Heat Dissipation Panels for Satellites via Metal AM
Inhaltsübersicht
Introduction: The Critical Role of Thermal Management in Satellite Systems
The final frontier, space, presents an environment of extreme contrasts. Satellites orbiting our planet, or venturing further into the cosmos, are subjected to intense solar radiation on one side while facing the frigid vacuum of space on the other. Internally, the sophisticated electronics, powerful communication systems, sensitive optical payloads, and propulsion units generate significant amounts of waste heat. Managing these thermal loads is not merely an operational convenience; it is absolutely paramount for mission success, component longevity, and the overall reliability of these multi-million or even billion-dollar assets. Inadequate thermal management can lead to component overheating, performance degradation, signal distortion, reduced lifespan, and, in catastrophic cases, complete mission failure. The field of satellite thermal control engineering is dedicated to maintaining all satellite components within their specific, often narrow, allowable temperature ranges throughout all mission phases – from launch and deployment to orbital operations and potential end-of-life maneuvers. This involves a complex interplay of passive methods (like insulation blankets and surface coatings) and active methods (like heaters and mechanically pumped fluid loops). Central to many passive thermal control strategies are heat dissipation panels, often referred to as radiators, which are designed to efficiently collect waste heat from internal sources and radiate it away into the cold expanse of space.
Traditionally, these crucial components have been manufactured using methods like CNC machining of solid metal blocks (often aluminum or copper alloys), assembling complex structures through brazing or bonding multiple pieces, or embedding heat pipes into panels. While effective, these conventional approaches face inherent limitations, particularly as satellite designs become more compact, power-hungry, and demand higher performance within stringent mass budgets. Machining complex internal channel geometries for enhanced fluid flow or intricate external fin structures for maximum radiative surface area can be difficult, time-consuming, and generate significant material waste. Assembling multiple parts introduces potential points of failure at joints and adds complexity to the manufacturing and quality assurance processes. Furthermore, the design freedom offered by these methods is often constrained, limiting the potential for truly optimized thermal performance and weight reduction – a critical factor given that every kilogram launched into orbit incurs substantial cost.
Eingeben Additive Fertigung von Metall (AM), also known as metal 3D-Druck. This disruptive technology is rapidly transforming the landscape of aerospace engineering, offering unprecedented capabilities for creating highly complex, lightweight, and performance-optimized components. Unlike subtractive methods that remove material, AM builds parts layer by layer directly from a digital model, typically using a high-energy laser or electron beam to fuse fine metal powders. This layer-wise approach unlocks a new dimension of design freedom, enabling the creation of geometries previously impossible or prohibitively expensive to manufacture. For satellite heat dissipation panels, this translates into the ability to design and produce components with:
- Optimized Topologies: Internal structures can be algorithmically designed to follow thermal load paths precisely, placing material only where needed for heat transfer and structural support, drastically reducing mass.
- Complex Internal Channels: Conformal cooling channels that closely follow the contours of heat-generating components or intricate networks mimicking biological vascular systems can be directly printed, maximizing heat absorption efficiency.
- Integrierte Funktionen: Mounting points, brackets, fluid connectors, and even embedded sensor housings can be incorporated into a single monolithic part, reducing assembly complexity and potential leak paths.
- Enhanced Surface Area: Lattice structures, fins, and complex surface textures can be integrated to significantly increase the effective radiating surface area without a proportional increase in mass.
- Werkstoff-Innovation: AM allows for the processing of advanced alloys specifically chosen for their thermal properties and space suitability, and even opens the door to functionally graded materials where properties vary within a single component.
Unternehmen wie Met3dp, a leading provider of additive manufacturing solutions specializing in high-performance metal powders and industrial 3D printing equipment, are at the forefront of this technological shift. By leveraging advanced powder manufacturing techniques like gas atomization and Plasma Rotating Electrode Process (PREP), Met3dp produces high-quality spherical metal powders, including alloys ideal for aerospace thermal applications, ensuring the consistency and purity required for mission-critical components. Their expertise in processes like Selective Electron Beam Melting (SEBM) further enables the production of dense, reliable metal parts with superior mechanical properties. As the aerospace industry continues to push the boundaries of performance and efficiency, metal AM stands poised to revolutionize the design and production of satellite thermal management systems, making space missions more capable, reliable, and cost-effective. Procurement managers and aerospace engineers looking for B2B solutions and reliable suppliers for advanced satellite hardware should consider the transformative potential offered by metal AM providers.
What Are Satellite Heat Dissipation Panels Used For?
Satellite heat dissipation panels, in their various forms (radiators, heat sinks, thermal straps), serve a singular, critical purpose: maintaining the thermal equilibrium of the spacecraft. They act as the primary interface for rejecting waste heat, generated by onboard systems, into the surrounding space environment. Without efficient heat rejection, the internal temperatures of a satellite could quickly rise beyond the operational limits of sensitive electronics, scientific instruments, batteries, and other critical hardware.
Core Function: The fundamental principle is thermal radiation. Heat generated by internal components is conducted or convected (via heat pipes or fluid loops) to the radiator panel. The outer surface of this panel, often treated with specific coatings to maximize thermal emissivity and minimize solar absorptivity, then radiates this thermal energy away as infrared radiation into the near-perfect vacuum of space, which acts as an effective heat sink. The efficiency of this process depends heavily on the panel’s surface area, its surface properties (emissivity), its temperature, and its view factor to cold space (avoiding views of the Sun, Earth, or other spacecraft parts).
Key Industries & Stakeholders:
- Spacecraft Manufacturers: Prime contractors (e.g., Boeing, Airbus Defence and Space, Lockheed Martin, Thales Alenia Space, Maxar Technologies) and specialized satellite builders who design, assemble, integrate, and test entire satellite systems rely heavily on efficient thermal control subsystems. They are major consumers and Großhandelskäufer of satellite components, including heat dissipation panels.
- Satelliten-Betreiber: Companies and government agencies (e.g., Intelsat, SES, Eutelsat, NASA, ESA, national space agencies) that own and operate satellite constellations for communication, Earth observation, navigation, or scientific purposes depend on the long-term reliability ensured by robust thermal management.
- Aerospace & Defense Contractors: Companies supplying subsystems and components to prime manufacturers often specialize in areas like thermal control hardware. These B2B-Lieferanten require access to advanced manufacturing technologies like metal AM to meet demanding specifications.
- Component Suppliers & Distributors: Businesses focused on providing specific aerospace-grade components, including thermal management hardware, often act as intermediaries or specialized manufacturers, seeking reliable sources for Masse orders or custom parts.
Specific Applications within Satellites:
- Primary Radiator Panels: These are often large, externally mounted panels, sometimes deployable, designed to reject the bulk of the spacecraft’s waste heat. Their size and placement are carefully calculated based on the total heat load and orbital environment. Metal AM allows for lightweight structural designs with integrated heat pipes or complex fin geometries to maximize the area-to-mass ratio.
- Component-Specific Heat Sinks: High-power electronic components like Solid State Power Amplifiers (SSPAs), Traveling Wave Tube Amplifiers (TWTAs), processors, and FPGAs generate concentrated heat loads. Smaller, dedicated heat sinks, often intricately shaped to conform to the component’s footprint and maximize contact area, are used to draw heat away effectively. AM enables highly complex shapes, potentially with internal micro-channel structures for liquid cooling integration or optimized fin designs far beyond machining capabilities.
- Integrated Structural/Thermal Panels: In modern satellite design, there’s a push towards multifunctional structures. Panels that serve both as load-bearing structural elements and heat radiators are increasingly common. Metal AM is ideal for creating such components, optimizing both mechanical stiffness and thermal dissipation pathways within a single, lightweight part. Topology optimization algorithms can generate complex, organic-looking structures that efficiently manage both mechanical loads and heat flow.
- Thermal Straps and Doublers: Flexible thermal straps are sometimes used to conduct heat across joints or moving parts, while thermal doublers (thin plates of high-conductivity material) help spread concentrated heat loads over a larger area of a radiator panel. While straps are often different materials, AM can be used to create highly optimized thermal doublers or rigid thermal links with complex shapes and minimal mass, potentially integrating them directly into the panel structure.
- Heat Pipe Assemblies: Heat pipes are highly efficient passive heat transfer devices. AM can be used to create complex evaporator and condenser sections for heat pipes or even to embed the heat pipe structure directly within the walls of a structural panel or electronic enclosure, leading to highly integrated and efficient thermal solutions. This requires specialized AM techniques and materials, often involving copper alloys like CuCrZr for the wick and casing due to their high thermal conductivity.
The demand for increasingly sophisticated satellite capabilities, coupled with the drive for smaller, lighter, and more cost-effective platforms (like CubeSats and smallsats), places immense pressure on thermal management systems. Procurement managers and engineers in the satellite industry are constantly seeking innovative solutions and reliable Zulieferer der Luft- und Raumfahrtindustrie capable of delivering next-generation performance. Metal additive manufacturing, offered by experienced providers, presents a compelling pathway to meet these evolving requirements for satellite heat dissipation panels and related thermal hardware, offering unparalleled design freedom and performance potential.
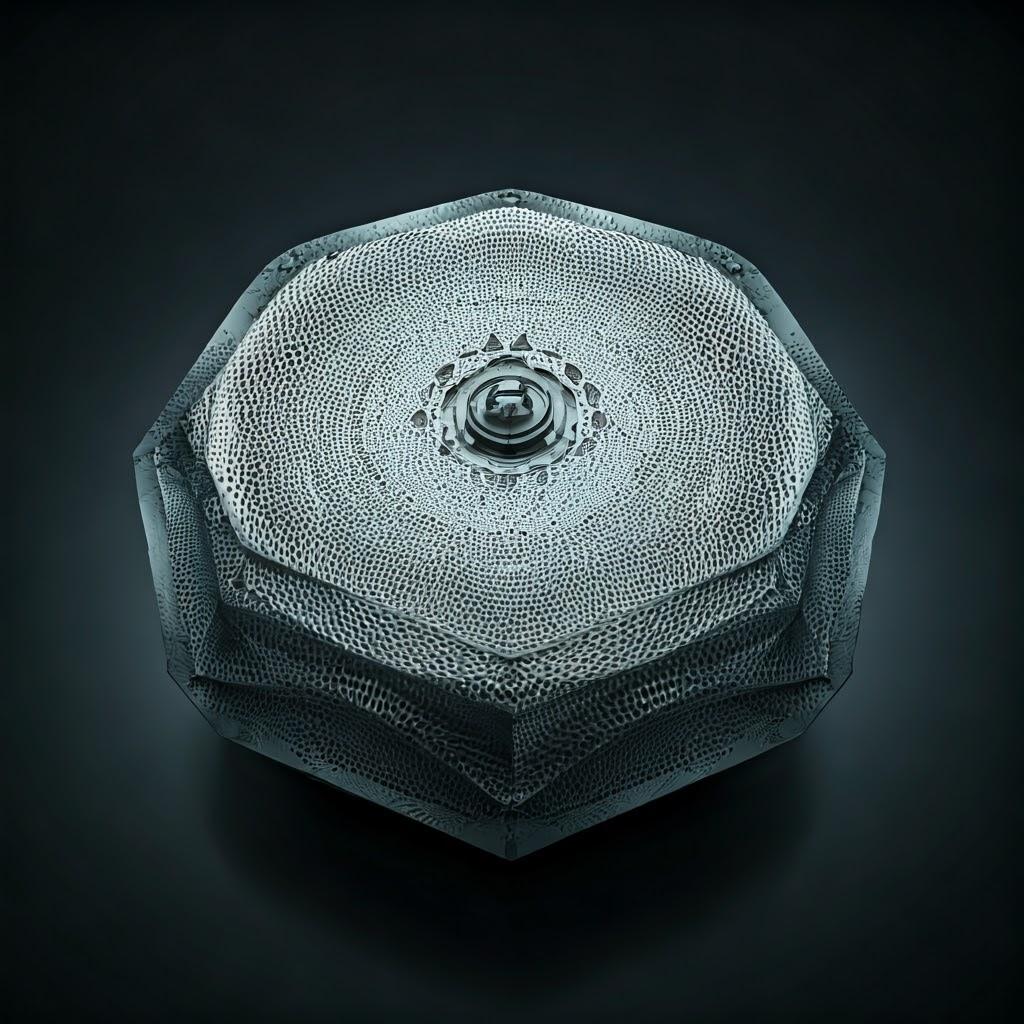
Why Use Metal 3D Printing for Satellite Heat Dissipation Panels?
The decision to adopt metal additive manufacturing for critical components like satellite heat dissipation panels stems from a confluence of compelling advantages that directly address the limitations of traditional methods and align perfectly with the demanding requirements of the aerospace industry. While conventional manufacturing techniques like CNC machining, sheet metal forming, and brazing/welding assemblies have served the industry well, metal AM offers a paradigm shift, enabling enhanced performance, reduced lead times, and novel design possibilities. For B2B-Beschaffung specialists and design engineers focused on optimizing satellite subsystems, understanding these benefits is crucial.
Key Advantages of Metal AM for Heat Dissipation Panels:
- Unparalleled Geometric Complexity: This is arguably the most significant advantage. AM builds parts layer-by-layer, freeing designers from the constraints imposed by subtractive machining or assembly processes.
- Optimized Internal Channels: Design intricate, smoothly curving internal channels that conform precisely to heat sources, minimizing thermal resistance. Create complex manifold designs for fluid-cooled panels that would be impossible to machine.
- Gitterförmige Strukturen: Incorporate periodic or stochastic lattice structures within the panel. These cellular materials offer extremely high surface area-to-volume ratios, ideal for enhancing convective heat transfer (if internally cooled) or radiative surface area, while simultaneously providing structural support at very low densities. This leads to significantly lighter panels compared to solid structures.
- Biomimicry: Mimic natural cooling structures, like vascular networks or lung alveoli, to create highly efficient heat exchange pathways.
- Integrierte Funktionen: Print mounting bosses, alignment features, connector ports, and complex fin geometries as integral parts of the panel, eliminating assembly steps, reducing part count, and improving reliability.
- Significant Weight Reduction (Mass Optimization): Launch costs are a primary driver in satellite design, often costing tens of thousands of dollars per kilogram sent to orbit. AM excels at creating lightweight structures.
- Topologie-Optimierung: Use computational tools to distribute material only where it’s needed for thermal performance and structural integrity, removing unnecessary mass. This results in highly efficient, often organic-looking designs that are significantly lighter than conventionally manufactured counterparts.
- Thin Walls & Complex Cores: Print panels with thin, structurally sound walls and complex internal corrugations or lattice cores, achieving high stiffness and thermal performance with minimal material usage.
- Teil Konsolidierung: Redesign assemblies previously made of multiple joined parts into a single, monolithic AM component. This inherently reduces weight by eliminating fasteners, flanges, and redundant material at interfaces.
- Part Consolidation and Reduced Assembly: As mentioned above, the ability to integrate multiple functions and features into a single printed part dramatically simplifies the supply chain and manufacturing process.
- Fewer Components: Reduces inventory management, procurement complexity, and potential points of failure (joints, welds, fasteners).
- Simplified Assembly: Less time and labor required for final satellite integration.
- Verbesserte Verlässlichkeit: Monolithic parts generally have higher structural integrity and fewer potential leak paths compared to complex assemblies.
- Rapid Prototyping and Accelerated Design Iteration: AM allows engineers to move quickly from digital design to physical prototype.
- Faster Testing Cycles: Produce functional prototypes of heat dissipation panels in days or weeks rather than months, allowing for rapid testing, validation, and refinement of designs.
- Flexibilität bei der Gestaltung: Easily modify designs based on test results and print new iterations quickly without the need for expensive re-tooling associated with traditional methods. This iterative process leads to more highly optimized final parts.
- Anpassungen: Efficiently produce unique or low-volume custom designs tailored to specific mission requirements or component interfaces without incurring high setup costs.
- Material Optimization and Advanced Alloys: AM processes can work with a growing range of advanced metal alloys, including those specifically optimized for thermal management and the space environment.
- Optimized Material Selection: Choose materials like AlSi10Mg for its balance of low density and good thermal conductivity, or CuCrZr for applications demanding maximum heat transfer, leveraging the specific strengths of each alloy.
- Functionally Graded Materials (Research Area): Future potential exists to vary material composition across a single part using AM, potentially creating panels with tailored thermal conductivity gradients or integrated zones of high strength and high conductivity.
- Geringerer Materialabfall: AM is an additive process, typically using only the material needed for the part and necessary supports, resulting in significantly less waste compared to subtractive machining, which can machine away up to 80-90% of the initial stock material. This is particularly important for expensive aerospace-grade alloys. Sourcing metal powder wholesale becomes more efficient.
- Reduced Lead Times for Complex & Custom Parts: While setting up an AM build requires preparation, the actual printing and post-processing time for highly complex or custom, low-volume parts can be significantly shorter than the combined time required for tooling, machining setup, multi-axis milling, and assembly using traditional methods. This is a critical advantage for aerospace suppliers needing to meet tight program schedules.
Die Auswahl einer 3D-Druck-Dienstleister für Metall like Met3dp, with deep expertise in aerospace applications, advanced materials like AlSi10Mg and CuCrZr, and robust process control, allows satellite manufacturers and their B2B partners to fully leverage these advantages. Met3dp’s focus on producing high-quality, spherical powders ensures optimal processing and final part quality, while their experience with industrial printers guarantees the accuracy and reliability needed for mission-critical space hardware. The transition towards AM for components like heat dissipation panels represents a strategic move towards building more capable, lighter, and cost-effective satellites.
Recommended Materials (AlSi10Mg & CuCrZr) and Why They Matter
Selecting the right material is fundamental to the success of any engineering component, and this holds especially true for satellite heat dissipation panels where performance, weight, and reliability are critical. Metal additive manufacturing opens up possibilities but also necessitates careful consideration of how materials behave during the printing process and how their final properties align with application requirements. For thermal management in space, two materials frequently stand out in the AM landscape: Aluminum alloy AlSi10Mg and Copper alloy CuCrZr. Understanding their respective properties, processing nuances, and ideal use cases is essential for engineers and Beschaffungsmanager sourcing AM components or metal powders wholesale.
Aluminum Alloy: AlSi10Mg
AlSi10Mg is one of the most common and well-characterized aluminum alloys used in metal AM, particularly via Laser Powder Bed Fusion (LPBF). It’s essentially a casting alloy adapted for additive processes, known for its excellent combination of properties that make it highly suitable for a wide range of aerospace applications, including thermal components.
- Wichtige Eigenschaften:
- Geringe Dichte: Approximately 2.67g/cm3. This is a major advantage for aerospace, contributing significantly to weight reduction compared to copper, steel, or titanium alloys.
- Gute Wärmeleitfähigkeit: Typically in the range of 120−180W/(m⋅K) after appropriate heat treatment. While not as high as pure aluminum or copper, it’s sufficient for many radiator and heat sink applications, especially when AM allows for designs with greatly increased surface area.
- Gutes Verhältnis von Stärke zu Gewicht: Offers decent mechanical strength and stiffness, particularly after heat treatment (e.g., T6), allowing panels to serve structural roles as well.
- Excellent Processability in AM: Melts and solidifies well under laser exposure, generally resulting in dense parts with good dimensional accuracy. It’s widely available from various Lieferanten von Metallpulver.
- Korrosionsbeständigkeit: Exhibits good resistance to corrosion.
- Suitability for Heat Dissipation Panels:
- Ideal for larger radiator panels where overall mass is a primary concern.
- Suitable for integrated structural/thermal components where both mechanical support and heat dissipation are required.
- Effective for heat sinks dealing with moderate heat loads where the design freedom of AM can compensate for the moderate thermal conductivity through increased surface area (fins, lattices).
- Cost-effective compared to copper alloys or specialized high-temperature materials.
- Überlegungen zur Verarbeitung:
- Typically processed using LPBF systems.
- Requires post-process heat treatment (e.g., stress relief followed by solution annealing and artificial aging – T6 cycle) to optimize mechanical properties and thermal conductivity, and to ensure dimensional stability.
- Can be susceptible to porosity if process parameters or powder quality are not carefully controlled.
- Support structures are generally required for overhanging features and need careful removal.
- Sourcing: High-quality AlSi10Mg powder, characterized by controlled particle size distribution, high sphericity, low satellite content, and low oxygen levels, is crucial for achieving consistent, high-density parts. Reputable B2B powder distributors and manufacturers like Met3dp, employing advanced atomization techniques (like gas atomization), ensure these critical powder characteristics are met, providing powders optimized for demanding LPBF applications. Met3dp’s range of metal powders includes high-grade AlSi10Mg suitable for aerospace needs.
Copper Alloy: CuCrZr (Copper-Chromium-Zirconium)
When maximum thermal and electrical conductivity is the primary requirement, copper alloys are the go-to materials. CuCrZr is a precipitation-strengthened alloy that offers an exceptional combination of high conductivity and relatively good mechanical strength, especially at moderately elevated temperatures, making it superior to pure copper in terms of processability and structural integrity in AM.
- Wichtige Eigenschaften:
- Ausgezeichnete Wärmeleitfähigkeit: Typically exceeding 300W/(m⋅K), more than twice that of AlSi10Mg. This is crucial for efficiently transferring high heat fluxes.
- Excellent Electrical Conductivity: Also possesses high electrical conductivity, making it suitable for applications involving combined thermal/electrical management (e.g., bus bars with integrated cooling).
- Good High-Temperature Strength: Retains strength better at elevated temperatures compared to pure copper or many aluminum alloys.
- Gute Korrosionsbeständigkeit: Similar to copper, offers good resistance in many environments.
- Höhere Dichte: Significantly denser than aluminum alloys (approx. 8.9g/cm3), making weight a more critical design consideration.
- Suitability for Heat Dissipation Panels:
- Ideal for compact, high-performance heat sinks dealing with very concentrated heat loads (e.g., cooling high-power electronics, laser diodes).
- Used in heat exchangers and heat pipe components where maximizing heat transfer efficiency is paramount.
- Applications requiring high electrical conductivity in addition to thermal dissipation.
- Situations where the higher density can be tolerated or offset by highly optimized AM designs.
- Überlegungen zur Verarbeitung:
- Challenging to process via AM due to copper’s high reflectivity and high thermal conductivity, which affects energy absorption from lasers or electron beams.
- Requires high-power lasers (often green lasers in LPBF) or electron beams (SEBM) with carefully optimized parameters to achieve dense, defect-free parts.
- Susceptible to oxidation; processing under a high-purity inert atmosphere or vacuum (as in SEBM) is essential.
- Requires post-process heat treatment (solution treatment and aging) to achieve the desired combination of strength and conductivity.
- Powder quality (sphericity, purity, flowability) is extremely critical for successful processing.
- Sourcing: Finding a reliable CuCrZr powder supplier experienced in producing powders specifically for AM is vital due to the processing challenges. The powder must have excellent flowability and high purity. Companies like Met3dp, leveraging their expertise in advanced powder production methods like PREP (Plasma Rotating Electrode Process) for reactive and high-quality spherical powders, are well-positioned to supply challenging materials like CuCrZr, ensuring the characteristics needed for successful, high-performance AM builds. Procurement managers should specifically inquire about experience and quality control for copper alloy powders when evaluating wholesale AM material providers.
Comparison Table: AlSi10Mg vs. CuCrZr for AM Thermal Applications
Merkmal | AlSi10Mg | CuCrZr | Key Consideration for Satellite Panels |
---|---|---|---|
Primärer Vorteil | Lightweight, Good Processability | Excellent Thermal/Electrical Conductivity | Application dictates priority (Weight vs. Performance) |
Dichte | Low (~2.67g/cm3) | High (~8.9g/cm3) | Critical for launch cost; favors AlSi10Mg |
Wärmeleitfähigkeit | Good (120−180W/(m⋅K)) | Excellent (>300W/(m⋅K)) | Critical for high heat flux; favors CuCrZr |
Mechanische Festigkeit | Good (especially after T6 treatment) | Moderate to Good (Precipitation hardened) | Important for structural/thermal roles |
AM Verarbeitbarkeit | Generally Easier (LPBF common) | More Challenging (High power/Reflectivity) | Impacts cost, lead time, choice of AM provider |
Kosten | Unter | Höher | Significant factor in budget constraints |
Typische Anwendung | Large radiators, Structural panels, Moderate heat sinks | High-performance heat sinks, Heat exchangers, Conductors | Match material to specific thermal load & function |
In Blätter exportieren
Die Rolle von Met3dp bei der Materialexzellenz:
A successful AM outcome for demanding applications like satellite heat dissipation panels begins with the quality of the raw material. Met3dp’s commitment to excellence in metal powder production is a key enabler for aerospace manufacturers.
- Advanced Atomization: Utilizing industry-leading gas atomization for alloys like AlSi10Mg ensures high sphericity, good flowability, controlled particle size distribution (PSD), and low oxygen content – all critical for achieving dense, reliable parts in LPBF.
- PREP-Technologie: For challenging or reactive materials potentially including specialized copper alloys or refractory metals sometimes considered for extreme temperature applications, Met3dp employs PREP technology. This electrode-based atomization method produces exceptionally spherical powders with very high purity and minimal satellite particles, ideal for demanding processes and critical applications.
- Qualitätskontrolle: Rigorous testing and characterization of powder batches ensure consistency, traceability, and adherence to stringent aerospace material specifications.
- Material-Portfolio: Met3dp manufactures a wide range of powders, including AlSi10Mg and potentially CuCrZr or other relevant alloys (like Ti alloys, superalloys also used in aerospace), providing a reliable source for wholesale metal powder procurement.
In conclusion, both AlSi10Mg and CuCrZr offer unique advantages for additively manufactured satellite heat dissipation panels. AlSi10Mg provides a lightweight, cost-effective solution suitable for many applications, while CuCrZr delivers superior thermal performance for high-heat-flux scenarios, albeit with higher density and processing complexity. The choice depends critically on the specific thermal requirements, mass budget, and structural demands of the application. Partnering with a knowledgeable AM provider like Met3dp, who not only understands the nuances of processing these materials but also supplies the high-quality powders essential for success, is key to unlocking the full potential of metal AM for advanced satellite thermal management.
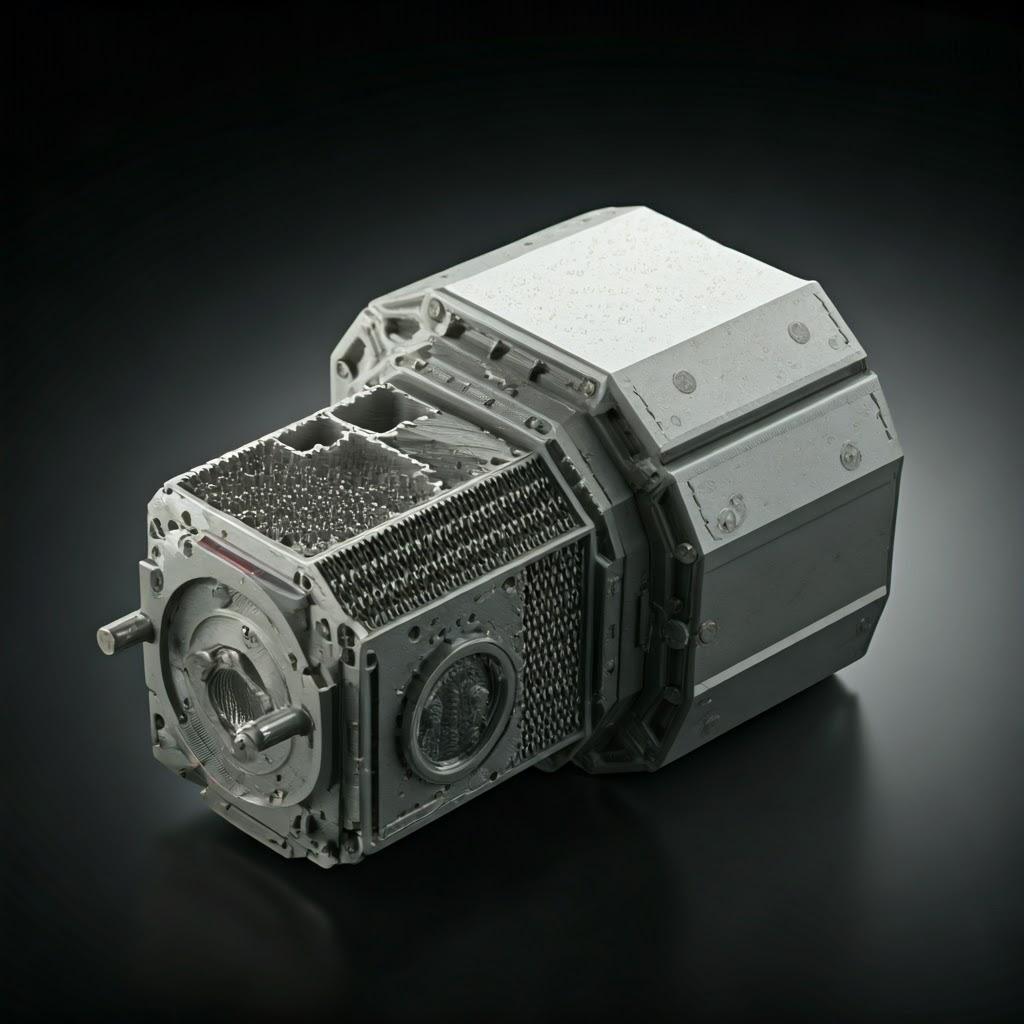
Design Considerations for Additively Manufactured Heat Dissipation Panels
The true power of metal additive manufacturing lies not just in its ability to build parts, but in its capacity to create components optimized in ways previously unimaginable. For satellite heat dissipation panels, leveraging Design for Additive Manufacturing (DfAM) principles is not just beneficial; it’s essential to unlock the full potential of the technology in terms of thermal performance, weight reduction, and overall system efficiency. DfAM is a mindset shift, moving beyond simply replicating traditionally designed parts with AM, towards designing für the unique capabilities and constraints of the layer-by-layer process. Engineers and designers working with AM-Lieferanten must embrace these principles early in the design cycle.
Leveraging DfAM for Thermal Superiority:
- Topology Optimization (TopOpt): This computational technique is revolutionary for designing lightweight, high-performance structures.
- Prozess: Finite Element Analysis (FEA) simulation software analyzes the thermal loads and structural requirements of the panel. Algorithms then iteratively remove material from areas of low stress or low thermal flux, leaving behind an optimized load path structure that efficiently transfers heat and withstands mechanical stresses with minimal mass.
- Das Ergebnis: Often results in organic, bio-inspired shapes that are highly efficient but impossible to manufacture conventionally. For heat dissipation panels, this means creating structures that channel heat directly to radiative surfaces or fluid channels while providing necessary stiffness, drastically reducing weight compared to solid or conventionally machined panels.
- Erwägungen: Requires specialized software and expertise. The resulting complex geometry needs careful evaluation for manufacturability within AM constraints (e.g., minimum feature size, support requirements). Collaborating with an aerospace AM supplier offering DfAM expertise is highly valuable.
- Lattice Structures and Cellular Materials: AM enables the direct fabrication of intricate internal lattice structures.
- Typen: Common types include strut-based lattices (like cubic, octet-truss) and surface-based lattices (like Triply Periodic Minimal Surfaces – TPMS, e.g., Gyroid, Schwarz).
- Benefits for Thermal Panels:
- Massive Surface Area Increase: Lattices offer exceptionally high surface area-to-volume ratios. If used within fluid channels, this enhances convective heat transfer. If used as part of the external radiating structure (though coatings are still key), they can increase effective emissivity over a given projected area with minimal mass.
- Gewichtsreduzierung: Lattices are inherently low density, replacing solid material with interconnected struts or shells, leading to substantial weight savings while maintaining tailored structural stiffness.
- Multifunctionality: Can be designed to manage fluid flow, provide structural support, and potentially even absorb vibration or impact energy.
- Design Choices: The choice of unit cell type, cell size, strut/wall thickness (relative density) allows engineers to tailor the mechanical properties (stiffness, strength) and thermal properties (effective conductivity, surface area) of the panel. TPMS lattices often offer smoother surfaces and better stress distribution compared to strut-based ones.
- Complex Internal Channel Design:
- Conformal Cooling/Heating: Design channels that precisely follow the contours of heat-generating components mounted to the panel, ensuring intimate thermal contact and efficient heat extraction. This is far superior to drilling straight holes in a machined block.
- Optimierte Flusspfade: Create smooth, curved channels with optimized cross-sections to minimize pressure drop and maximize heat transfer for liquid-cooled panels or heat pipe integration. Avoid sharp corners that can trap powder or impede flow.
- Manifold Integration: Complex inlet/outlet manifolds for fluid distribution can be seamlessly integrated into the panel design, reducing the need for external plumbing and potential leak points.
- Designing for AM Process Constraints: While AM offers freedom, it has its own rules. Ignoring them leads to build failures, defects, or excessive post-processing costs.
- Minimum Feature Size & Wall Thickness: Each AM machine and material combination has limits on the smallest features and thinnest walls it can reliably produce (often around 0.3-0.5 mm, but varies). Designs must respect these limits. Thin walls are desirable for weight but must be structurally sound and manufacturable.
- Overhangs and Support Structures: Features angled below a certain threshold relative to the build plate (typically <45 degrees for many metal LPBF processes) require support structures to prevent collapse during printing. Supports consume extra material, add print time, require removal effort (a significant cost driver), and can mar the surface. DfAM involves:
- Designing Self-Supporting Angles: Orienting features or using chamfers/fillets to exceed the critical overhang angle wherever possible.
- Optimizing Part Orientation: Choosing a build orientation that minimizes the volume of required supports, even if it slightly impacts build time or surface finish on certain faces.
- Designing for Support Removal: Ensuring supports are accessible for manual or machining-based removal. Internal supports in complex channels can be particularly challenging or impossible to remove, necessitating design modifications (e.g., diamond or teardrop shaped channels that are self-supporting).
- Entfernung von Puder: For parts with internal channels or cavities, ensuring complete removal of unfused powder after printing is critical. Designs should include strategically placed drain/access holes and avoid geometries that trap powder. Smooth internal surfaces help facilitate removal.
- Reststress-Management: AM involves rapid heating and cooling, leading to internal stresses. While process parameters and post-process heat treatments address this, design choices (e.g., avoiding large bulk masses connected by thin sections, using generous fillets) can help mitigate stress concentration and potential warping or cracking. Simulation tools can predict stress buildup and inform design adjustments.
- Merkmal Integration: Consolidate parts by integrating features directly into the AM design.
- Befestigungspunkte: Print bosses, threaded holes (may require post-machining/inserts), and alignment features directly.
- Connectors: Integrate ports for fluid or electrical connections.
- Stiffening Ribs: Add strategically placed ribs for structural integrity without adding excessive weight.
Engaging with an experienced AM partner like Met3dp early in the design phase is crucial. Their understanding of specific metal 3d printing processes like SEBM and LPBF, material behaviors (AlSi10Mg, CuCrZr), and the nuances of DfAM can help B2B customers optimize their satellite heat dissipation panel designs for manufacturability, performance, and cost-effectiveness. This collaborative approach ensures that the final design fully leverages AM’s capabilities while respecting its limitations.
Tolerance, Surface Finish, and Dimensional Accuracy in Metal AM
Achieving the required precision is non-negotiable for aerospace components. While metal AM offers incredible geometric freedom, understanding the achievable levels of tolerance, surface finish, and overall dimensional accuracy is critical for managing expectations and ensuring parts meet stringent satellite integration requirements. These factors are influenced by the chosen AM process, the material, part geometry, machine calibration, and post-processing steps. Aerospace procurement teams must specify these requirements clearly and work with capable precision metal component suppliers.
Maßgenauigkeit und Toleranzen:
- Typical Achievable Tolerances: Metal AM processes like Laser Powder Bed Fusion (LPBF) and Selective Electron Beam Melting (SEBM) can typically achieve dimensional accuracies in the range of:
- ±0.1 to ±0.2 mm for smaller dimensions (e.g., < 100 mm).
- ±0.1% to ±0.2% for larger dimensions.
- These are general guidelines; tighter tolerances can sometimes be achieved on specific features with careful process control and optimization, while complex geometries or large parts might exhibit slightly larger deviations. Met3dp’s focus on industry-leading equipment and process control aims to deliver high accuracy and reliability.
- Faktoren, die die Genauigkeit beeinflussen:
- Kalibrierung der Maschine: Regular and precise calibration of the printer’s lasers/electron beam, scanning system, and Z-axis movement is fundamental.
- Thermische Effekte: The rapid heating and cooling inherent in AM cause expansion and contraction. Residual stresses can build up, potentially leading to warping or distortion, especially in large or geometrically complex parts. This is a major source of dimensional deviation. SEBM, often performed at elevated chamber temperatures (as utilized by Met3dp for relevant materials), can help reduce residual stresses compared to some LPBF processes.
- Materialeigenschaften: Different alloys have different shrinkage rates and thermal conductivity, affecting solidification behavior and final dimensions.
- Geometrie und Größe des Teils: Large, bulky parts or those with significant variations in cross-section are more prone to thermal distortion. Internal stresses can accumulate differently depending on the shape.
- Scan-Strategie: The pattern used by the laser/electron beam to melt the powder layers impacts the thermal history and resulting microstructure and stresses.
- Unterstützende Strukturen: Supports help anchor the part and dissipate heat, influencing accuracy, but their removal can also affect final dimensions slightly.
- Enge Toleranzen einhalten: For critical interfaces, mounting points, or mating surfaces requiring tolerances tighter than standard AM capabilities, post-process CNC machining is typically employed. This combines the geometric freedom of AM with the high precision of subtractive machining.
Oberflächengüte (Rauhigkeit):
- Oberflächenrauhigkeit (Ra) im Ist-Zustand: The surface finish of parts directly out of the AM machine is inherently rougher than machined surfaces. Typical Ra values depend heavily on:
- Prozess: LPBF generally produces slightly smoother surfaces than SEBM due to finer powder sizes.
- Material: Powder characteristics influence melt pool behavior and surface texture.
- Orientierung: Surfaces parallel to the build plate (upward-facing) tend to be smoother than vertical walls, which are smoother than downward-facing surfaces or those requiring support structures. Downward-facing surfaces often exhibit the highest roughness due to the partially melted powder particles adhering to them.
- Parameter: Layer thickness, beam power, scan speed all play a role.
- Typische Ra-Werte: As-built Ra for metal AM often ranges from 5μm to 25μm (200μin to 1000μin), but this can vary significantly.
- Einfluss der Oberflächenbeschaffenheit:
- Thermal Radiation: Surface finish, particularly after coating, significantly impacts the emissivity and absorptivity of radiator panels. While the intrinsic material roughness might be secondary to the coating properties, a controlled surface is needed for consistent coating adhesion and performance.
- Fluid Flow: For internal channels in liquid-cooled panels or heat pipes, rough surfaces can increase pressure drop and potentially affect heat transfer coefficients.
- Assembly: Rough surfaces on mating interfaces can affect fit and thermal contact resistance.
- Erreichen der gewünschten Oberflächengüte: If the as-built roughness is insufficient, various post-processing techniques are used:
- Abrasives Strahlen (Perlen-/Sandstrahlen): Provides a uniform matte finish, effective for removing partially sintered particles and improving cosmetic appearance. Ra values typically improve to the 3−10μm range.
- Taumeln/Gleitschleifen: Uses abrasive media in a tumbling barrel or vibratory bowl to smooth surfaces and deburr edges, particularly effective for batches of smaller parts.
- Polieren (manuell oder automatisiert): Can achieve very smooth, mirror-like finishes (Ra < 0.8μm or better) but is often labor-intensive or requires complex robotic setups, especially for complex shapes.
- Chemical Etching/Electropolishing: Can smooth surfaces, especially complex internal channels, but requires careful control and material compatibility.
- CNC-Bearbeitung: Used for specific surfaces requiring high smoothness and tight tolerances.
Das Engagement von Met3dp für Präzision:
Achieving consistent dimensional accuracy and predictable surface finishes requires rigorous process control and advanced equipment. Met3dp utilizes industry-leading printing systems, potentially including high-precision SEBM technology known for producing parts with low residual stress and good accuracy in suitable materials. Coupled with their expertise in powder quality control and optimized build parameters, Met3dp is committed to delivering parts that meet the demanding specifications of the aerospace industry. They understand the importance of working closely with B2B-Kunden to define achievable tolerances and finishes, incorporating necessary post-processing steps to guarantee compliance for mission-critical satellite components. When evaluating AM service providers, inquiring about their quality management systems, machine capabilities, and track record with precision aerospace parts is essential for procurement teams.
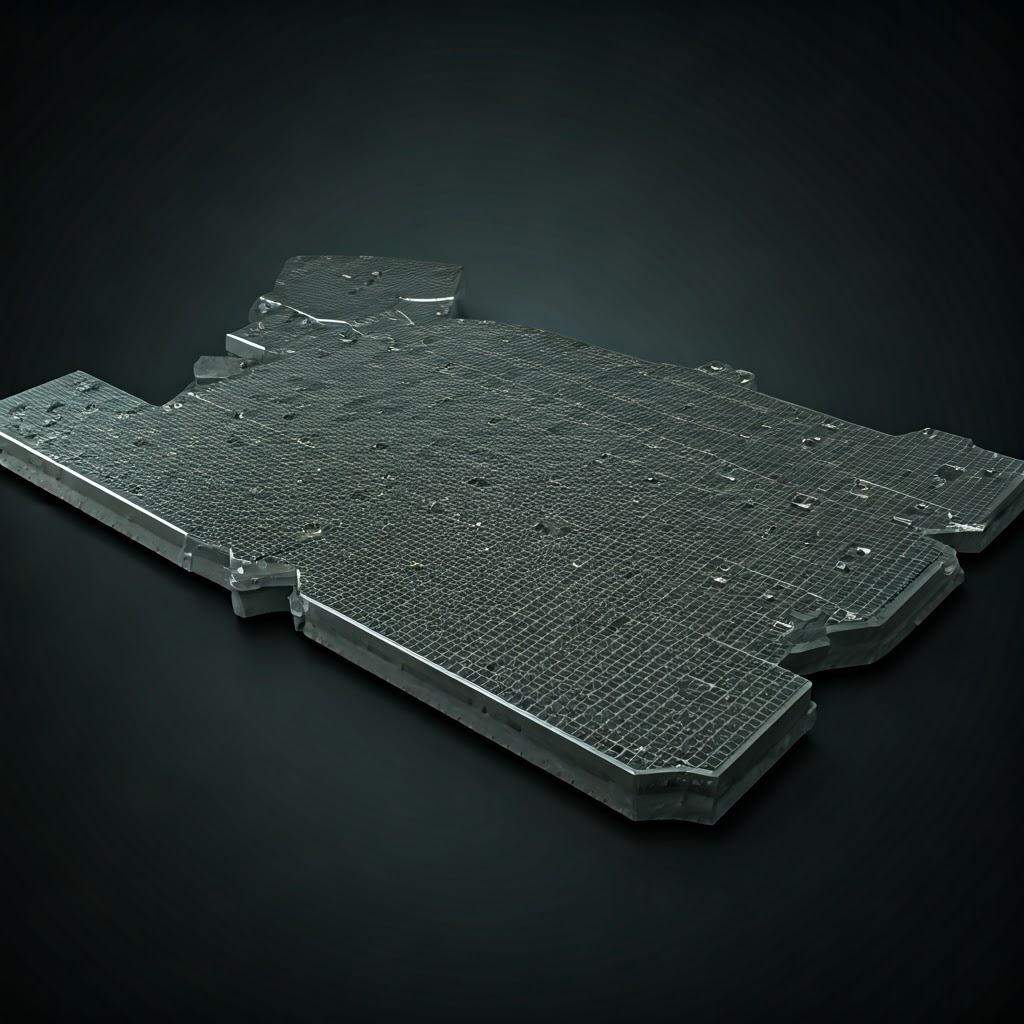
Post-Processing Requirements for AM Satellite Panels
A common misconception about metal additive manufacturing is that parts come out of the machine ready to use. In reality, especially for demanding applications like aerospace, the printing process is often just the first major step. A series of crucial post-processing steps are typically required to transform the as-built part into a functional, reliable satellite heat dissipation panel that meets all performance, quality, and integration specifications. These steps add cost and time but are non-negotiable for ensuring the component’s integrity and functionality in the harsh environment of space. Wholesale AM production planning must account for these essential stages.
Essential Post-Processing Steps:
- De-powdering: Carefully removing all unfused metal powder from the build chamber and, critically, from the part itself. This includes loose powder on surfaces and trapped powder within internal channels or complex lattice structures. Methods include brushing, vacuuming, bead blasting, and specialized vibration or flushing systems for internal geometries. Incomplete powder removal can compromise performance, add weight, and pose risks if particles dislodge during operation. Design for de-powdering (access holes, smooth channels) is vital.
- Stressabbau: As-built metal AM parts contain significant residual stresses due to the rapid heating and cooling cycles. A stress relief heat treatment, performed while the part may still be attached to the build plate, is often the first thermal step. This involves heating the part to a specific temperature (below critical transformation points), holding it for a period, and then slowly cooling it. This reduces internal stresses, minimizing the risk of distortion during subsequent removal from the build plate or during machining, and preventing premature failure under load. Specific cycles depend on the alloy (e.g., AlSi10Mg vs. CuCrZr).
- Entnahme von der Bauplatte: Separating the printed part(s) from the metal build plate they were fused to. This is typically done using wire Electrical Discharge Machining (EDM) or a band saw. Care must be taken to avoid damaging the part.
- Entfernung der Stützstruktur: Removing the support structures required during the build process. This can be a labor-intensive step, depending on the complexity and accessibility of the supports.
- Manuelle Entfernung: Breaking or cutting away supports using hand tools. Suitable for easily accessible structures.
- Bearbeitungen: Using CNC milling or grinding to remove supports, especially useful for achieving a clean surface finish at the support interface points.
- Drahterodieren: Can sometimes be used for precise removal in tight areas.
- Design Impact: Minimizing the need for supports through DfAM significantly reduces this effort and cost.
- Solution Annealing and Aging (Heat Treatment): Beyond stress relief, further heat treatments are often necessary to achieve the desired final material properties (strength, ductility, hardness, thermal conductivity).
- AlSi10Mg: Typically undergoes a T6 cycle involving solution treatment (heating to dissolve precipitates), quenching (rapid cooling), and artificial aging (re-heating to a lower temperature to precipitate strengthening phases). This significantly improves mechanical strength.
- CuCrZr: Requires solution treatment followed by aging to develop the chromium and zirconium precipitates that provide its strength while largely maintaining high conductivity.
- Prozesskontrolle: Accurate furnace control, appropriate atmospheres (inert or vacuum to prevent oxidation), and precise timing are crucial for successful heat treatment. This requires specialized equipment and expertise often found with experienced Zulieferer der Luft- und Raumfahrtindustrie or dedicated B2B heat treatment service providers.
- Oberflächenveredelung: As discussed previously, achieving the required surface smoothness (Ra) often requires additional steps beyond basic de-powdering.
- Abrasive Blasting: Gemeinsam für ein einheitliches, mattes Finish.
- Taumeln/Gleitschleifen: For smoother finishes and deburring, suitable for batch processing.
- Polieren: For very low Ra requirements on specific surfaces.
- Chemical/Electropolishing: For smoothing complex internal or external surfaces.
- CNC-Bearbeitung: For critical dimensions, tolerances, and surface finishes that cannot be achieved by AM alone. This includes:
- Mating Interfaces: Ensuring flat, smooth surfaces for mounting electronics or integration with other structures, minimizing thermal contact resistance.
- Threaded Holes: Creating precise threads for fasteners (often using thread inserts like Helicoils for softer alloys like aluminum).
- Versiegeln von Oberflächen: Achieving the necessary smoothness and flatness for fluid or vacuum seals.
- Precise Diameters/Bores: Machining holes or features to tight tolerance requirements.
- Thermisch kontrollierte Beschichtungen: Satellite radiator surfaces are almost always coated to tailor their thermo-optical properties.
- Zweck: Achieve high thermal emissivity (efficiently radiating heat, typically > 0.85) and low solar absorptivity (minimizing heat gain from the sun, typically < 0.2).
- Common Coatings: Specialized white paints (e.g., polyurethane or silicone-based with pigments like AZ-93), Silvered Teflon (Optical Solar Reflectors – OSRs), or potentially newer technologies like nanostructured surfaces.
- Anwendung: Requires specialized cleaning and coating processes performed in controlled environments to ensure adhesion and performance longevity in the space environment. Requires working with qualified satellite thermal coatings suppliers.
- Reinigung: Final cleaning procedures to ensure the part is free from contaminants (oils, residues from handling or machining, loose particles) before integration.
- Zerstörungsfreie Prüfung (NDT) und Inspektion: Rigorous inspection is mandatory for aerospace components to ensure integrity and quality.
- Prüfung der Abmessungen: Using Coordinate Measuring Machines (CMM), 3D scanning, or traditional metrology tools to verify dimensions and tolerances.
- Internal Structure Inspection: X-ray Computed Tomography (CT scanning) is invaluable for AM parts, allowing visualization of internal geometries (channels, lattices), detection of internal defects (porosity, inclusions), and verification of powder removal.
- Surface Defect Inspection: Visual inspection, Dye Penetrant Inspection (DPI), or Magnetic Particle Inspection (MPI, for ferromagnetic materials) to detect surface cracks or flaws.
- Dichtheitsprüfung: For panels involving fluid channels or heat pipes, pressure testing or helium leak testing ensures integrity.
- Material Verification: Positive Material Identification (PMI) or other material testing may be required.
Integrierte Lösungen:
Managing this complex chain of post-processing steps requires significant logistical coordination and technical expertise. Some B2B additive manufacturing service providers, like potentially Met3dp or their close partners, may offer vertically integrated solutions, managing many of these steps in-house or through a network of qualified wholesale post-processing partners. This simplifies the supply chain for the customer, ensuring accountability and streamlined workflow from initial design consultation to final, inspected, and coated part delivery. When selecting an AM partner, understanding their post-processing capabilities and quality management system is just as important as their printing technology.
Common Challenges in AM for Heat Dissipation Panels and How to Avoid Them
While metal additive manufacturing offers transformative potential for satellite components, it’s not without its challenges. Being aware of potential issues and implementing mitigation strategies during design, simulation, printing, and post-processing is crucial for successful outcomes, particularly given the high-stakes nature of aerospace applications. Partnering with an experienced AM-Lieferant like Met3dp, who understands these challenges and possesses the technology and expertise to overcome them, is paramount.
Zentrale Herausforderungen und Strategien zur Abhilfe:
- Warping and Distortion (Residual Stress):
- Herausforderung: The rapid, localized heating and cooling during AM creates significant thermal gradients, leading to internal stresses within the part. As these stresses accumulate layer by layer, they can cause the part to warp, distort, or even detach from the build plate, compromising dimensional accuracy. This is particularly prevalent in large parts or designs with varying cross-sections.
- Milderung:
- Simulation: Use process simulation software to predict thermal gradients and stress accumulation based on the part geometry and scan strategy. Modify the design, orientation, or support structures proactively.
- Optimierte Unterstützungsstrukturen: Design robust supports to anchor the part securely to the build plate and help conduct heat away, minimizing distortion.
- Build Plate Heating: Pre-heating the build plate (common in many LPBF machines and standard at higher temperatures in SEBM) reduces thermal gradients between the solidified material and the new layer, significantly lowering residual stress. Met3dp’s potential use of SEBM leverages this benefit.
- Optimierte Scan-Strategien: Employ specific scan patterns (e.g., island scanning, alternating hatch directions) to distribute heat input more evenly and reduce stress buildup.
- Stressabbau Wärmebehandlung: Performing a stress relief cycle immediately after printing (often before removing the part from the build plate) is critical to relax internal stresses.
- Unvollständige Entfernung des Pulvers:
- Herausforderung: Unfused powder can become trapped within intricate internal channels, complex lattice structures, or small cavities. If not fully removed, this adds unwanted mass, can impede fluid flow or heat transfer, and potentially dislodge during operation, causing contamination or damage.
- Milderung:
- DfAM für die Pulverentfernung: Design internal channels with smooth paths, sufficient diameters, and strategically placed drain/access holes. Avoid dead-end cavities. Teardrop or diamond channel cross-sections can be self-supporting and easier to clear than simple circular ones.
- Optimierte Ausrichtung: Orient the part on the build plate to facilitate powder drainage after the build.
- Thorough Post-Processing: Employ rigorous de-powdering techniques (vibration, compressed air/inert gas blowing, potentially ultrasonic cleaning or flushing for specific designs).
- Inspektion: Use CT scanning or borescopes to verify complete powder removal from critical internal passages.
- Porosität:
- Herausforderung: Small voids or pores within the printed material can act as stress concentrators, reducing mechanical strength, fatigue life, and potentially compromising leak-tightness. Porosity can arise from gas trapped within the powder or melt pool (gas porosity) or from incomplete melting between layers or scan tracks (lack of fusion porosity).
- Milderung:
- Hochwertiges Pulver: Use powder with high sphericity, low internal gas content, controlled particle size distribution, and low satellite presence. Met3dp’s focus on advanced powder production using gas atomization and PREP directly addresses this, ensuring optimal powder characteristics. Sourcing from a reliable B2B metal powder distributor with strong quality control is essential.
- Optimierte Prozessparameter: Fine-tuning laser/electron beam power, scan speed, layer thickness, and hatch spacing is crucial to ensure complete melting and fusion. Parameter development is key for each specific alloy and machine.
- Kontrollierte Atmosphäre: Processing in a high-purity inert gas environment (LPBF) or vacuum (SEBM, as used by Met3dp) minimizes oxygen pickup and reduces gas porosity.
- Heiß-Isostatisches Pressen (HIP): For applications requiring maximum density and fatigue life (though potentially adding cost and complexity), HIP can be used as a post-processing step to close internal pores through high pressure and temperature.
- NDT: Use CT scanning to detect and quantify internal porosity.
- Knacken:
- Herausforderung: Some alloys, particularly certain aluminum or nickel superalloys, can be susceptible to solidification cracking or liquation cracking during the rapid heating/cooling cycles of AM, especially under high residual stress conditions.
- Milderung:
- Auswahl der Legierung: Choose alloys known to have good weldability/printability (AlSi10Mg is generally good; some other Al alloys can be more challenging).
- Optimierung der Parameter: Adjusting parameters (e.g., pre-heating, scan strategy) can modify the thermal history to reduce cracking susceptibility.
- Stress Management: Implementing effective stress mitigation techniques (build plate heating, supports, stress relief) is crucial.
- Entwurf: Avoid sharp corners or abrupt changes in geometry that act as stress raisers.
- Achieving Leak-Tightness:
- Herausforderung: For heat dissipation panels involving fluid channels or integrated heat pipes, ensuring the component is completely leak-tight is critical. Porosity, micro-cracks, or defects at interfaces can lead to leaks.
- Milderung:
- Robuste Prozesskontrolle: Ensuring dense, defect-free printing through powder quality, optimized parameters, and controlled atmosphere.
- Angemessenes Design: Ensuring sufficient wall thickness for channels.
- Nachbearbeiten: HIP can help close potential leak paths. Careful machining of sealing surfaces is needed.
- Strenge Tests: Implementing thorough leak testing (e.g., pressure decay test, helium mass spectrometry) as part of the quality assurance protocol.
- Kontrolle der Oberflächengüte:
- Herausforderung: Achieving the specific surface finish required for thermal radiation, fluid dynamics, or assembly interfaces directly from the AM process can be difficult due to the inherent roughness.
- Milderung:
- Understanding As-Built Limits: Recognize the typical Ra achievable for the process and material, and design accordingly.
- Targeted Post-Processing: Plan for necessary surface finishing steps (blasting, polishing, machining) on critical surfaces as part of the manufacturing workflow. Budget and schedule accordingly.
Successfully navigating these challenges requires a combination of careful design, material science understanding, precise process control, thorough post-processing, and rigorous quality assurance. Working with an experienced additive manufacturing B2B partner like Met3dp, which combines expertise in materials (high-quality powders), processes (advanced SEBM/LPBF printers), and potentially integrated post-processing and quality control, provides the best foundation for mitigating these risks and reliably producing high-performance satellite heat dissipation panels.
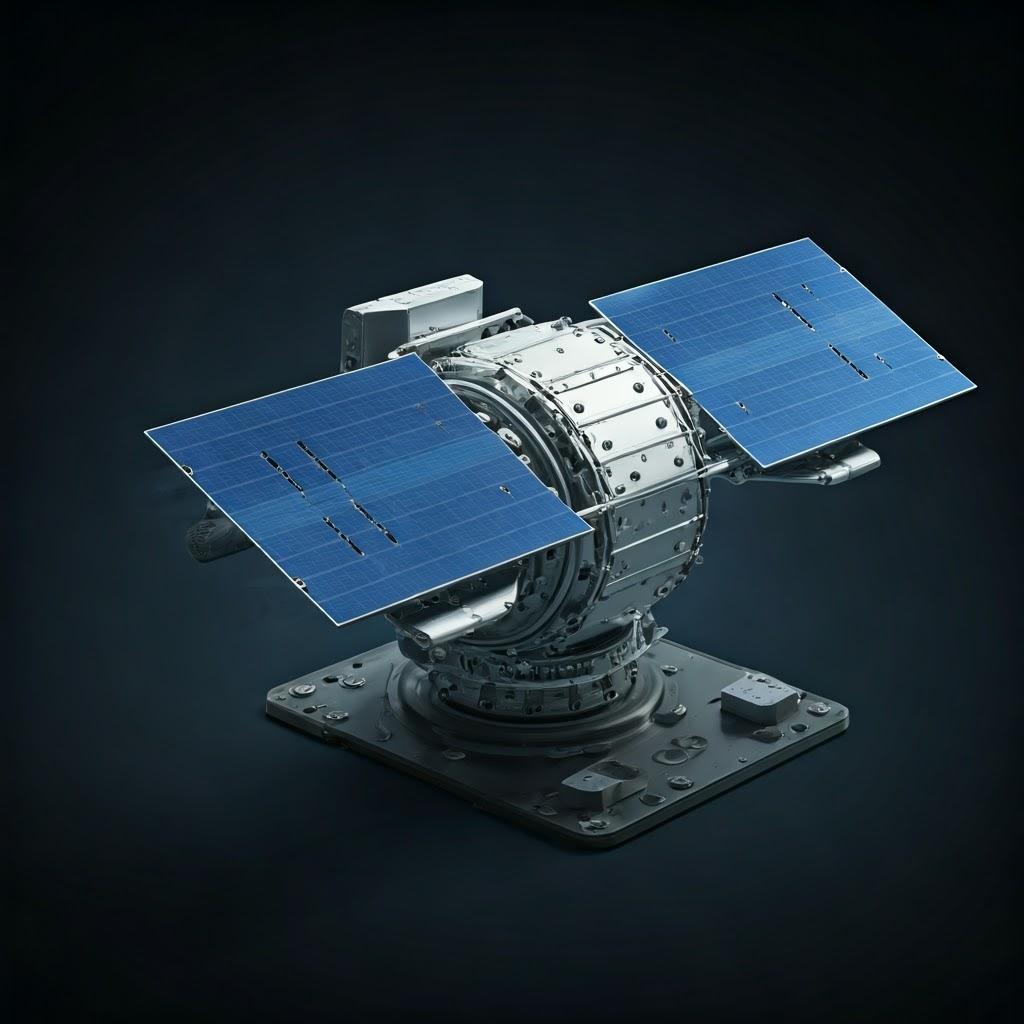
How to Choose the Right Metal 3D Printing Service Provider for Aerospace Components
Selecting the right manufacturing partner is always critical, but the stakes are exceptionally high when dealing with aerospace components produced using advanced technologies like metal additive manufacturing. The performance and reliability demands of satellite systems leave no room for error. For engineers and Beschaffungsmanager tasked with sourcing AM heat dissipation panels, choosing a service provider requires rigorous evaluation beyond just price. It necessitates finding a true B2B partner with demonstrated expertise, robust processes, and a deep understanding of aerospace requirements.
Here’s a comprehensive checklist to guide your selection process when evaluating potential metal AM suppliers for mission-critical satellite hardware:
1. Aerospace Certifications and Quality Management System (QMS): * AS9100-Zertifizierung: This is the gold standard QMS for the Aviation, Space, and Defense industries. Certification to AS9100 (or equivalent) demonstrates a provider’s commitment to quality, traceability, risk management, and continuous improvement processes specifically tailored for aerospace. Non-negotiable for critical flight hardware. * Robustes QMS: Beyond the certificate, evaluate the maturity and implementation of their QMS. Ask about their documentation control, process control procedures, corrective action processes, and how they ensure consistency from batch to batch. * NADCAP-Akkreditierung: While not always required for basic AM part production, NADCAP (National Aerospace and Defense Contractors Accreditation Program) accreditation may be relevant if the supplier performs critical in-house special processes like heat treatment, NDT, or chemical processing/coating.
2. Proven Experience and Expertise: * Aerospace Track Record: Have they successfully produced parts for previous space missions or demanding aerospace applications? Can they provide (non-confidential) case studies or references? Experience matters significantly in navigating the unique challenges of space hardware. * Fachwissen über Werkstoffe: Do they have extensive, demonstrated experience processing the specific alloys required (e.g., AlSi10Mg, CuCrZr)? Understand their experience with parameter development, achieving desired material properties, and handling potential issues with these materials. Ask about their metal powder sourcing – do they use high-quality powder from reputable Distributoren or manufacturers like Met3dp, or even produce their own? * DfAM-Unterstützung: Does the provider offer Design for Additive Manufacturing consultation? Can their engineers work collaboratively with your team to optimize the design for performance, manufacturability, and cost-effectiveness, leveraging AM’s unique benefits?
3. Technological Capability and Capacity: * Geeignete AM-Technologie: Do they operate the right type of AM machines for your material and application needs (e.g., LPBF for general AlSi10Mg, potentially high-power LPBF or SEBM for CuCrZr)? Met3dp’s potential expertise in SEBM, for instance, could be advantageous for certain materials requiring high temperature processing and low residual stress. * Maschinenpark: What is the size and age of their machine fleet? Do they have sufficient capacity to handle your project timelines, including potential Großaufträge oder wholesale production runs? What is the build volume of their machines – can they accommodate the size of your heat dissipation panels? * Machine Calibration and Maintenance: What are their procedures for ensuring machine accuracy and consistency? Regular calibration and preventative maintenance are crucial for reliable output.
4. In-House vs. Outsourced Post-Processing: * Comprehensive Capabilities: Does the provider offer the necessary post-processing steps in-house (stress relief, heat treatment, support removal, basic surface finishing, potentially CNC machining, NDT)? An integrated workflow often leads to better control, accountability, and potentially faster lead times. * Managed Partner Network: If they outsource certain steps (e.g., specialized coatings, advanced NDT, complex machining), do they have a well-vetted network of qualified wholesale post-processing partners? How do they manage quality and traceability across this supply chain? Understand their process for managing external vendors.
5. Material Traceability and Testing: * Powder Control: How do they manage and track metal powder batches from sourcing through production? Full traceability is essential in aerospace. Ask about their powder testing and recycling procedures. Using high-quality powders, like those produced by Met3dp using advanced atomization, provides a strong starting point. * Überprüfung der Materialeigenschaften: Do they have capabilities for testing material coupons printed alongside the parts to verify mechanical properties, microstructure, and density meet specifications?
6. Project Management and Communication: * Clear Communication: Is their team responsive, knowledgeable, and easy to communicate with? Establishing a good working relationship is vital for complex projects. * Projektleitung: Do they have clear processes for project intake, status updates, and managing deliverables?
7. Cost and Lead Time (See Next Section): * Transparent Quoting: Do they provide detailed, transparent B2B quotes that break down the costs? * Realistische Vorlaufzeiten: Can they provide reliable lead time estimates based on current capacity and project complexity?
Met3dp as Your Potential Partner: Companies like Met3dp, specializing in both advanced 3D-Druck von Metall equipment and high-performance metal powders, are uniquely positioned. Their potential vertical integration – understanding powder production (gas atomization, PREP), machine operation (potentially SEBM and LPBF), and application development – provides a deep, fundamental understanding of the entire AM process chain. When evaluating partners, consider suppliers like Met3dp who bring this comprehensive expertise, focusing on quality control from the raw material through to the final part, making them a strong candidate for producing mission-critical satellite components. Thoroughly vetting potential suppliers against these criteria will help ensure you select a partner capable of delivering the high-quality, reliable AM heat dissipation panels your satellite mission demands.
Cost Factors and Lead Time for AM Satellite Heat Dissipation Panels
While the performance benefits of additively manufactured satellite components are compelling, understanding the associated costs and typical lead times is crucial for project planning, budgeting, and effective B2B-Beschaffung. Metal AM involves different cost drivers compared to traditional manufacturing, and lead times can vary significantly based on complexity and workflow. Getting accurate AM quotes requires providing detailed information to potential Lieferanten.
Die wichtigsten Kostentreiber:
- Materialkosten:
- Pulver Preis: The cost per kilogram of aerospace-grade metal powder varies significantly by alloy. Common alloys like AlSi10Mg are generally less expensive than high-conductivity copper alloys like CuCrZr or specialized superalloys. The price is influenced by raw material costs, production complexity (e.g., atomization method), and quality specifications. Sourcing metal powder wholesale can offer cost benefits for larger volumes.
- Teilvolumen und Dichte: The sheer volume of material required for the part directly impacts cost. Denser materials like CuCrZr (8.9g/cm3) will inherently cost more for the same volume compared to AlSi10Mg (2.67g/cm3).
- Unterstützungsstruktur Volumen: Material used for support structures also adds to the cost. Minimizing supports through DfAM is key.
- Buy-to-Fly Ratio: AM generally has a much better buy-to-fly ratio (ratio of raw material purchased to the weight of the final part) than subtractive machining, reducing waste, especially for complex parts and expensive materials.
- Maschinenzeit:
- Vorbereitung des Baus: Time spent by engineers setting up the build file (orientation, supports, slicing, parameter assignment) using specialized software.
- Druckzeit: The core cost factor, determined by the total volume of material being fused (part + supports) and, more directly, the number of layers (part height in the build orientation). Taller parts take longer. Machine hourly rates vary based on the technology (LPBF, SEBM), size, and sophistication of the printer.
- Machine Utilization: Costs are often calculated based on booking time on expensive capital equipment. Efficient nesting of multiple parts in a single build can reduce per-part machine time cost for Großaufträge.
- Arbeitskosten:
- Setup & Teardown: Labor involved in preparing the machine, loading powder, overseeing the build, and removing the completed build plate and parts.
- Nachbearbeitungsarbeiten: Dies kann ein very significant cost component. Manual support removal, de-powdering complex parts, surface finishing (especially manual polishing), inspection, and assembly (if needed) all require skilled labor hours. The complexity of the part heavily influences this.
- Support Structure Complexity:
- Lautstärke: More supports mean more material cost and potentially longer print times.
- Removal Difficulty: Supports in hard-to-reach areas or on delicate features require more time and skill to remove cleanly without damaging the part, driving up labor costs.
- Nachbearbeitungsintensität:
- Wärmebehandlung: Costs associated with furnace time, energy consumption, and potentially specialized atmospheres (vacuum, inert gas). Complex multi-step cycles take longer and cost more.
- CNC-Bearbeitung: Costs depend on the number of features being machined, the required tolerances, surface finish, setup time, and machine time.
- Oberflächenveredelung: Costs vary greatly depending on the method (blasting is relatively inexpensive; extensive polishing is expensive) and the required final Ra value.
- Beschichtung: Specialized thermal control coatings require specific expertise and facilities, adding significant cost.
- Qualitätssicherung und Inspektion:
- NDT: Costs for CT scanning, DPI, leak testing, etc., depend on the level of inspection required by aerospace standards and project specifications. 100% inspection is common for critical parts.
- Prüfung der Abmessungen: CMM or 3D scanning time and analysis.
- Dokumentation: Time spent generating required quality documentation and certifications.
- Auftragsvolumen:
- Prototypen: Typically have a higher per-part cost due to setup overheads spread over a small quantity.
- Production Runs: Larger Großaufträge allow for amortization of setup costs, potential optimization of build layouts (nesting), and can sometimes leverage Großhandelspreisgestaltung structures, leading to a lower per-part cost.
Typische Vorlaufzeiten:
Lead times for AM parts can vary widely, from days for simple prototypes to many weeks or months for complex production parts requiring extensive post-processing and qualification.
- Prototyping: Simple AlSi10Mg parts might be produced in 1-3 weeks, depending on machine availability and basic post-processing needs. More complex prototypes or those using challenging materials like CuCrZr could take longer.
- Produktionsteile: For qualified aerospace components, lead times of 6-12 weeks or more are common, factoring in:
- Detailed planning and potential DfAM iteration.
- Printing time (can be multiple days for large/complex builds).
- Full post-processing chain (heat treatments, machining, finishing, coating can each add days or weeks).
- Rigorous NDT and inspection procedures.
- Queue times at the AM-Lieferant and any external post-processing partners.
- Factors Influencing Speed: Part complexity, size, material choice, required post-processing steps, required quality level/documentation, and the supplier’s current workload all impact the final delivery date.
Getting Accurate B2B Quotes:
To receive a meaningful additive manufacturing quote from a supplier like Met3dp, provide as much information as possible:
- CAD-Modell: 3D model in a standard format (e.g., STEP).
- 2D Drawings: If specific tolerances, surface finishes, or critical dimensions are required beyond standard AM capabilities.
- Spezifikation des Materials: Clearly state the alloy (e.g., AlSi10Mg, CuCrZr) and any required standards (e.g., AMS specifications).
- Menge: Number of parts required (specify if prototype or production volume).
- Tolerance Requirements: Define critical dimensional tolerances.
- Anforderungen an die Oberflächenbeschaffenheit: Specify required Ra values for specific surfaces.
- Post-Processing-Bedarf: Detail all required steps (heat treatment cycle, machining operations, specific surface finish, coating type).
- Prüfung und Zertifizierung: List all required NDT, inspection, and certification requirements (e.g., CMM report, material certs, AS9100 compliance).
- Desired Delivery Date: Provide your required timeline.
Providing comprehensive information allows potential B2B-Lieferanten to accurately assess the scope of work and provide a realistic cost and lead time estimate.
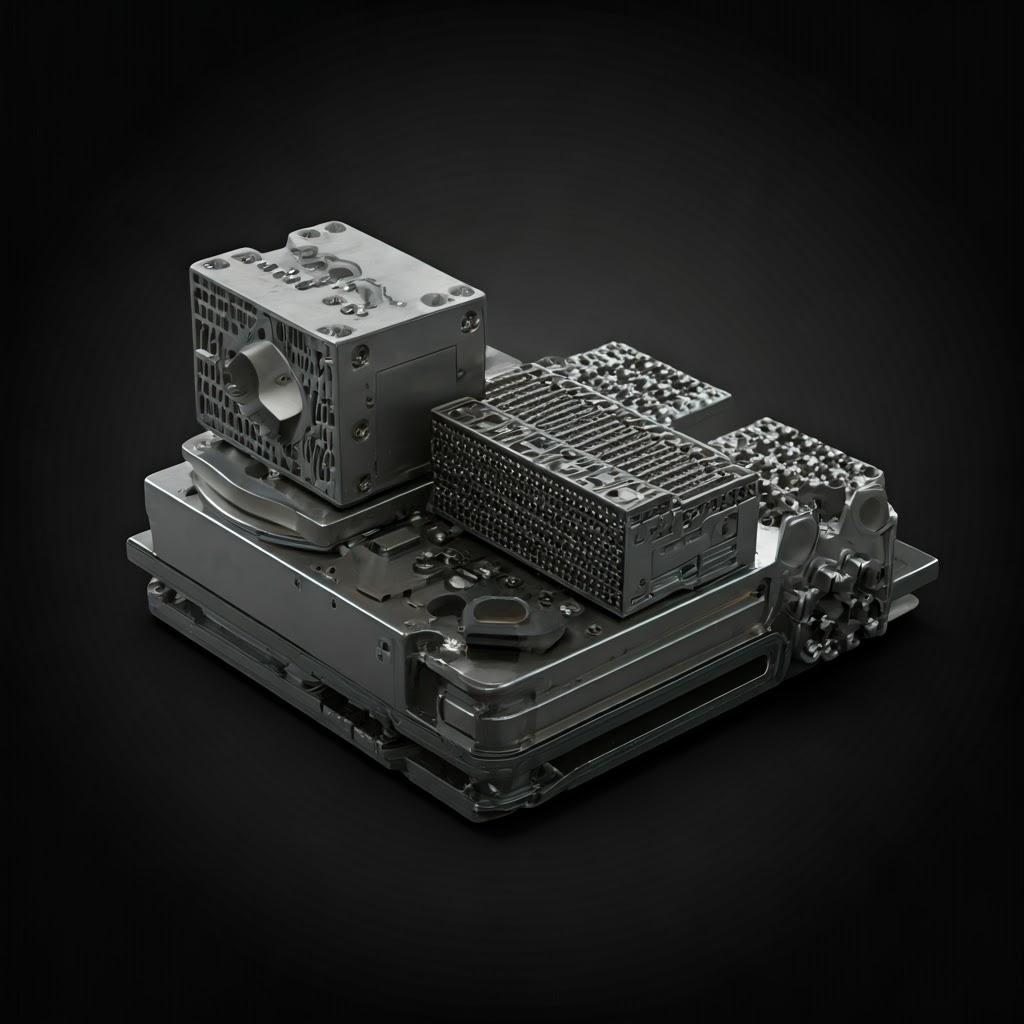
Häufig gestellte Fragen (FAQ)
Here are answers to some common questions engineers and procurement managers have about using metal AM for satellite heat dissipation panels:
Q1: What are the primary benefits of using AM for satellite radiators compared to traditional methods?
- A1: The key benefits stem from AM’s design freedom:
- Enhanced Thermal Performance: Ability to create complex geometries like internal lattices or conformal channels significantly increases surface area for heat transfer, often leading to more efficient cooling within the same volume or mass constraints compared to simpler machined or assembled designs.
- Significant Weight Reduction: Topology optimization and lattice structures allow for placing material only where needed for thermal and structural requirements, drastically reducing component mass, which is critical for lowering launch costs.
- Teil Konsolidierung: Multiple components (e.g., panel, brackets, manifolds) can often be combined into a single, monolithic AM part, reducing assembly complexity, potential failure points (joints, welds), and simplifying the supply chain.
- Schnelle Prototypenerstellung und Anpassung: AM enables faster iteration during the design phase and efficient production of custom or low-volume parts tailored to specific mission needs without the high tooling costs of traditional methods.
Q2: How does the thermal performance of AM AlSi10Mg or CuCrZr compare to traditionally manufactured parts?
- A2: When processed correctly and subjected to appropriate post-process heat treatments, the bulk material properties (including thermal conductivity) of AM AlSi10Mg and CuCrZr can be very close to their cast or wrought counterparts. For AlSi10Mg, T6 heat treatment is crucial for optimizing conductivity. For CuCrZr, proper solutionizing and aging achieve high conductivity. While as-built AM parts might initially have slightly different microstructures, post-processing aims to homogenize properties. Crucially, the system-level thermal performance of an AM heat dissipation panel often exceeds that of traditional designs due to the ability to create highly optimized geometries (enhanced surface area, optimized flow paths) that simply cannot be made any other way. The design freedom enabled by AM is the key performance differentiator.
Q3: What quality certifications should I look for in a B2B supplier of AM satellite components?
- A3: The most important certification is AS9100. This signifies the supplier operates a Quality Management System specifically designed for the rigorous demands of the Aviation, Space, and Defense industries, covering aspects like traceability, risk management, process control, and configuration management. Depending on the scope of work, NADCAP accreditation for special processes (like heat treatment, NDT, welding/brazing if applicable to assembly, coatings) performed in-house might also be relevant or required by the prime contractor. ISO 9001 is a general QMS standard but is usually considered insufficient on its own for critical aerospace hardware. Always verify the scope and validity of certifications.
Q4: Can Met3dp handle bulk orders for satellite heat dissipation panels?
- A4: Met3dp is positioned as a provider of both industrial AM equipment and high-quality metal powders, indicating a focus on enabling industrial-scale additive manufacturing. While specific production capacity details would need direct discussion, companies like Met3dp, with their foundation in AM technology and materials, are typically structured to support Großhandel und Großbestellung requirements for B2B customers. It’s recommended to contact Met3dp directly to discuss specific volume needs, production scheduling, and capabilities for scaling from prototypes to serial production.
Q5: What information is needed to get an accurate quote for an AM heat dissipation panel project from a distributor or supplier?
- A5: To get the most accurate B2B quote, provide the following:
- 3D-CAD-Modell (bevorzugt STEP-Format).
- 2D drawings calling out critical dimensions, tolerances, and surface finishes.
- Specified material (e.g., AlSi10Mg, CuCrZr) and any relevant material standards.
- Required quantity (and whether it’s for prototyping or production).
- Detailed post-processing requirements (heat treatment cycle, specific surfaces to be machined, required Ra values, coating specifications).
- All required testing, inspection (e.g., CT scanning, leak testing), and certification documentation.
- Desired delivery timeline. The more detail provided upfront, the faster and more accurately the supplier can assess the project and provide a reliable quotation.
Conclusion: Elevating Satellite Performance with Advanced Metal AM Heat Dissipation
The thermal challenges faced by modern satellites demand innovative solutions. As spacecraft become more powerful, compact, and operate in increasingly demanding environments, efficient and reliable heat dissipation is more critical than ever. Traditional manufacturing methods, while mature, often struggle to deliver the complex geometries and optimized structures needed to meet these escalating requirements within stringent mass budgets.
Metal additive manufacturing represents a fundamental shift, offering unparalleled design freedom to create satellite heat dissipation panels that are lighter, more efficient, and highly integrated. By leveraging techniques like topology optimization and lattice structures, AM enables engineers to design thermal management components previously confined to the realm of theory. Materials like lightweight, versatile AlSi10Mg and high-conductivity CuCrZr, when processed using controlled AM techniques and appropriate post-processing, provide the material properties needed for demanding space applications.
However, harnessing the full potential of AM requires more than just access to a printer. It demands expertise in Design for Additive Manufacturing, a deep understanding of material science, precise process control, rigorous post-processing capabilities, and an unwavering commitment to quality assurance – all within the framework of aerospace industry standards. Choosing the right manufacturing partner, one with proven aerospace experience, the right certifications (like AS9100), advanced technological capabilities, and comprehensive support, is paramount to success.
Met3dp, with its foundation in both advanced AM systems and the production of high-quality metal powders using industry-leading techniques like gas atomization and PREP, embodies the expertise required for such critical applications. Their focus on enabling next-generation manufacturing positions them as a valuable partner for aerospace companies seeking to push the boundaries of satellite performance.
The journey to implementing AM for critical flight hardware involves careful consideration of design, materials, tolerances, costs, and supplier capabilities. By embracing metal AM and partnering with knowledgeable experts, the aerospace industry can continue to develop more capable, reliable, and cost-effective satellites, truly elevating performance in the final frontier.
Ready to explore how metal additive manufacturing can revolutionize your satellite thermal management systems? Contact Met3dp today to discuss your specific requirements and learn how our comprehensive solutions can power your organization’s additive manufacturing goals.
Teilen auf
Facebook
Twitter
LinkedIn
WhatsApp
E-Mail
MET3DP Technology Co., LTD ist ein führender Anbieter von additiven Fertigungslösungen mit Hauptsitz in Qingdao, China. Unser Unternehmen ist spezialisiert auf 3D-Druckgeräte und Hochleistungsmetallpulver für industrielle Anwendungen.
Fragen Sie an, um den besten Preis und eine maßgeschneiderte Lösung für Ihr Unternehmen zu erhalten!
Verwandte Artikel
Über Met3DP
Aktuelles Update
Unser Produkt
KONTAKT US
Haben Sie Fragen? Senden Sie uns jetzt eine Nachricht! Wir werden Ihre Anfrage mit einem ganzen Team nach Erhalt Ihrer Nachricht bearbeiten.
Holen Sie sich Metal3DP's
Produkt-Broschüre
Erhalten Sie die neuesten Produkte und Preislisten
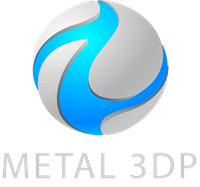
Metallpulver für 3D-Druck und additive Fertigung
UNTERNEHMEN
PRODUKT
cONTACT INFO
- Qingdao Stadt, Shandong, China
- [email protected]
- [email protected]
- +86 19116340731