfaces create microscopic gaps when two components are joined, trapping air (a poor conductor) and significantly increasing resistance to heat flow across the interface. For heat pipe enclosures mating with heat sources or radiators, minimizing TCR is critical. Smooth surfaces (low Ra) achieved through machining or polishing are often required at these interfaces.
- Versiegeln: Rough surfaces can compromise the effectiveness of seals (e.g., O-rings, metallic seals), leading to potential leaks – unacceptable for contained fluids or maintaining vacuum integrity. Sealing surfaces almost always require post-process machining or polishing.
- Radiative Properties: Surface roughness affects the emissivity and absorptivity of the enclosure’s external surfaces, influencing its ability to radiate heat to space. While coatings are often used to precisely control these properties, the underlying roughness can play a role.
- Fluid Flow: High roughness in internal channels (e.g., in LHPs) can increase pressure drop.
- Verbesserung der Oberflächengüte: Common post-processing methods include:
- Machining (Milling, Turning): Provides very smooth, precise surfaces (Ra < 1 µm possible).
- Polieren: Mechanical or electro-chemical polishing for very low Ra values.
- Abrasives Strahlen (Sandstrahlen, Perlstrahlen): Creates a uniform matte finish, can remove minor imperfections but typically increases Ra compared to machining.
- Abrasive Fließbearbeitung (AFM): Used to smooth internal channels by flowing an abrasive putty through them.
- Mass Finishing (Tumbling): Can smooth external surfaces and deburr edges, but less precise control.
Achieving Precision – A Holistic Approach:
Ensuring the required precision for a 3D printed heat pipe enclosure involves:
- Entwurf: Designing critical features with machining stock allowance if tight tolerances or smooth finishes are needed. Avoiding complex internal supports where possible.
- Material & Process Selection: Choosing the appropriate AM process and material, understanding their inherent accuracy and roughness capabilities.
- Optimierung der Parameter: Working with an experienced AM provider to optimize build parameters for density, accuracy, and minimal stress.
- Nachbearbeitungsplan: Defining necessary machining, polishing, or other finishing steps for critical features during the design phase.
- Qualitätskontrolle: Implementing rigorous inspection methods (CMM measurements, surface profilometry, CT scanning) to verify dimensions and surface characteristics.
By carefully considering these factors, engineers can confidently leverage metal AM to produce heat pipe enclosures that meet the stringent precision requirements of space applications.
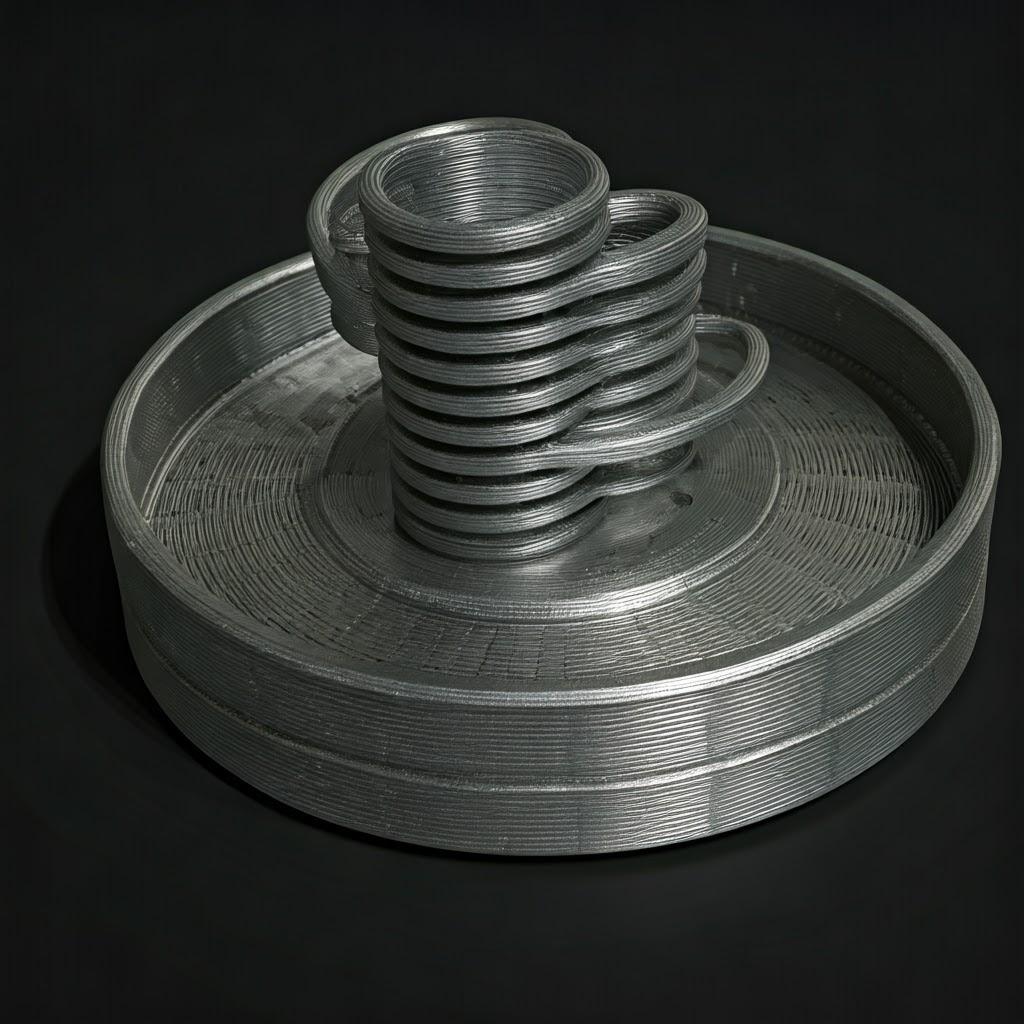
Beyond the Print: Essential Post-Processing for Space-Grade Enclosures
The journey of a 3D printed metal heat pipe enclosure doesn’t end when it comes out of the printer. The “as-built” part requires several crucial post-processing steps to transform it from a raw print into a flight-ready, functional component. These steps are essential to relieve stress, achieve the required dimensional accuracy and surface finish, ensure material properties meet specifications, and guarantee cleanliness for the space environment. Omitting or improperly performing these steps can compromise the part’s integrity and performance.
Common Post-Processing Steps for AM Heat Pipe Enclosures:
- De-powdering / Cleaning (Initial):
- Zweck: Remove the bulk of unfused metal powder surrounding and potentially trapped within the part after the build is complete.
- Methoden: Manual brushing, compressed air blow-off, vibration, bead blasting (carefully, to avoid surface damage). For complex internal channels, specialized powder removal systems or careful manipulation may be needed.
- Wichtigkeit: Residual powder can interfere with subsequent steps (heat treatment, machining), add weight, and potentially become loose contamination later. Complete powder removal from intricate internal geometries is a significant challenge requiring careful DfAM.
- Stressabbau Wärmebehandlung:
- Zweck: Relieve internal stresses built up during the rapid heating and cooling cycles of the layer-by-layer AM process. These stresses can cause distortion (warpage) after the part is removed from the build plate or during subsequent machining, and can negatively impact mechanical properties.
- Methoden: Heating the part (often while still attached to the build plate) in a controlled atmosphere furnace to a specific temperature below the alloy’s aging or melting point, holding it for a set time, and then slowly cooling it. Parameters (temperature, time, atmosphere) are specific to the alloy (AlSi10Mg, CuCrZr).
- Wichtigkeit: Mandatory step for almost all metal AM parts, especially those with complex geometries or tight tolerances, to ensure dimensional stability and prevent cracking.
- Entfernen von der Bauplatte und Entfernen der Stützstruktur:
- Zweck: Separate the printed part(s) from the base plate they were built upon and remove the support structures used to anchor the part and prevent overhangs from collapsing during printing.
- Methoden:
- Build Plate Removal: Wire EDM (Electrical Discharge Machining), sawing, or machining.
- Unterstützung bei der Entfernung: Manual breaking/cutting (for easily accessible supports), CNC machining, grinding, wire EDM (for delicate or hard-to-reach supports).
- Wichtigkeit: Supports are necessary for printing but are sacrificial. Removal must be done carefully to avoid damaging the part surface. Areas where supports were attached will typically have a rougher surface finish and require further treatment. Ease of support removal should be a key DfAM consideration.
- Further Heat Treatment (Solutionizing & Aging / Annealing):
- Zweck: Optimize the material’s microstructure to achieve the desired final mechanical properties (strength, ductility, hardness) and thermal conductivity.
- Methoden:
- AlSi10Mg: Typically requires a T6 heat treatment involving solutionizing (heating to dissolve Si and Mg into the Al matrix) followed by quenching and artificial aging (lower temperature heating to precipitate strengthening phases).
- CuCrZr: Often requires solution annealing followed by quenching and precipitation hardening (aging) to maximize strength and conductivity.
- Parameter: Specific temperatures, hold times, quench rates, and aging parameters are critical and depend on the alloy and desired properties, often following aerospace specifications. Controlled atmosphere or vacuum furnaces are typically required to prevent oxidation.
- Wichtigkeit: Essential for meeting the performance requirements specified in the design. As-printed or only stress-relieved parts generally do not possess the optimal material properties.
- Spanende Bearbeitung (CNC):
- Zweck: Achieve tight tolerances on critical dimensions, create precise interface surfaces (for mating parts, thermal contact), produce smooth sealing surfaces, and machine features that are difficult or impossible to print accurately (e.g., threaded holes).
- Methoden: Milling, turning, grinding, drilling, tapping.
- Wichtigkeit: Bridges the gap between AM’s geometric freedom and the high precision often required for functional interfaces. Essential for ensuring proper assembly, sealing, and efficient thermal transfer at contact points. Requires careful fixture design to hold the potentially complex AM part securely without distortion.
- Oberflächenveredelung:
- Zweck: Improve surface roughness for thermal, sealing, or aesthetic reasons; deburr edges; prepare surfaces for coating; or apply specific surface characteristics (e.g., controlled emissivity/absorptivity).
- Methoden: Abrasive blasting (various media), tumbling/mass finishing, mechanical polishing, electro-polishing, micro-machining, potentially anodizing (for aluminum alloys), or application-specific coatings (e.g., thermal control coatings like white paint, black paint, optical solar reflectors, or specialized low-outgassing aerospace coatings).
- Wichtigkeit: Tailors the surface properties to meet functional requirements beyond basic geometry and dimensional accuracy. Coatings are very common for thermal control surfaces in space.
- Final Cleaning & Inspection:
- Zweck: Remove any residual machining fluids, polishing compounds, debris, or handling contamination to meet stringent aerospace cleanliness standards (preventing outgassing or particulate contamination in space). Perform final quality verification.
- Methoden: Multi-stage cleaning processes (ultrasonic baths with specific solvents/detergents), precision cleaning protocols, final inspection under magnification or using specialized equipment. Non-Destructive Testing (NDT) is crucial:
- Computertomographie (CT) Scannen: Detects internal voids, porosity, cracks, and verifies complex internal geometries and powder removal.
- Prüfung der Abmessungen: Coordinate Measuring Machine (CMM) for verifying critical dimensions and tolerances.
- Messung der Oberflächenrauhigkeit: Profilometry to confirm surface finish requirements.
- Materialprüfung: Tensile testing, hardness testing, chemical analysis on witness coupons printed alongside the part.
- Pressure/Leak Testing: If the enclosure is designed for containment.
- Wichtigkeit: Ensures the final part is free from defects, meets all specifications, and is safe for flight.
The specific sequence and necessity of these steps depend heavily on the part design, material, and application requirements. Partnering with a knowledgeable AM service provider experienced in aerospace applications is vital to ensure all necessary post-processing is identified, planned, and executed correctly to deliver a reliable, space-qualified heat pipe enclosure.
Navigating Challenges: Overcoming Hurdles in 3D Printing Heat Pipe Enclosures
While metal additive manufacturing offers transformative advantages for creating complex heat pipe enclosures, the technology is not without its challenges, especially when producing high-stakes components for space systems. Understanding these potential hurdles and implementing effective mitigation strategies is crucial for successful adoption. Experienced AM providers develop robust processes to address these common issues.
Gemeinsame Herausforderungen und Abhilfestrategien:
- Eigenspannung und Verzug:
- Herausforderung: The rapid, localized heating and cooling inherent in powder bed fusion processes create significant thermal gradients, leading to internal stresses within the printed part. These stresses can cause distortion (warpage) during or after the build, especially in large or geometrically complex parts, compromising dimensional accuracy.
- Milderung:
- Simulation: Using process simulation software to predict stress accumulation and distortion, allowing for design or parameter adjustments.
- Strategie aufbauen: Optimizing part orientation, using appropriate laser/electron beam scanning strategies (e.g., island scanning, sectoring) to manage heat input.
- Unterstützende Strukturen: Designing robust support structures to anchor the part securely to the build plate and counteract warping forces.
- Wärmemanagement: Preheating the build plate (common in LPBF, inherent in SEBM) reduces thermal gradients.
- Obligatorischer Stressabbau: Performing a stress relief heat treatment cycle immediately after printing and often before removing the part from the build plate is essential.
- Porosität:
- Herausforderung: Small voids or pores can form within the printed material due to trapped gas (gas porosity) or incomplete melting/fusion between layers or scan tracks (lack-of-fusion porosity). Porosity degrades mechanical properties (especially fatigue life), can reduce thermal conductivity, and may act as crack initiation sites. Aerospace standards typically have very strict limits on allowable porosity.
- Milderung:
- Optimierung der Parameter: Developing and meticulously controlling process parameters (laser/beam power, scan speed, layer thickness, hatch spacing) specific to the material (AlSi10Mg, CuCrZr) and machine to achieve >99.5% density (often >99.9%). This requires significant expertise.
- Qualität des Pulvers: Using high-quality, dry, spherical powder with controlled particle size distribution minimizes gas entrapment. Proper powder handling and storage are critical.
- Prozessüberwachung: Employing in-situ monitoring tools (e.g., melt pool monitoring) to detect potential anomalies during the build.
- Heiß-Isostatisches Pressen (HIP): A post-processing step where the part is subjected to high temperature and high inert gas pressure. HIP can effectively close internal pores (except surface-connected ones), significantly improving density and mechanical properties. Often required for critical aerospace components.
- Entfernung der Stützstruktur:
- Herausforderung: While necessary, support structures must be removed. This can be time-consuming and costly, especially for complex internal supports within channels or hard-to-reach areas. Aggressive removal can damage the part surface, while incomplete removal leaves undesirable protrusions or potential contamination traps.
- Milderung:
- DfAM: Designing parts to be self-supporting where possible, minimizing overhang angles, and designing supports for easier access and removal (e.g., using lower-density, easily breakable supports where appropriate).
- Orientierung: Choosing build orientations that minimize the need for critical internal supports.
- Erweiterte Entfernungstechniken: Utilizing methods like wire EDM or specialized machining for delicate or inaccessible supports.
- Soluble/Chemically Removable Supports: Research is ongoing, but less common for structural metal AM currently.
- Validierung und Konsistenz von Materialeigenschaften:
- Herausforderung: Ensuring that the mechanical, thermal, and chemical properties of the final printed and post-processed part consistently meet the stringent specifications required for space applications. Properties can be influenced by build parameters, location within the build chamber, powder batch variations, and heat treatment cycles. Anisotropy (properties varying with build direction) can also occur.
- Milderung:
- Rigorous Process Control: Maintaining strict control over all process variables (machine calibration, parameters, powder quality, atmosphere, heat treatment).
- Gutscheine bezeugen: Printing standardized test coupons alongside the actual parts in each build. These coupons undergo destructive testing (tensile tests, hardness tests, metallography, chemical analysis) to verify the properties achieved in that specific build cycle.
- Database Development: Building a statistical database of material properties based on consistent process parameters.
- Qualification Protocols: Following established aerospace qualification procedures (e.g., process qualification, part qualification) involving extensive testing.
- Contamination Control:
- Herausforderung: Metal AM processes, especially powder handling, require clean environments. Contamination from foreign particles, moisture, or cross-contamination between different powder types can lead to defects in the final part or compromise its performance in the vacuum of space (e.g., outgassing).
- Milderung:
- Dedicated Equipment: Using machines dedicated to specific materials or material families (e.g., one machine for aluminum, another for copper).
- Strict Powder Handling: Implementing rigorous procedures for powder storage, loading, sieving, and recycling in controlled environments (e.g., inert gas glove boxes).
- Build Chamber Environment: Maintaining high purity inert gas (Argon, Nitrogen) atmosphere within the build chamber to prevent oxidation.
- Cleaning Protocols: Thorough cleaning of parts after printing and post-processing to meet aerospace cleanliness standards.
- Surface Finish Limitations:
- Herausforderung: As-built surface roughness, especially on supported surfaces or internal channels, may not meet the requirements for thermal interfaces, sealing, or fluid flow without significant post-processing effort.
- Milderung:
- DfAM & Orientation: Designing and orienting parts to place critical surfaces in orientations that naturally produce better finishes (e.g., upward-facing or vertical).
- Targeted Post-Processing: Incorporating necessary machining, polishing, or AFM steps into the manufacturing plan specifically for critical surfaces.
- Realistic Expectations: Understanding the inherent roughness limitations of the chosen AM process and planning accordingly.
Successfully navigating these challenges requires a deep understanding of materials science, AM process physics, DfAM principles, and robust quality management systems. Partnering with an established metal AM provider like Met3dp, which possesses decades of collective expertise and utilizes advanced equipment and powder manufacturing technologies like their various printing methods, significantly de-risks the process and increases the likelihood of producing high-quality, reliable heat pipe enclosures for demanding space applications.
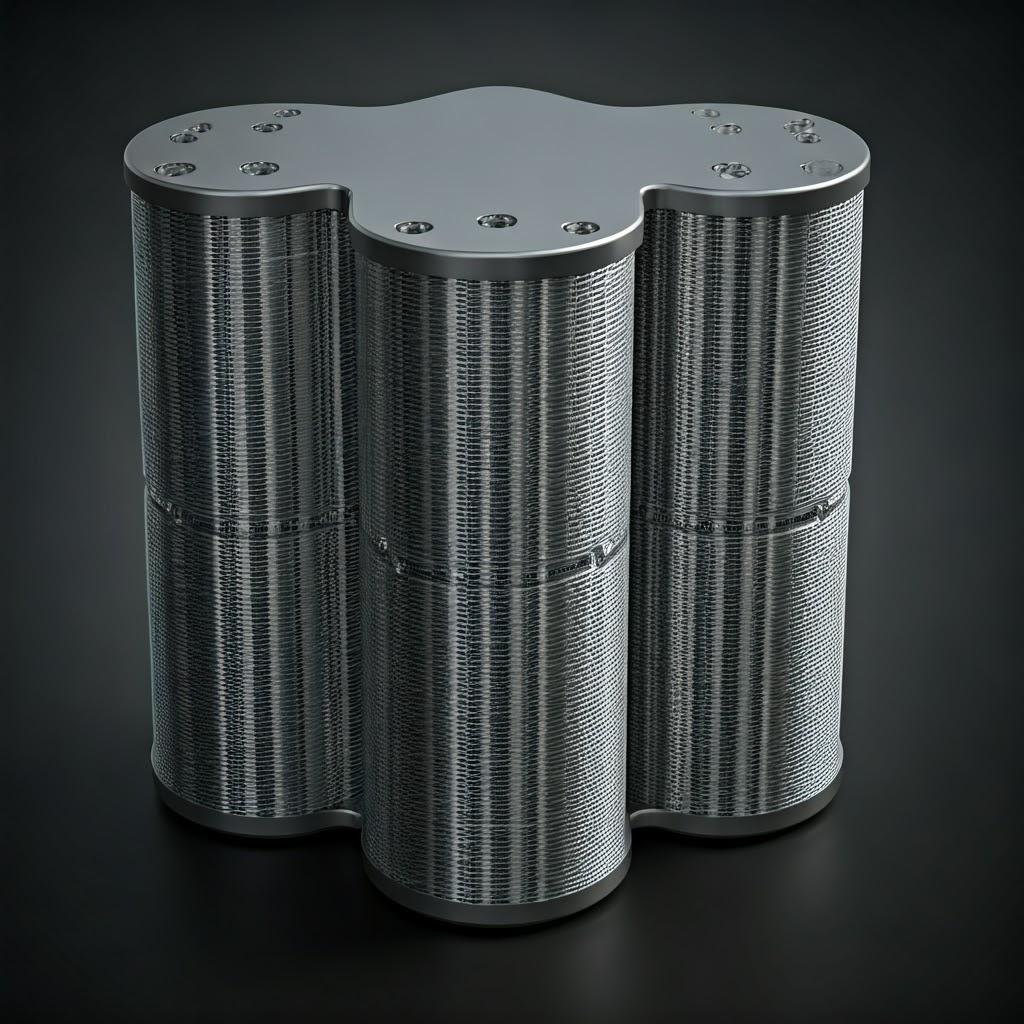
Supplier Selection: Choosing the Right Metal AM Partner for Aerospace Components
Selecting the right additive manufacturing service provider is as critical as the design and material choice, particularly for demanding aerospace applications like heat pipe enclosures. The difference between a successful, flight-qualified part and a costly failure often lies in the capabilities, experience, and quality systems of your manufacturing partner. Procurement managers and engineers sourcing these critical components need to evaluate potential suppliers rigorously based on a comprehensive set of criteria. Choosing an unsuitable partner can lead to delays, budget overruns, parts that fail qualification, and ultimately, mission risk.
Key Criteria for Evaluating Metal AM Suppliers for Space Applications:
- Aerospace Certifications and Compliance:
- AS9100: This is the standard quality management system (QMS) requirement for the aviation, space, and defense industries. Certification to AS9100 demonstrates a provider’s commitment to quality, traceability, risk management, and continuous improvement, tailored to the sector’s stringent demands. It’s often a non-negotiable requirement for prime contractors and OEMs.
- Nadcap: While more common for specific processes like heat treatment or NDT, Nadcap accreditation in relevant areas signifies adherence to industry-managed special process standards.
- Export Control Compliance: Familiarity and compliance with regulations like ITAR (International Traffic in Arms Regulations) or EAR (Export Administration Regulations) if applicable to the project’s nature and location.
- Proven Space Heritage and Experience:
- Erfolgsbilanz: Has the supplier successfully produced components currently flying on satellites, probes, or other spacecraft? Demonstrable experience with space-grade parts provides confidence in their understanding of the unique requirements (e.g., outgassing, radiation resistance, thermal vacuum testing).
- Fallstudien: Look for examples of similar projects, materials, and complexities they have handled.
- Understanding of Space Requirements: Do they understand cleanliness levels, material traceability, documentation needs, and qualification processes specific to space hardware?
- Material Expertise (AlSi10Mg, CuCrZr, and Beyond):
- Spezifische Legierungserfahrung: Deep knowledge of processing the specific alloys required (AlSi10Mg, CuCrZr). This includes optimized build parameters, appropriate heat treatment cycles, and understanding potential challenges like copper’s reflectivity or aluminum’s cracking susceptibility.
- Powder Handling & Quality: Robust procedures for handling, storing, sieving, and recycling aerospace-grade metal powders to maintain purity and prevent contamination. Ideally, they source from reputable suppliers or, like Met3dp, possess in-house capabilities for producing high-quality spherical powders using advanced methods like gas atomization or PREP, ensuring powder consistency and traceability.
- Broader Portfolio: While specific alloy experience is key, a broader portfolio (e.g., Titanium alloys, Nickel superalloys, specialty metals like TiNi, TiTa as offered by Met3dp) often indicates deeper materials science expertise.
- Technology, Equipment, and Capacity:
- Process Capability: Mastery of the relevant AM process (typically LPBF for fine features and good surface finish, sometimes SEBM for specific materials like titanium or crack-prone alloys).
- Machine Quality & Maintenance: Utilizing industrial-grade, well-maintained, and calibrated AM systems from reputable manufacturers. Consistent machine performance is vital for part quality.
- Capacity & Redundancy: Sufficient machine capacity to meet lead time requirements, potentially with multiple machines for redundancy and scalability.
- Environment Control: Build environments with controlled inert gas atmospheres (Argon/Nitrogen) and temperature management.
- Robustes Qualitätsmanagementsystem (QMS):
- Rückverfolgbarkeit: End-to-end traceability of materials (powder batches), process parameters, operator actions, and inspection results for each part.
- Inspektionskapazitäten: In-house or certified third-party access to necessary inspection equipment: CMM for dimensional checks, surface profilometry, NDT methods (CT scanning is highly valuable for AM internal feature validation), material analysis tools.
- Dokumentation: Ability to provide comprehensive documentation packages required for aerospace qualification (e.g., Certificates of Conformance, material certs, process logs, inspection reports).
- DfAM and Engineering Support:
- Kollaboration: Willingness and capability to work collaboratively with the customer’s design team, providing DfAM feedback to optimize the part for manufacturability, cost, and performance.
- Fachwissen: Access to experienced AM engineers and metallurgists who can advise on design rules, support strategies, material selection, and post-processing options.
- In-House vs. Managed Post-Processing:
- Integrated Services: Does the supplier offer critical post-processing steps (stress relief, heat treatment, support removal, basic finishing) in-house? This can streamline the workflow and improve control.
- Managed Network: If outsourcing post-processing (e.g., complex machining, specialized coatings, HIP, advanced NDT), do they have a network of qualified and trusted partners, and do they manage this process seamlessly?
- Lead Times and Responsiveness:
- Zitiergeschwindigkeit: Ability to provide timely and accurate quotes.
- Production Lead Times: Realistic and reliable lead time estimates for both prototypes and production parts.
- Kommunikation: Clear and proactive communication throughout the design, manufacturing, and delivery process.
- Wettbewerbsfähigkeit bei den Kosten:
- While cost is always a factor, it should be evaluated in the context of the other criteria. The cheapest option may not provide the necessary quality, reliability, or expertise for critical space components. Look for value – a balance of cost, quality, and service.
Checkliste zur Lieferantenbewertung (Beispiel):
Kriterien | Frage | Importance (High/Med/Low) | Notes / Target |
---|---|---|---|
Zertifizierungen | AS9100 Certified? | Hoch | Mandatory for many programs |
Erleben Sie | Proven space flight hardware experience? Case studies available? | Hoch | Look for relevant examples (thermal, materials) |
Material Expertise (AlSi10Mg) | Demonstrated success printing AlSi10Mg to aerospace specs? Optimized parameters? T6 treatment capability? | Hoch | Verify process control, property data |
Material Expertise (CuCrZr) | Experience with challenging copper alloy printing? Parameter control? Heat treatment capability? | Hoch | Requires specialized expertise |
Qualität des Pulvers | Strict powder handling/sourcing/QC? In-house powder production (e.g., Met3dp)? | Hoch | Fundamental to part quality |
Technologie | Appropriate AM machines (LPBF/SEBM)? Well-maintained? | Hoch | Machine quality impacts consistency |
QMS & Traceability | Robust QMS? Full traceability? Comprehensive documentation? | Hoch | Essential for qualification |
Inspection/NDT | In-house CMM, Surface analysis? Access to CT scanning? | Hoch | Verify capabilities match requirements |
DfAM-Unterstützung | Offers design review/feedback? Experienced AM engineers? | Med-High | Value-add, improves manufacturability |
Nachbearbeitung | Key steps in-house (Heat Treat, Machining)? Qualified network? | Med-High | Streamlines process, ensures quality |
Vorlaufzeit | Reliable quoting & production timelines? | Med | Balance with quality; understand bottlenecks |
Empfänglichkeit | Good communication? Proactive problem-solving? | Med | Important for smooth project execution |
Kosten | Competitive pricing relative to value/quality? | Med | Evaluate total cost of ownership, including risk |
In Blätter exportieren
Choosing a partner like Met3dp, which emphasizes its branchenführendes Druckvolumen, Genauigkeit und Zuverlässigkeit alongside advanced powder manufacturing and comprehensive solutions, aligns well with these critical selection criteria for demanding aerospace projects. Thorough due diligence in supplier selection is a vital investment in ensuring the success and reliability of 3D printed heat pipe enclosures for space systems.
Understanding Investment: Cost Factors and Lead Times for AM Heat Pipe Enclosures
While metal additive manufacturing offers significant performance and design benefits, it’s essential for procurement managers and engineers to understand the factors influencing the cost and lead time associated with producing 3D printed heat pipe enclosures. A clear understanding allows for better budgeting, project planning, and comparison with traditional manufacturing alternatives where feasible. Costs are typically higher per part than mass-produced simple items but can be highly competitive for complex, low-volume, high-performance components like those used in space.
Key Cost Drivers for Metal AM Heat Pipe Enclosures:
- Materialart und Verbrauch:
- Wahl der Legierung: Copper alloy powders (CuCrZr) are generally significantly more expensive than aluminum alloy powders (AlSi10Mg).
- Qualität des Pulvers: High-quality, aerospace-grade powders with specific particle size distributions and purity levels command higher prices.
- Part Volume & Support Volume: The total amount of material melted (part volume) and the amount used for support structures directly impact cost. DfAM efforts to minimize volume and supports help reduce material consumption.
- Powder Reuse: Efficient powder recycling practices by the supplier can help mitigate costs, but require careful quality control.
- Maschinenzeit (Bauzeit):
- Teilhöhe (Z-Höhe): Build time is strongly correlated with the number of layers, meaning taller parts take longer, irrespective of their footprint. Building multiple parts simultaneously can optimize machine time if they fit within the build envelope.
- Teilband: Larger volume parts require more material to be melted per layer, increasing scan time.
- Komplexität: Highly complex geometries, thin walls, and intricate features often require slower scan speeds or specific strategies, increasing build time.
- AM-Prozess: Print speeds can vary between different AM technologies (e.g., LPBF vs. SEBM) and specific machine models.
- Maschinentarif: The hourly operational cost of the industrial metal AM system, factoring in depreciation, energy, maintenance, and consumables (gas, filters).
- Arbeitskosten:
- Vorbereitung der Datei: Preparing the CAD file for printing, generating support structures, and creating the build layout requires skilled labor.
- Machine Setup & Monitoring: Loading powder, setting up the build, monitoring the process, and unloading the finished part.
- Nachbearbeitungsarbeiten: Significant labor is involved in de-powdering, support removal, heat treatment, machining, finishing, cleaning, and inspection. The complexity of the part heavily influences this cost component.
- Engineering/DfAM Support: If collaborative design optimization is involved, associated engineering labor costs apply.
- Post-Processing Complexity:
- Wärmebehandlung: Costs associated with furnace time, energy, and controlled atmospheres. Complex or multi-stage cycles add cost.
- Unterstützung bei der Entfernung: Difficult-to-access supports require more manual labor or advanced techniques (EDM, machining), increasing cost.
- Bearbeitungen: The extent and precision of required CNC machining significantly impact cost (number of setups, machining time, tooling).
- Oberflächenveredelung: Polishing, blasting, or specialized coating requirements add cost based on the process and area treated.
- HIP: Hot Isostatic Pressing adds a substantial cost but may be required for critical applications demanding maximum density and fatigue life.
- ZfP und Inspektion: Costs associated with CT scanning, CMM measurements, surface analysis, and other required quality checks.
- Qualification and Documentation:
- Prüfung: If specific part qualification testing (e.g., thermal vacuum, vibration, proof testing) is required, these costs are added.
- Documentation Package: Compiling comprehensive traceability and quality documentation for aerospace standards requires significant effort and adds to the cost.
- Auftragsvolumen:
- Skalenvorteile: While AM is suited for low volumes, some economies of scale exist. Setup costs are amortized over more parts in larger batches. Printing multiple parts in a single build optimizes machine utilization. However, the cost reduction per part typically flattens out much sooner than in traditional mass production methods.
Typische Vorlaufzeiten:
Lead times for 3D printed metal heat pipe enclosures can vary widely based on several factors:
- Prototyping: Simple prototypes with minimal post-processing might be produced in 1-3 Wochen.
- Functional Prototypes: Parts requiring heat treatment and basic machining could take 3-6 Wochen.
- Production Parts (Non-Qualified): Complex parts with extensive post-processing (machining, finishing) but without formal qualification might range from 6-10 weeks.
- Flight-Qualified Parts: Parts requiring full aerospace qualification, including extensive post-processing, rigorous NDT, potential HIP, and comprehensive documentation, can have lead times of 10-20 weeks or more, depending on the complexity of the qualification process and testing requirements.
Faktoren, die die Vorlaufzeit beeinflussen:
- Supplier Queue: Current workload and machine availability at the chosen service provider.
- Komplexität des Designs: More complex parts take longer to print and post-process.
- Bauhöhe: Taller parts take longer to print.
- Nachbearbeitungsanforderungen: Each step (heat treatment, machining, coating, HIP, NDT) adds time. Outsourced steps can introduce further delays.
- Qualification Process: Iterations, testing schedules, and documentation reviews add significant time.
- Materialverfügbarkeit: Ensuring the specific aerospace-grade powder is in stock or has an acceptable procurement lead time.
It’s crucial to discuss cost drivers and lead time expectations transparently with potential suppliers early in the project. Providing a clear definition of requirements, including CAD models, material specifications, tolerance/finish requirements, post-processing needs, and required documentation, will enable suppliers to provide more accurate quotes and realistic timelines.
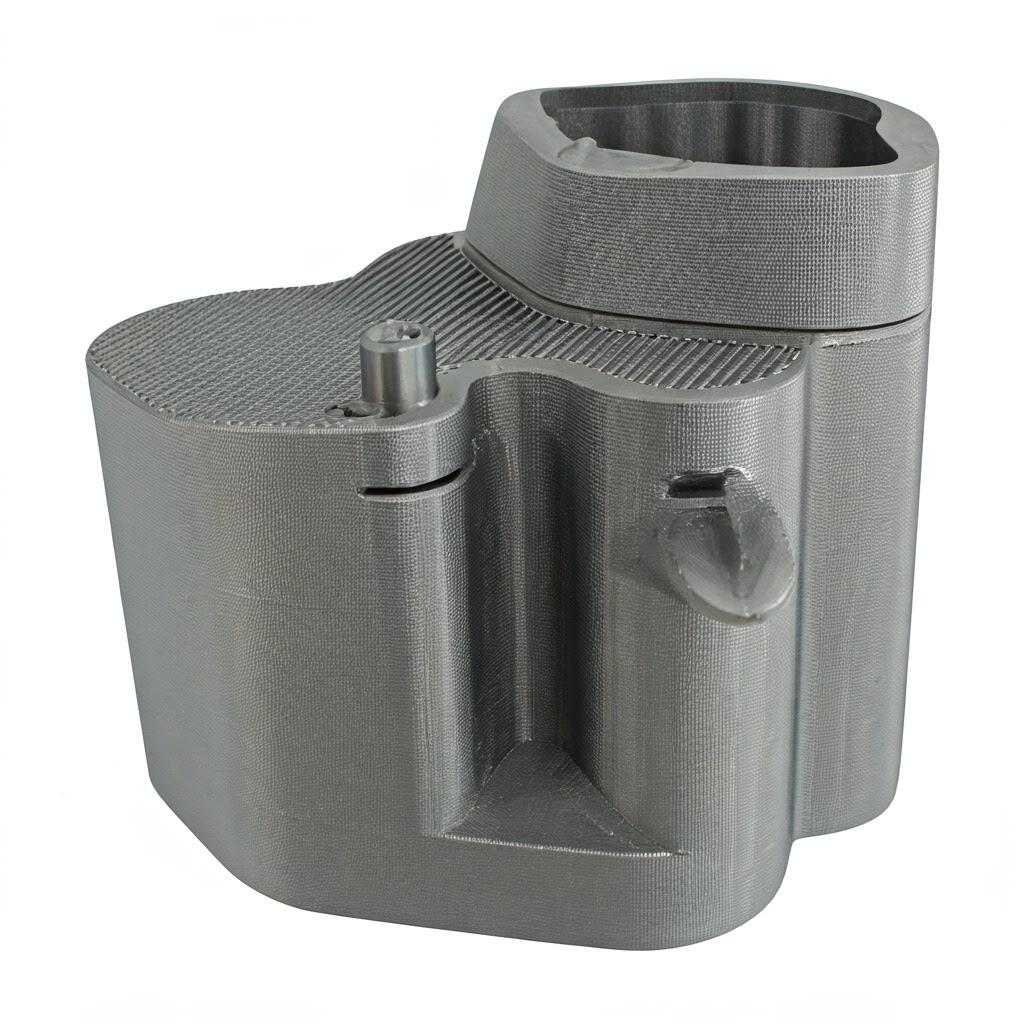
Frequently Asked Questions (FAQ) about 3D Printed Heat Pipe Enclosures
Here are answers to some common questions regarding the use of metal additive manufacturing for heat pipe enclosures in space systems:
- How does the thermal performance of a 3D printed heat pipe enclosure compare to a traditionally manufactured one?
- The performance can be equal or significantly better, depending on the design. Metal AM allows for highly optimized designs that are impossible to make traditionally. Features like conformal channels, integrated fins, optimized internal wick structures (for LHPs/CPLs), and consolidated assemblies (reducing thermal resistance at joints) can enhance heat transfer efficiency. While the bulk thermal conductivity of an AM material might be slightly lower than its wrought equivalent due to microstructure, the design freedom often more than compensates, leading to superior overall thermal performance in the final assembly. Careful material selection (e.g., high-conductivity CuCrZr where needed) and DfAM are key.
- What is the Technology Readiness Level (TRL) for metal AM components like heat pipe enclosures in space?
- Metal AM for space applications has matured significantly. For many applications, particularly using well-characterized alloys like AlSi10Mg and Ti6Al4V, the TRL is considered high (TRL 7-9), meaning the technology has been demonstrated and proven in operational environments (i.e., flown in space). Numerous examples exist of non-critical and increasingly critical structural and thermal components produced via AM on satellites and spacecraft. For newer alloys (like potentially AM CuCrZr in some specific complex geometries) or highly critical primary structures, the TRL might be slightly lower (TRL 5-7), requiring further qualification and demonstration for specific mission contexts. Verification always depends on the specific part, material, process, and mission requirements.
- Are 3D printed AlSi10Mg and CuCrZr enclosures suitable for long-duration space missions considering radiation, vacuum, and thermal cycling?
- Yes, generally.
- Vacuum: Both AlSi10Mg and CuCrZr are metallic alloys with very low outgassing properties (when properly cleaned), making them suitable for vacuum environments. Material selection considers vapor pressure and outgassing rates to prevent contamination of sensitive surfaces.
- Radiation: Metallic structures offer inherent radiation shielding. While extreme radiation can cause some material property degradation over very long missions, aluminum and copper alloys are commonly used in space structures and are generally considered suitable. Specific effects depend on the orbit, mission duration, and shielding design.
- Thermal Cycling: Both alloys can withstand the thermal cycling experienced in space, although careful design and stress relief are crucial to prevent fatigue failure due to expansion/contraction stresses over many cycles. Material properties at operational temperature extremes must be considered during design. Rigorous testing (thermal cycling, thermal vacuum) is part of the standard space qualification process.
- Yes, generally.
- What information does an AM supplier need to provide an accurate quote for a 3D printed heat pipe enclosure?
- To get the most accurate quote, provide as much detail as possible:
- 3D-CAD-Modell: A STEP file is standard.
- Spezifikation des Materials: Clearly state the required alloy (e.g., AlSi10Mg or CuCrZr) and any specific material standards (e.g., AMS specifications).
- Technical Drawing: Include critical dimensions, tolerances, surface finish requirements (Ra values) for specific features, and locations requiring post-machining.
- Nachbearbeitungsanforderungen: Specify required heat treatments (stress relief, aging), surface treatments (coatings, polishing), HIP (if needed).
- Menge: Number of parts required.
- Quality & Documentation Requirements: Specify required certifications (e.g., AS9100), inspection methods (NDT needs like CT scanning), and documentation package (CofC, material certs, inspection reports).
- Anwendungskontext (fakultativ, aber hilfreich): Briefly describing the application helps the supplier understand the criticality and functional requirements.
- To get the most accurate quote, provide as much detail as possible:
Conclusion: The Future of Thermal Control – Advanced Manufacturing with Met3dp
The effective management of thermal loads remains one of the most critical challenges in designing and operating reliable, high-performance spacecraft. Heat pipes are indispensable tools in this endeavor, and the enclosures that house and integrate them are evolving rapidly thanks to the transformative capabilities of metal additive manufacturing. As we’ve explored, utilizing AM technologies like Laser Powder Bed Fusion (LPBF) with advanced materials such as lightweight AlSi10Mg or high-conductivity CuCrZr allows engineers to break free from the constraints of traditional manufacturing.
The advantages are compelling: unparalleled design freedom enabling complex, conformal geometries and integrated features; significant lightweighting through topology optimization and lattice structures, directly impacting launch costs; part consolidation leading to reduced complexity, assembly time, and potential failure points; and accelerated development cycles via rapid prototyping and iteration. These benefits translate directly into more efficient, compact, and reliable thermal control systems, paving the way for more ambitious scientific missions, denser satellite payloads, and sustainable long-duration space exploration.
Successfully leveraging these advantages, however, requires a holistic approach. It demands embracing Design for Additive Manufacturing (DfAM) principles from the outset, carefully selecting the optimal material for the specific thermal and structural requirements, understanding and planning for necessary post-processing steps (from heat treatment to precision machining and rigorous inspection), and navigating the inherent challenges of the AM process through robust process control and quality assurance.
Crucially, success hinges on collaboration with the right manufacturing partner. Choosing a supplier with proven aerospace experience, deep material and process expertise, stringent quality certifications (like AS9100), comprehensive capabilities spanning DfAM support to final inspection, and a commitment to quality – such as Met3dp – is paramount. Met3dp, headquartered in Qingdao, China, stands as a leading provider of additive manufacturing solutions, specializing in both advanced 3D printing equipment and the high-performance metal powders essential for industrial applications in aerospace, medical, and automotive sectors. Their utilization of industry-leading gas atomization and PREP technologies ensures the production of high-sphericity, high-flowability metal powders, including innovative alloys, enabling customers to print dense, high-quality parts with superior properties. With decades of collective expertise, Met3dp offers comprehensive solutions, partnering with organizations to accelerate their digital manufacturing transformations.
Metal additive manufacturing is no longer a futuristic novelty for space; it is a present-day enabler of next-generation capabilities. For thermal management systems, 3D printed heat pipe enclosures represent a significant leap forward, offering performance and integration possibilities previously unattainable. As the space industry continues to push boundaries, advanced manufacturing techniques and expert partners like Met3dp will be instrumental in realizing the innovative thermal solutions required for mission success.
Teilen auf
Facebook
Twitter
LinkedIn
WhatsApp
E-Mail
MET3DP Technology Co., LTD ist ein führender Anbieter von additiven Fertigungslösungen mit Hauptsitz in Qingdao, China. Unser Unternehmen ist spezialisiert auf 3D-Druckgeräte und Hochleistungsmetallpulver für industrielle Anwendungen.
Fragen Sie an, um den besten Preis und eine maßgeschneiderte Lösung für Ihr Unternehmen zu erhalten!
Verwandte Artikel
Über Met3DP
Aktuelles Update
Unser Produkt
KONTAKT US
Haben Sie Fragen? Senden Sie uns jetzt eine Nachricht! Wir werden Ihre Anfrage mit einem ganzen Team nach Erhalt Ihrer Nachricht bearbeiten.
Holen Sie sich Metal3DP's
Produkt-Broschüre
Erhalten Sie die neuesten Produkte und Preislisten
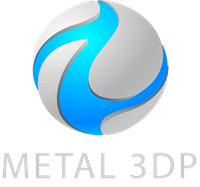
Metallpulver für 3D-Druck und additive Fertigung
UNTERNEHMEN
PRODUKT
cONTACT INFO
- Qingdao Stadt, Shandong, China
- [email protected]
- [email protected]
- +86 19116340731