Valve Housings for Cryogenic Rocket Systems via AM
目次
Introduction: The Critical Role of Valve Housings in Cryogenic Rocket Propulsion
The relentless pursuit of space exploration and satellite deployment hinges on the reliability and performance of launch vehicles. At the heart of these sophisticated machines lie intricate propulsion systems, responsible for generating the immense thrust required to overcome Earth’s gravity. Within these systems, managing the flow of volatile cryogenic propellants – primarily liquid oxygen (LOX) and liquid hydrogen (LH2), stored at temperatures hundreds of degrees below freezing – is paramount. This critical task falls upon a range of components, among which the valve housing stands out as a linchpin of operational integrity.
Rocket valve housings are far more than simple enclosures. They are sophisticated, pressure-bearing structures designed to contain and direct the flow of cryogenic fluids under extreme pressure differentials and thermal gradients. They form the body of various valve types – check valves, control valves, shut-off valves, relief valves – each essential for precise propellant management during different phases of flight, from engine start-up and thrust vector control to stage separation and mission termination. The failure of even a single valve housing can have catastrophic consequences, leading to mission failure or worse.
Traditionally, manufacturing these critical components has relied heavily on subtractive methods like CNC machining from billet or investment casting, often followed by extensive joining processes like welding. While proven, these methods face significant challenges when applied to the demanding requirements of modern rocketry:
- Geometric Complexity: Optimal fluid dynamics often require intricate internal passages and external mounting features, pushing the limits of machining capabilities and driving up costs. Complex geometries may necessitate manufacturing multiple individual pieces that are later welded or bolted together, introducing potential leak paths and points of failure.
- 廃棄物: Subtractive manufacturing inherently involves carving the final shape from a larger block of material, leading to substantial waste, especially when dealing with expensive aerospace-grade superalloys.
- Long Lead Times: The multi-step process involving tooling creation (for casting), complex machining setups, and assembly can result in lead times stretching over months, hindering rapid development cycles crucial in the competitive space industry.
- 重量の制約: Every kilogram launched into orbit carries a significant cost penalty. Traditional methods often result in over-engineered, heavier components than necessary, as optimizing for weight reduction through machining complex internal structures is difficult and costly.
- Supply Chain Bottlenecks: Reliance on specialized foundries or machine shops can create bottlenecks, particularly for niche alloys or complex low-volume parts.
入力 アディティブ・マニュファクチャリング(AM), more commonly known as 3Dプリンティング. Specifically, metal AM techniques like Laser Powder Bed Fusion (LPBF) are emerging as a disruptive force, offering a transformative approach to producing high-performance aerospace components like rocket valve housings. AM builds parts layer by layer directly from a digital model using fine metal powder, enabling the creation of geometries previously unattainable through conventional means. This technology allows engineers to rethink component design, focusing on function and performance rather than being constrained by manufacturability limitations. For cryogenic valve housings, AM offers a compelling pathway to enhanced performance, reduced weight, faster development cycles, and improved supply chain resilience – critical advantages in the demanding world of aerospace engineering. As aerospace manufacturers and procurement specialists seek more efficient, high-performance solutions, understanding the potential of AM for producing these vital components is no longer optional, but essential.
Applications and Demands: Where are AM Rocket Valve Housings Used?
The adoption of Additive Manufacturing for rocket valve housings isn’t a futuristic concept; it’s a rapidly growing reality driven by tangible performance and production benefits. These components are finding their place in the most demanding sections of modern launch vehicles and spacecraft, where precision, reliability, and weight are non-negotiable. Understanding their specific applications highlights the demanding operational environment they endure and why AM is uniquely suited to meet these challenges.
Key Application Areas:
- Main Engine Propulsion Systems: This is arguably the most critical application. Valve housings here control the massive flow rates of LOX and LH2 (or other propellants like RP-1 and methane) into the main combustion chamber.
- Functions: Main propellant shut-off valves (ensuring safe engine start and shutdown), throttle valves (controlling thrust levels), pre-valves (controlling flow into turbopumps), and check valves (preventing backflow).
- Demands: Must withstand extreme pressures (often hundreds of bars), cryogenic temperatures (-183°C for LOX, -253°C for LH2), high vibrations during engine firing, and require absolute leak-tight integrity. AM enables complex internal flow paths designed for optimal fluid dynamics and minimal pressure drop, alongside integrated sensor ports or mounting features, reducing part count and potential leak points.
- Upper Stage Engines: These engines operate in the vacuum of space and are often required to perform multiple restarts. Their valve systems manage smaller propellant quantities but require high precision for orbital maneuvering and payload deployment.
- Functions: Propellant isolation valves, mixture ratio control valves, ullage pressure control valves.
- Demands: High reliability over potentially long mission durations, precise flow control, minimal weight, ability to withstand thermal cycling (heating during firing, cooling during coast phases). AM’s lightweighting potential is particularly valuable here, as mass saved in the upper stage directly translates to increased payload capacity.
- Reaction Control Systems (RCS) / Attitude Control Systems (ACS): These systems use small thrusters to control the orientation (attitude) of the launch vehicle or spacecraft. Their valves handle smaller flows of hypergolic propellants or cold gas.
- Functions: Thruster isolation valves, pressure regulators.
- Demands: Fast response times, high cycle life (requiring excellent fatigue resistance), compatibility with potentially corrosive propellants, extreme miniaturization potential. AM allows for highly integrated manifolds and valve blocks, combining multiple valve functions into a single, compact, lightweight printed component.
- Satellite Propulsion Systems: Similar to upper stages and RCS, satellite propulsion demands miniaturization, reliability, and long-term performance for station-keeping and orbital adjustments.
- Functions: Latch valves, regulators, thruster control valves.
- Demands: Very low leak rates, long operational life (often 15+ years), radiation resistance, minimal mass. AM facilitates the creation of bespoke valve designs optimized for specific satellite platforms and mission profiles.
Meeting Stringent Industry Demands:
The aerospace industry, encompassing commercial space launch providers (like SpaceX, Blue Origin, Rocket Lab), defense contractors, and government agencies (like NASA, ESA), imposes incredibly high standards. AM valve housings must meet or exceed the performance of their traditionally manufactured counterparts.
- Reliability & Safety: Non-negotiable. Extensive testing, qualification, and process control are required to ensure parts perform flawlessly under extreme conditions. Material properties must be consistent and predictable.
- パフォーマンス Achieving precise flow rates, minimizing pressure drops, ensuring tight sealing, and meeting specific actuation requirements are crucial. AM allows designers to optimize internal geometries for fluid dynamics in ways previously impossible.
- 軽量化: A primary driver for AM adoption. Reducing component mass, particularly in upper stages and satellites, yields significant cost savings and performance gains (e.g., higher payload capacity, delta-v capability). AM enables topology optimization and the use of internal lattice structures to shed weight without compromising structural integrity.
- 費用対効果: While AM machine time and specialized powders can be expensive, the total cost of ownership can be lower due to reduced material waste, eliminated tooling costs (especially for low-volume production), consolidated parts (reducing assembly labor and time), and significantly shorter lead times enabling faster iteration and quicker time-to-market. Companies seeking an Aerospace Valve Supplier または AM Production Partner are increasingly turning to additive methods for these benefits.
The demanding nature of these applications underscores why advanced metal AM techniques, coupled with high-performance materials like IN625 and IN718, are becoming indispensable tools for aerospace engineers and procurement managers responsible for sourcing Rocket Engine Parts and critical Cryogenic Valve Applications.
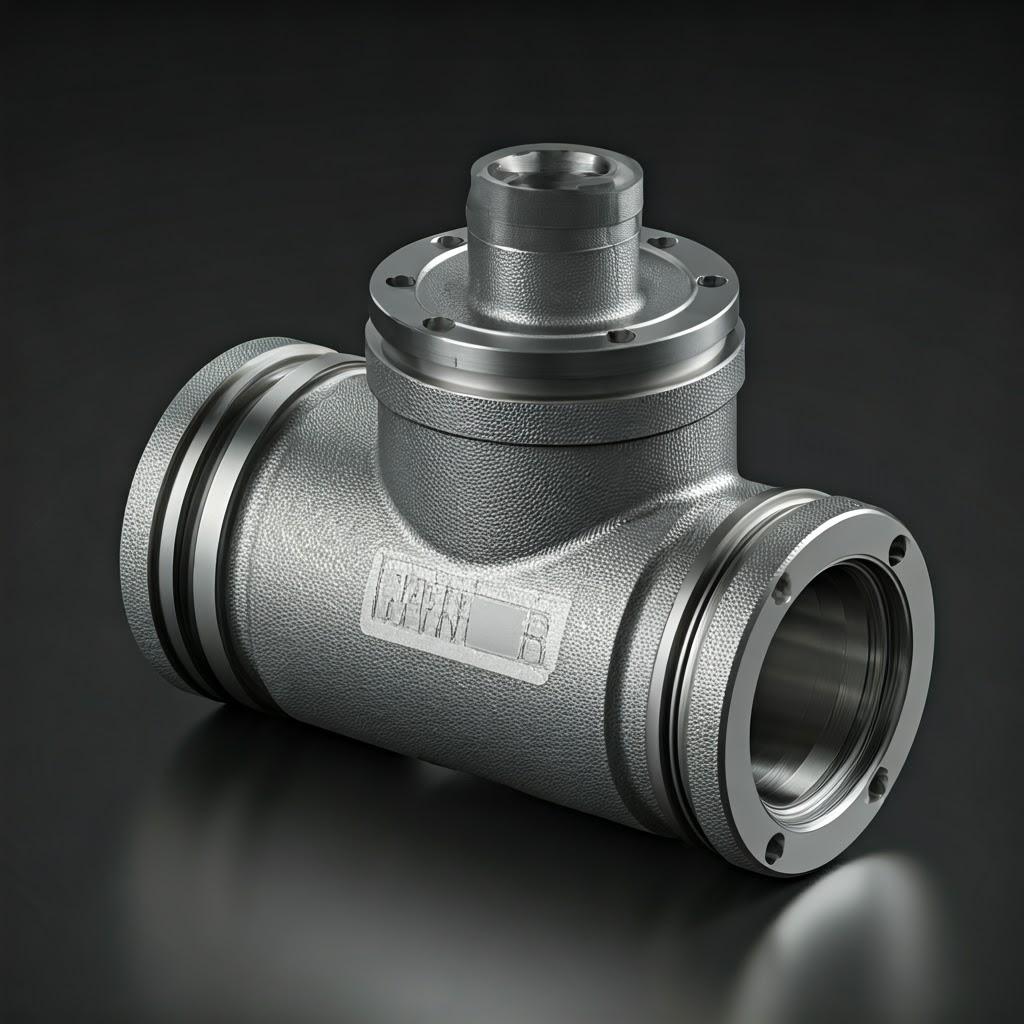
Why Metal Additive Manufacturing for Rocket Valve Housings? Unlocking Performance Benefits
The decision to transition from established manufacturing processes like casting or machining to metal Additive Manufacturing for critical components like rocket valve housings is driven by a compelling set of advantages. While traditional methods have served the industry well, AM offers unique capabilities that directly address the core challenges of aerospace design and production: complexity, weight, speed, and cost-efficiency, particularly for demanding cryogenic applications. Let’s compare AM, specifically Laser Powder Bed Fusion (LPBF), with conventional methods and delve into the specific benefits unlocked.
AM vs. Traditional Manufacturing for Valve Housings:
特徴 | CNC Machining (Subtractive) | インベストメント鋳造 | 鍛造 | Metal AM (LPBF) (Additive) |
---|---|---|---|---|
複雑さ | Limited by tool access, internal features hard | Good for complex shapes, requires tooling | Simpler shapes, high strength | High geometric freedom, complex internal channels feasible |
部品の統合 | Difficult, often requires assembly | Possible, but tooling complexity rises | 限定 | Excellent, multiple parts can be integrated into one print |
軽量化 | Limited by subtractive nature | Some potential, requires tooling mods | 限定 | High potential via topology optimization, lattices |
廃棄物 | High (material removed) | Moderate (gates, runners) | 低い | Low (powder recycled), supports generate some waste |
リードタイム | Moderate (programming, setup) to Long (complex) | Long (tooling design & fabrication) | Long (die design & fabrication) | Short (prototypes) to Moderate (production), no hard tooling |
金型費用 | Low (fixtures) to Moderate (custom tools) | High (wax patterns, ceramic shells) | Very High (dies) | None (digital manufacturing) |
素材オプション | Wide range of machinable alloys | Castable alloys | Forgeable alloys | Growing range of weldable alloys (IN625, IN718 suitable) |
Ideal Volume | Low to High | 中~高 | 高い | Low (prototypes, custom) to Medium (series production) |
Export to Sheets
Key Advantages of Metal AM for Rocket Valve Housings:
- Unprecedented Geometric Freedom & Part Consolidation:
- チャレンジ: Traditional methods struggle with intricate internal flow paths needed for optimal fluid dynamics or compact designs. Often, multiple machined or cast pieces must be welded or brazed together, introducing potential leak paths, stress concentrations, and additional inspection points.
- AM Solution: LPBF builds the part layer by layer, allowing for the creation of highly complex internal channels, conformal cooling passages, and organically shaped structures optimized for flow and stress distribution. Crucially, multiple components (e.g., housing body, flanges, brackets, internal baffles) can often be consolidated into a single printed part.
- Benefit: Reduced part count simplifies assembly, lowers weight, eliminates potential leak paths associated with joints, improves structural integrity, and reduces the overall Bill of Materials (BOM) and associated logistical overhead. This capability is a game-changer for designing high-performance, reliable Complex Aerospace Parts.
- Significant Lightweighting Potential:
- チャレンジ: Minimizing mass is critical in aerospace. Traditional methods make it difficult to remove material selectively from low-stress areas without compromising strength or significantly increasing machining time.
- AM Solution: AM enables advanced lightweighting strategies:
- Topology Optimization: Software algorithms analyze stress distributions and remove material from non-critical areas, creating highly efficient, organic-looking structures that maintain strength only where needed.
- 格子構造: Internal voids can be filled with engineered lattice structures, providing support and rigidity at a fraction of the weight of solid material.
- Benefit: Drastic weight reductions (often 20-50% or more) compared to traditionally manufactured counterparts, directly improving payload capacity and mission performance. This makes AM ideal for producing Lightweight Rocket Components.
- Accelerated Prototyping, Iteration, and Production:
- チャレンジ: Designing, tooling, manufacturing, and testing a prototype using traditional methods can take weeks or months. Design iterations are slow and costly due to tooling modifications or new machining programs.
- AM Solution: AM operates directly from a digital CAD file, eliminating the need for physical tooling. Prototypes can often be printed in days. Design modifications involve simply changing the CAD model and initiating a new print.
- Benefit: 有効にする Aerospace Rapid Prototyping, allowing engineers to test multiple design variations quickly and affordably. This drastically shortens the development cycle, fosters innovation, and allows design flaws to be caught earlier. Furthermore, for production parts, AM can significantly reduce lead times compared to casting or forging, offering greater supply chain agility – a key factor for businesses seeking a responsive AM Production Partner. Explore the different 印刷方法 available to understand the possibilities.
- Reduced Material Waste and Buy-to-Fly Ratio:
- チャレンジ: Machining high-value superalloys like Inconel generates significant scrap material (chips and shavings), leading to a poor “buy-to-fly” ratio (the ratio of the weight of the raw material purchased to the weight of the final part).
- AM Solution: LPBF is an additive process, using only the material needed for the part and its support structures. Unfused powder within the build chamber can typically be sieved and recycled for subsequent builds.
- Benefit: Dramatically reduces material waste, making the process more sustainable and cost-effective, especially for expensive aerospace materials. Improves the buy-to-fly ratio significantly.
- On-Demand Manufacturing and Supply Chain Flexibility:
- チャレンジ: Traditional manufacturing often relies on large batch production runs to amortize high tooling costs. Maintaining large inventories ties up capital, and sourcing specialized components can involve long waits and vulnerable supply chains.
- AM Solution: AM enables economically viable production of small batches or even single custom parts without tooling costs. Parts can be produced “on-demand” closer to the point of need.
- Benefit: Reduces inventory requirements, enables the creation of highly customized valve housings for specific mission profiles, and provides a pathway for digital inventory and distributed manufacturing, enhancing supply chain resilience against disruptions.
By leveraging these benefits, metal additive manufacturing provides aerospace engineers and procurement managers with a powerful tool to overcome the limitations of traditional processes, enabling the development and production of next-generation cryogenic rocket valve housings that are lighter, more complex, potentially cheaper in the long run, and available faster than ever before.
Material Focus: IN625 and IN718 for Cryogenic Extremes
The extreme operating environment of a cryogenic rocket valve housing – encompassing temperatures from ambient down to -253°C, high pressures, thermal cycling, and the need for absolute reliability – dictates the use of highly specialized materials. Among the frontrunners for these demanding applications, particularly when using additive manufacturing, are the nickel-based superalloys Inconel 625 (IN625) and Inconel 718 (IN718). These materials possess an exceptional combination of properties that make them ideally suited for the challenges of space propulsion, and importantly, they are well-characterized and readily processable using Laser Powder Bed Fusion (LPBF) techniques.
Understanding Nickel-Based Superalloys:
Nickel-based superalloys are a class of metallic materials engineered to operate under severe conditions of high temperature, high stress, and corrosive or oxidizing environments. Their exceptional performance stems from their face-centered cubic (FCC) austenite matrix, strengthened by various alloying elements that form stable precipitates (like gamma prime and gamma double-prime phases in IN718) or provide solid-solution strengthening (like Molybdenum and Niobium in IN625). While often associated with high-temperature applications (jet engine turbines, combustion chambers), many nickel superalloys also exhibit outstanding toughness and maintain strength at cryogenic temperatures, making them versatile for aerospace extremes.
Inconel 625 (IN625 / UNS N06625 / Alloy 625): The Corrosion-Resistant Workhorse
IN625 is a non-magnetic, nickel-chromium-molybdenum-niobium alloy known for its superb fabricability and outstanding corrosion resistance across a wide range of aggressive environments.
- Key Properties & Benefits for Cryogenic Valves:
- Excellent Cryogenic Toughness: Unlike many steels that become brittle at low temperatures, IN625 retains excellent ductility and toughness down to cryogenic levels (-196°C and below), preventing brittle fracture under stress.
- 高い強度: While not as strong as precipitation-hardened alloys like IN718 at room temperature or elevated temperatures, IN625 offers good tensile and fatigue strength across a broad temperature range, including cryogenic conditions. Its strength primarily comes from the solid-solution strengthening effects of Molybdenum and Niobium within the nickel-chromium matrix.
- Superb Corrosion Resistance: Highly resistant to pitting, crevice corrosion, and general corrosion in various media, including oxidizing and reducing acids, and chloride stress-corrosion cracking. This is beneficial if trace contaminants or different fluid types might be encountered.
- Excellent Weldability & Fabricability: IN625 is known for being relatively easy to weld and process using AM techniques like LPBF. It has lower susceptibility to post-weld cracking compared to some other superalloys. This translates to good printability with lower risk of cracking during the layer-by-layer fusion process in AM.
- Thermal Fatigue Resistance: Handles thermal cycling between ambient and cryogenic temperatures well.
- AM Considerations: IN625 generally prints well with LPBF, achieving high densities (>99.5%). Standard stress relief heat treatments are typically required after printing to minimize residual stresses built up during the rapid heating and cooling cycles. Solution annealing can further enhance properties.
Inconel 718 (IN718 / UNS N07718 / Alloy 718): The High-Strength Standard
IN718 is arguably the most widely used nickel-based superalloy, particularly in aerospace. It’s a precipitation-hardenable alloy, meaning its exceptional strength is derived from heat treatments that cause the formation of finely dispersed strengthening phases (gamma prime and gamma double-prime) within the material matrix.
- Key Properties & Benefits for Cryogenic Valves:
- 並外れた強さ: Offers significantly higher tensile, yield, creep, and rupture strength compared to IN625, particularly at temperatures up to around 700°C (1300°F). This high strength is largely maintained down to cryogenic temperatures. This allows for thinner walls and lighter designs while still meeting pressure requirements.
- Good Cryogenic Performance: While perhaps slightly less ductile than IN625 at the lowest cryogenic temperatures, IN718 still exhibits good toughness and is widely used in cryogenic applications requiring high strength-to-weight ratios.
- Excellent Fatigue Resistance: Critical for components subjected to vibrational loads and pressure cycles common in rocket engines.
- Good Weldability (for a precipitation-hardenable alloy): Its sluggish precipitation kinetics make it more weldable (and thus, more printable via AM) than many other precipitation-strengthened superalloys, reducing susceptibility to cracking during the build or post-heat treatment.
- 良好な耐食性: While generally not considered as corrosion-resistant as IN625 in highly aggressive environments, IN718 offers adequate resistance for typical aerospace propellant applications.
- AM Considerations: IN718 is one of the most common and well-understood superalloys processed by LPBF. Achieving optimal properties requires careful control over printing parameters and, crucially, specific post-processing heat treatments. These typically involve stress relief, followed by solution annealing and a two-step aging process (precipitation hardening) to develop the strengthening phases and achieve the desired high strength and hardness. Hot Isostatic Pressing (HIP) is often recommended for critical IN718 components to close any residual internal porosity and further enhance fatigue properties.
Why These Materials Matter for AM Valve Housings:
The choice between IN625 and IN718 often depends on the specific requirements of the valve housing:
- If maximum strength and fatigue life are the primary drivers, allowing for the lightest possible design, IN718 is often preferred, provided the necessary multi-step heat treatments are performed correctly.
- If maximum corrosion resistance or slightly better ductility/toughness at the lowest cryogenic temperatures are paramount, or if simpler post-processing is desired, IN625 の方がいいかもしれない。
- Both materials offer the 高強度重量比 essential for aerospace.
- Crucially, both have demonstrated good processability via LPBF, enabling the complex geometries and consolidated designs that make AM so attractive for these components.
The Role of High-Quality Metal Powders:
The success of manufacturing IN625 and IN718 valve housings via AM is fundamentally dependent on the quality of the input material – the metal powder. Inferior powder can lead to defects in the final part, compromising its integrity. Key powder characteristics include:
- 真球度: Highly spherical powder particles ensure good flowability within the AM machine’s recoating system, leading to uniform powder layers and consistent melting.
- 粒度分布(PSD): A controlled PSD is crucial for achieving high packing density in the powder bed, which contributes to producing fully dense final parts with minimal porosity.
- 化学組成: Must strictly adhere to aerospace specifications for the respective alloy (e.g., AMS standards). Impurities can degrade mechanical properties.
- Low Oxygen/Nitrogen Content: Excessive interstitial gas content can lead to porosity and embrittlement.
- Absence of Satellites: Small, irregular particles attached to larger spherical ones (satellites) can hinder flowability and packing density.
This is where specialized powder manufacturers play a critical role. Companies like メット3dp, utilizing advanced production techniques such as Vacuum Induction Melting Gas Atomization (VIGA) そして プラズマ回転電極プロセス(PREP), focus on producing 高性能金属粉末 with optimized sphericity, controlled PSD, high purity, and excellent flowability specifically tailored for demanding AM applications. Met3dp’s expertise in powder metallurgy ensures that the IN625 and IN718 powders used possess the ideal characteristics required to reliably print dense, high-quality, mission-critical cryogenic valve housings meeting the stringent demands of the aerospace industry. Their commitment extends beyond powders, offering insights into the entire 金属3Dプリンティング ecosystem. Partnering with a supplier that understands both material science and the nuances of the AM process is vital for aerospace manufacturers and procurement specialists sourcing these critical components. Sources and related content
Design for Additive Manufacturing (DfAM): Optimizing Valve Housings for Printing
Simply taking a design intended for traditional manufacturing and sending it to a metal 3D printer rarely yields optimal results, especially for demanding components like cryogenic rocket valve housings. To fully harness the benefits of Additive Manufacturing – lightweighting, part consolidation, enhanced performance – engineers must embrace 積層造形設計 (DfAM) principles. DfAM is not just about ensuring a part できる be printed; it’s about actively designing the part to leverage AM’s unique capabilities while mitigating its constraints. For valve housings, this means considering factors like build orientation, support structures, internal channel design, and feature resolution from the outset.
Key DfAM Principles for AM Valve Housings:
- Build Orientation Strategy:
- What it is: Deciding how the valve housing will be oriented on the build plate within the AM machine.
- Why it matters: Orientation significantly impacts:
- サポート体制: Areas overhanging beyond a certain angle (typically 45 degrees from the horizontal, though material and parameter dependent) require support structures to prevent collapse during the build. Orientation determines where supports are needed, how extensive they are, and how easy they are to remove later. Minimizing supports, especially on critical internal or external surfaces, is key.
- 表面仕上げ: Downward-facing (underside) surfaces and surfaces directly attached to supports tend to have poorer surface finish than upward-facing or vertical walls. Critical sealing surfaces or internal flow paths should ideally be oriented to minimize contact with supports or be self-supporting.
- 機械的特性: Due to the layer-by-layer nature, AM parts can exhibit slight anisotropy (directional differences in properties). Orientation can influence strength and fatigue life relative to the direction of primary loading.
- Build Time & Cost: Taller builds generally take longer. Orienting the part to minimize its height (Z-axis) in the build chamber can reduce print time.
- Optimization: Engineers must strategically orient the valve housing to balance these factors, often using simulation software to predict support needs and potential distortion.
- Support Structure Optimization:
- What it is: Designing the temporary structures that anchor the part to the build plate and support overhanging features during printing.
- Why it matters: Poorly designed supports can be difficult and time-consuming to remove (increasing post-processing costs), potentially damage the part surface during removal, and consume valuable material and print time. Conversely, insufficient supports can lead to build failure or part distortion.
- Optimization:
- Minimize Need: Design features to be “self-supporting” wherever possible by using angles less than the critical overhang limit (e.g., chamfering sharp overhangs instead of using 90-degree angles). Teardrop shapes for horizontal holes can often eliminate the need for internal supports.
- アクセシビリティ: Ensure support structures are placed in locations accessible for removal tools (manual or potentially wire EDM). Avoid designing supports deep within complex internal cavities where they cannot be reached.
- Contact Point Minimization: Use support types (e.g., conical or thin-walled supports) that minimize the contact area with the actual part surface, making removal easier and reducing surface blemishes (“witness marks”).
- Heat Dissipation: Supports also help conduct heat away from overhangs. Their design must consider thermal management during the build.
- Wall Thickness & Feature Resolution:
- What it is: Considering the minimum and maximum printable wall thicknesses and the smallest positive or negative features (like holes or pins) the specific AM process and material can reliably produce.
- Why it matters: Walls that are too thin may not print reliably, can warp, or lack structural integrity. Very thick sections can accumulate excessive heat, potentially leading to distortion or undesirable microstructures. Small features might lose definition due to the laser spot size and melt pool dynamics.
- Optimization: Adhere to machine/material-specific guidelines (e.g., minimum wall thickness often around 0.4-0.8 mm for LPBF). Ensure critical features like sealing grooves, sensor ports, or small orifices are designed within the achievable resolution limits. Use topology optimization tools carefully to avoid creating features too thin to print reliably. Maintain relatively uniform wall thicknesses where possible to promote even heating and cooling.
- Internal Channels & Passages:
- What it is: Designing the complex internal pathways crucial for fluid flow within the valve housing.
- Why it matters: AM excels at creating complex internal geometries, but trapped powder and support removal are major concerns. Internal channels must be designed to be self-supporting (avoiding flat horizontal ceilings) or allow for powder removal after printing.
- Optimization:
- Self-Supporting Shapes: Design internal channels with teardrop, diamond, or circular cross-sections where possible, ensuring overhang angles are below the critical limit. Avoid large, flat internal ceilings that would require extensive internal supports.
- Powder Removal Access: Ensure there are adequate inlet/outlet ports or strategically placed access holes (which might be plugged later) to allow unfused powder to be completely removed from internal cavities after the build using vibration, compressed air, or flushing. Trapped powder is a critical defect.
- 表面粗さ: Be aware that the as-built surface finish of internal channels will be rougher than machined surfaces, potentially impacting flow characteristics. Design for manufacturability considering this roughness, or incorporate post-processing steps like abrasive flow machining or electropolishing if a smoother internal finish is required.
- Part Consolidation Opportunities:
- What it is: Actively looking for opportunities to combine multiple components from a traditional assembly into a single, monolithic printed part.
- Why it matters: This is one of AM’s most significant advantages. Consolidating parts like the main valve body, mounting flanges, brackets, or even internal flow-directing features reduces assembly time and labor, eliminates potential leak paths from joints/welds/brazes, lowers overall weight, and simplifies the supply chain.
- Optimization: Re-evaluate the entire valve assembly. Can brackets be integrated directly into the housing? Can multiple flow paths be combined within a single printed manifold? Can sensor mounts be incorporated? DfAM encourages a holistic view of the component’s function within the larger system.
Leveraging Software and Expertise:
Successfully implementing DfAM for complex parts like cryogenic valve housings often requires sophisticated software tools for:
- Topology Optimization: Automatically generating lightweight, load-bearing structures.
- Lattice Generation: Creating weight-saving internal lattice structures.
- Build Simulation: Predicting thermal stresses, distortion, and optimal support strategies before printing.
- CAD for AM: Design tools specifically incorporating AM constraints and capabilities.
Furthermore, close collaboration with an experienced Metal AM Service Provider is invaluable. Providers like メット3dp possess deep expertise not only in operating LPBF machines but also in the nuances of DfAM for materials like IN625 and IN718. Their engineers can provide crucial feedback on design manufacturability, suggest optimizations for cost and performance, and help navigate the complexities of support strategy and orientation. Understanding the provider’s capabilities and leveraging their experience early in the design phase is key to maximizing the benefits of AM for Valve Design Optimization.
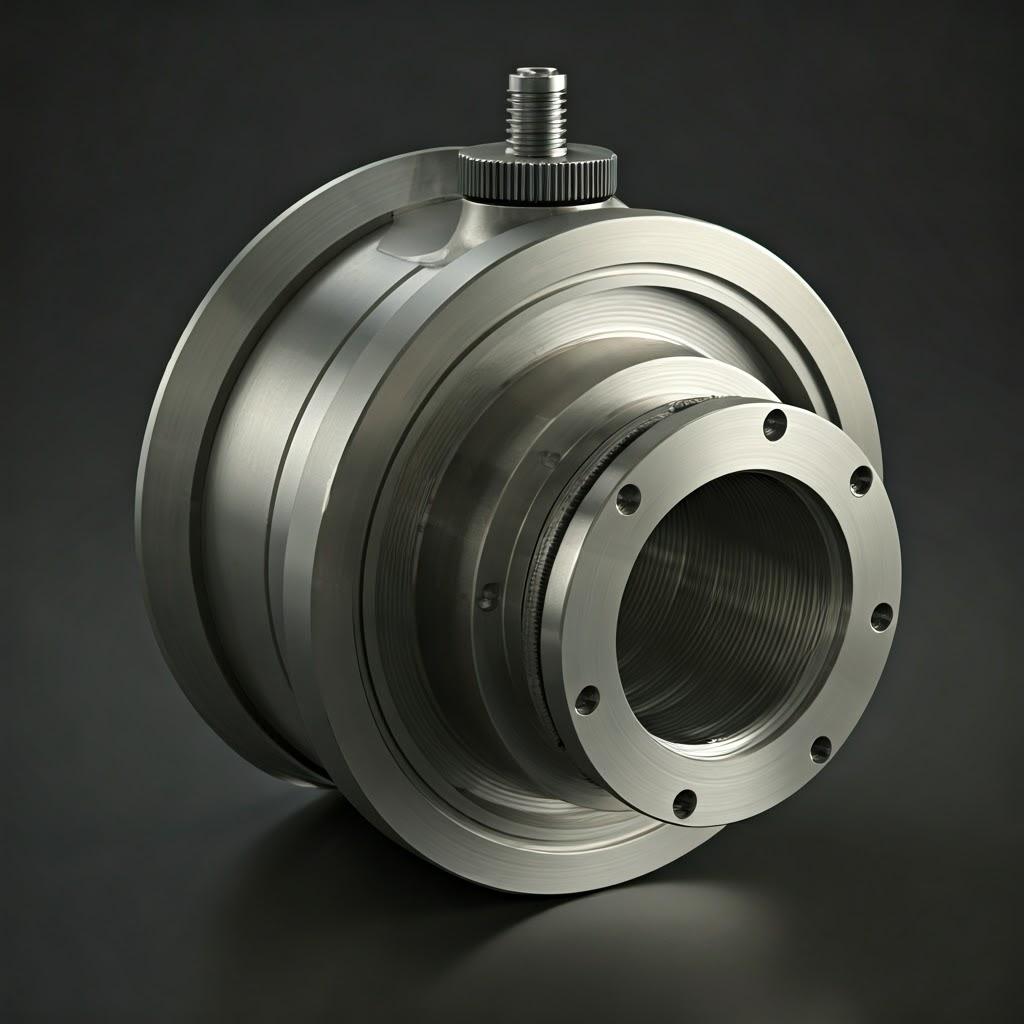
Achievable Precision: Tolerance, Surface Finish, and Dimensional Accuracy in AM Valve Housings
While Additive Manufacturing offers unparalleled geometric freedom, it’s crucial for engineers and procurement managers to understand the levels of precision achievable directly from the printing process and what post-processing steps might be necessary to meet the stringent requirements of cryogenic rocket valve housings. Factors like dimensional tolerance, surface finish (roughness), and overall accuracy are critical for ensuring proper sealing, assembly fit, and functional performance.
Typical Tolerances with Laser Powder Bed Fusion (LPBF):
LPBF is one of the most precise metal AM processes, but it’s not inherently as precise as high-end CNC machining across all dimensions straight off the build plate. Typical achievable tolerances for well-controlled LPBF processes using materials like IN625 and IN718 are often in the range of:
- General Dimensional Tolerance: ±0.1 mm to ±0.2 mm for smaller features (e.g., up to 50-100 mm).
- Larger Dimensions: Tolerances might scale with size, often cited as ±0.1% to ±0.2% of the nominal dimension for larger parts.
- Feature-Specific Tolerances: Hole diameters, wall thicknesses, and positional accuracy of features can vary depending on orientation, location within the build, and thermal effects.
Factors Influencing Tolerance:
- Machine Calibration: Accuracy of the laser scanning system, layer thickness control, and thermal management within the machine.
- 素材の特性: Thermal expansion and contraction characteristics of IN625/IN718.
- Part Geometry & Orientation: Complex shapes and orientations can lead to varying thermal gradients and potential distortion.
- Thermal Stresses: Residual stresses built up during printing can cause warping if not properly managed through support strategies and stress relief heat treatments.
- 後処理: Stress relief and HIP can cause minor, predictable dimensional changes.
As-Built Surface Finish (Roughness):
The surface finish of parts directly printed via LPBF is inherently rougher than machined surfaces. The roughness depends heavily on the orientation of the surface relative to the build direction and powder characteristics.
- Upward-Facing Surfaces & Vertical Walls: Typically exhibit the best finish, often in the range of Ra 6 µm to 15 µm (Roughness Average).
- Downward-Facing Surfaces (Overhangs): Tend to be rougher due to the interaction with support structures or the partially melted powder beneath, often Ra 15 µm to 25 µm またはそれ以上。
- Side-Facing Surfaces: Angled surfaces will have a “stair-stepping” effect due to the layers, influencing roughness.
Factors Influencing Surface Finish:
- 層の厚さ: Thinner layers generally result in smoother surfaces but increase build time.
- 粉末の粒度分布: Finer powders can lead to smoother finishes.
- Laser Parameters: Melting parameters (power, speed, hatch spacing) influence the melt pool characteristics and resulting surface texture.
- Orientation: As mentioned, surface angle relative to the build direction is a primary factor.
- Gas Flow: Shielding gas flow within the build chamber can affect melt pool stability and spatter, influencing surface quality.
Achieving Tighter Tolerances and Smoother Finishes:
For critical features on a cryogenic valve housing – such as sealing faces, mounting interfaces, thread locations, or precise internal diameters – the as-built tolerances and surface finish from LPBF are often insufficient. Post-machining is typically required.
- CNC Machining: Critical dimensions, sealing grooves (e.g., for O-rings or metallic seals), flange faces, and threaded holes are commonly finish-machined after printing and heat treatment. This allows achieving tolerances typical of conventional machining (e.g., ±0.01 mm to ±0.05 mm or tighter) and specific surface finish requirements (e.g., Ra < 1.6 µm or even lower for sealing surfaces).
- Other Finishing Processes: Depending on requirements, processes like grinding, polishing, electropolishing, or abrasive flow machining (for internal channels) can be used to achieve very smooth surfaces (Ra < 0.8 µm or better).
Dimensional Inspection and Ensuring Leak-Tight Performance:
Rigorous inspection is mandatory for aerospace components.
- Coordinate Measuring Machines (CMM): Used to verify critical dimensions against the CAD model and engineering drawings after printing and post-machining.
- 3D Scanning: Non-contact optical or laser scanners can capture the full geometry of the part for comparison against the nominal design, useful for complex shapes and verifying overall form.
- Leak Testing: Absolutely essential for valve housings. This involves pressurizing the component (often using helium in a vacuum chamber for high sensitivity) to ensure there are no leaks through the housing walls or critical sealing interfaces, meeting stringent aerospace standards. Leak-Tight Components are a primary requirement.
Implications for Design and Procurement:
- Design for Post-Machining: Engineers must design the AM part with sufficient extra material (machining allowance or “stock”) on surfaces that will require finish machining.
- Specify Requirements Clearly: Engineering drawings must clearly define final dimensional tolerances, surface finish requirements (using appropriate symbols like Ra), and inspection criteria for both as-built AM features and post-machined features.
- Supplier Capability: Procurement managers need to ensure their chosen Metal AM Service Provider has the capability not only to print the part accurately but also to manage or perform the necessary post-machining and inspection processes to meet final specifications, or has certified partners to do so.
Understanding the interplay between Metal 3D Printing Tolerances, AM Surface Finish, and post-processing is crucial for successfully manufacturing functional and reliable cryogenic rocket valve housings that meet the demanding Dimensional Accuracy Aerospace の基準を満たす。
Beyond Printing: Essential Post-Processing Steps for Rocket Valve Housings
Producing a dimensionally accurate metal shape layer by layer is only the first step in manufacturing a functional, flight-ready cryogenic rocket valve housing using Additive Manufacturing. The “as-built” part, fresh off the printer, requires a series of crucial AM Post-Processing steps to relieve internal stresses, remove temporary structures, achieve the desired material properties, ensure internal soundness, and meet final dimensional and surface finish requirements. Neglecting or improperly performing these steps can compromise the component’s integrity and performance.
For high-performance nickel superalloys like IN625 and IN718 used in critical aerospace applications, post-processing is not optional; it’s an integral part of the manufacturing workflow.
Common and Essential Post-Processing Steps:
- Stress Relief Heat Treatment:
- Why: The rapid heating and cooling cycles inherent in LPBF create significant residual stresses within the printed part. These stresses can cause warping (especially after removal from the build plate), distortion during subsequent machining, and can negatively impact mechanical properties, particularly fatigue life.
- プロセス Typically performed while the part is still attached to the build plate, often in a vacuum or inert atmosphere furnace. The part is heated to a specific temperature below the annealing or aging temperatures (e.g., ~870-980°C for IN718, ~870-900°C for IN625, but specific cycles depend on exact requirements and geometry), held for a period, and then slowly cooled. This allows the material’s microstructure to relax, significantly reducing internal stresses.
- Importance: Absolutely mandatory for IN625 and IN718 AM parts to ensure dimensional stability and prevent cracking.
- Part Removal from Build Plate:
- Why: The part is fused to a thick metal build plate during printing.
- プロセス Commonly done using wire Electrical Discharge Machining (Wire EDM) or a band saw. Wire EDM offers higher precision and a smoother cut surface.
- 支持構造の撤去:
- Why: Temporary supports used during printing must be removed.
- プロセス This can be challenging, especially for complex geometries or supports in internal areas. Methods include:
- 手動による除去: Using pliers, cutters, grinders, or specialized tools. Can be labor-intensive and requires skilled technicians to avoid damaging the part surface.
- 機械加工: Milling or turning can remove accessible supports.
- Wire EDM: Can sometimes be used for precise removal, especially near delicate features.
- Importance: Thorough removal is essential. Remaining support material can act as stress concentrators or interfere with function. Ease of Support Removal AM should be considered during the DfAM phase.
- Solution Annealing and Aging Heat Treatments (Especially for IN718):
- Why: To develop the desired microstructure and achieve the target mechanical properties (high strength, hardness, ductility). The as-built microstructure is often non-optimal.
- ソリューション・アニーリング: Dissolves precipitates formed during printing and homogenizes the microstructure. Involves heating to a high temperature (e.g., ~950-1050°C for IN718) followed by rapid cooling.
- Aging (Precipitation Hardening): A subsequent, lower-temperature, two-step heat treatment (e.g., ~720°C followed by ~620°C for IN718) causes the controlled precipitation of strengthening phases (gamma prime/double-prime). This step is critical for achieving IN718’s characteristic high strength.
- プロセス Performed in precisely controlled vacuum or inert atmosphere furnaces according to specific aerospace material specifications (e.g., AMS standards for Heat Treatment IN718).
- Importance: Essential for achieving the high-performance mechanical properties required for aerospace components. IN625 typically only requires solution annealing if maximum ductility or corrosion resistance is needed, as it’s primarily solid-solution strengthened.
- Why: To develop the desired microstructure and achieve the target mechanical properties (high strength, hardness, ductility). The as-built microstructure is often non-optimal.
- 熱間静水圧プレス(HIP):
- Why: Despite process optimization, LPBF parts can sometimes contain microscopic internal pores (due to trapped gas or incomplete fusion). These pores can act as crack initiation sites, significantly reducing fatigue life, which is unacceptable for critical components.
- プロセス The part is subjected to high temperature (below melting point, often near solution annealing temperature) and high isostatic pressure (typically 100-200 MPa) using an inert gas (like Argon) in a specialized HIP vessel. The combination of heat and pressure causes internal voids to collapse and diffusion bond, effectively eliminating porosity.
- Importance: Increasingly becoming standard practice for critical AM aerospace components, especially those made from IN718, to achieve maximum density (>99.9%), improve fatigue strength, ductility, and ensure material homogeneity. HIP Treatment Aerospace is a key quality assurance step.
- CNC Machining:
- Why: As discussed previously, to achieve tight tolerances on critical interfaces, sealing surfaces, threads, and precise bore diameters.
- プロセス Using mills, lathes, or multi-axis machining centers to selectively remove material from specific areas of the heat-treated and HIPed part. Requires careful fixture design to hold the often complex AM geometry.
- Importance: Bridges the gap between AM’s geometric freedom and the precision requirements of functional aerospace hardware. CNC Machining 3D Printed Parts requires expertise in handling near-net shape components.
- 表面仕上げ:
- Why: To improve surface smoothness beyond the as-built or machined state, potentially enhancing fluid flow, cleanability, or fatigue life.
- プロセス オプションには以下が含まれます:
- Manual Polishing/Grinding: For specific accessible areas.
- Mass Finishing: Tumbling or vibratory finishing with media (can be aggressive).
- Abrasive Flow Machining (AFM): Pumping abrasive media through internal channels to smooth them.
- Electropolishing: Electrochemical process to smooth surfaces, often used for improving corrosion resistance and cleanability.
- コーティング: Application of specialized coatings for thermal barrier, wear resistance, or other functions (less common for the housing body itself unless specific surface properties are needed).
- Importance: Applied based on specific functional requirements for certain surfaces of the valve housing.
- 非破壊検査(NDT):
- Why: To verify the internal and external integrity of the finished part without damaging it.
- プロセス Common NDT methods for AM aerospace parts include:
- Computed Tomography (CT) Scanning: Industrial X-ray technique to detect internal defects like porosity, inclusions, or cracks, and verify internal channel geometry.
- Fluorescent Penetrant Inspection (FPI): To detect surface-breaking cracks or defects.
- Ultrasonic Testing (UT): Can detect subsurface flaws.
- Radiographic Testing (X-ray): Traditional X-ray for internal defect detection.
- Importance: Mandatory quality assurance step to confirm the part meets stringent aerospace quality standards. NDT for AM Components is critical for flight certification.
The complexity and criticality of these post-processing steps underscore the need for a vertically integrated or well-managed supply chain. An ideal Aerospace Finishing Services partner, or an AM provider with strong in-house capabilities, is essential for reliably transforming a printed shape into a mission-ready cryogenic valve housing.
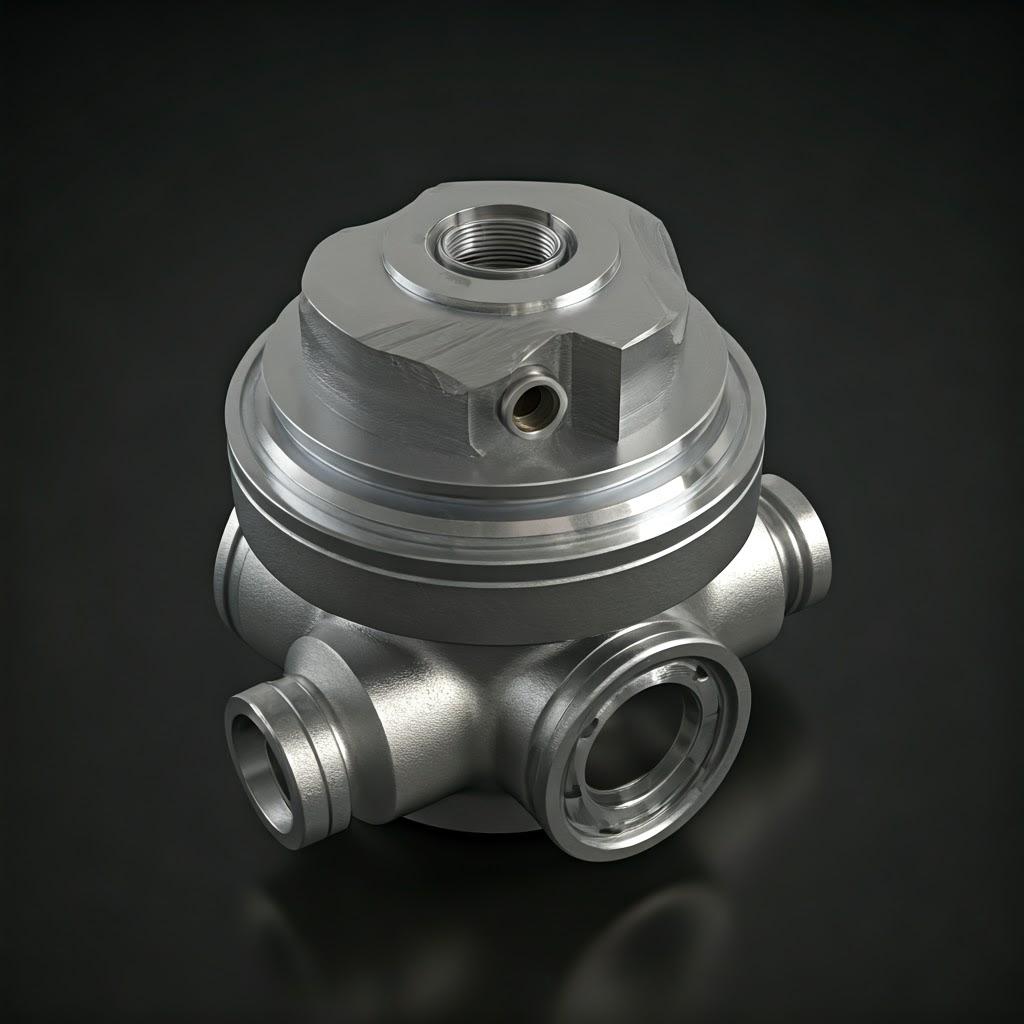
Navigating Challenges: Common Issues in AM Valve Housing Production and Solutions
While metal Additive Manufacturing offers significant advantages for producing complex components like cryogenic rocket valve housings, the process is not without its challenges. Understanding potential issues and implementing effective mitigation strategies are crucial for consistent, high-quality production. Aerospace engineers and procurement managers should be aware of these common hurdles when adopting AM.
Common Challenges in LPBF of IN625/IN718 Valve Housings:
- Warping and Distortion (Residual Stress):
- Issue: The intense, localized heating and rapid cooling during LPBF generate significant thermal gradients, leading to the build-up of internal residual stresses. Upon removal from the build plate or during post-processing, these stresses can cause the part to warp, distort, or even crack, compromising dimensional accuracy.
- Solutions:
- Build Simulation: Utilizing process simulation software before printing helps predict thermal behavior and distortion, allowing optimization of orientation and support structures to counteract warping tendencies.
- Optimized Support Strategy: Robust, well-designed supports anchor the part firmly to the build plate and help manage thermal stresses during the build. Support design is critical.
- Optimized Scan Strategy: The pattern and parameters used by the laser to melt the powder layers influence heat input and stress accumulation. Strategies like island scanning or sector-based scanning can help mitigate stress.
- Mandatory Stress Relief: Performing a proper stress relief heat treatment before removing the part from the build plate is essential to relax internal stresses and stabilize the component.
- Cracking (Solidification or Post-Heat Treatment):
- Issue: Nickel superalloys, particularly precipitation-hardenable ones like IN718, can be susceptible to cracking either during solidification in the AM process (solidification cracking) or during post-weld heat treatments (strain-age or post-weld heat treatment cracking).
- Solutions:
- Powder Quality Control: Using high-purity powder with controlled chemistry minimizes elements that promote cracking.
- Parameter Optimization: Fine-tuning laser power, speed, and hatch spacing to control the melt pool size, cooling rate, and thermal gradients.
- Optimized Heat Treatment Cycles: Carefully controlling heating/cooling rates and hold times during stress relief, solution annealing, and aging treatments according to established best practices for AM versions of these alloys is crucial to avoid cracking, especially for IN718. Slow heating rates through certain temperature ranges might be required.
- DfAM: Avoiding excessively thick sections or sharp internal corners where stress can concentrate.
- Difficulty in Support Removal:
- Issue: Supports, especially those in complex internal cavities or made from tough materials like Inconel, can be very difficult, time-consuming, and costly to remove without damaging the part. Incomplete removal compromises function and cleanliness.
- Solutions:
- DfAM Focus: Prioritize self-supporting designs where possible. Use optimized support types (e.g., thin walls, small contact points, breakaway structures). Ensure accessibility for removal tools during the design phase.
- Advanced Removal Techniques: Utilizing Wire EDM or specialized electrochemical or abrasive methods where manual removal is impractical.
- Material Choice: In some non-critical applications (though rare for valve housings), using different, more easily removable support materials might be explored if the AM system allows.
- Internal Porosity:
- Issue: Microscopic voids can form within the printed material due to trapped gas (e.g., Argon shielding gas), keyholing (vapor depression instability in the melt pool), or lack of fusion between layers/tracks. Porosity reduces density and severely degrades mechanical properties, especially fatigue strength.
- Solutions:
- Optimized Process Parameters: Developing robust parameter sets (laser power, speed, layer thickness, hatch spacing, gas flow) validated to produce fully dense (>99.5% or higher) material.
- Powder Quality & Handling: Using high-quality, dry, spherical powder with low gas content and proper handling procedures to prevent moisture pickup.
- 熱間静水圧プレス(HIP): The most effective way to eliminate any residual internal porosity, achieving near-100% density and significantly improving fatigue performance. Often considered mandatory for critical applications requiring high Porosity Control.
- Powder Removal from Internal Channels:
- Issue: Completely removing all unfused powder from intricate internal passages after the build can be extremely difficult. Trapped powder is a contaminant and can lead to component malfunction or failure.
- Solutions:
- DfAM for Powder Removal: Designing channels with smooth transitions, sufficient diameters, and strategically placed access ports for draining and flushing. Avoiding “dead ends” where powder can become permanently trapped.
- Post-Processing Procedures: Implementing rigorous cleaning procedures involving vibration tables, compressed air blow-outs (in controlled environments), ultrasonic cleaning, and potentially flushing with appropriate fluids.
- Inspection: Using techniques like CT scanning or borescopes to verify complete powder removal from critical internal areas.
- Surface Finish Limitations:
- Issue: As-built surface roughness, especially on overhangs or internal channels, may not meet requirements for sealing, flow efficiency, or fatigue resistance.
- Solutions:
- Orientation Strategy: Orienting critical surfaces optimally during build setup.
- Parameter Optimization: Fine-tuning parameters can slightly improve finish, but limitations remain.
- 後処理: Incorporating necessary steps like machining, polishing, AFM, or electropolishing for critical surfaces where the as-built finish is insufficient. Acknowledging the need for these steps during the planning phase is crucial.
Mitigating Challenges with Expertise and Partnership:
Successfully navigating these Metal AM Challenges requires more than just access to a printer. It demands deep process knowledge, materials science expertise, rigorous quality control, and often, significant investment in post-processing and inspection capabilities. This is why partnering with an experienced and reputable Metal AM Service Provider is often the most effective approach for companies entering the AM aerospace arena.
Organizations like メット3dp bring decades of collective expertise in metal additive manufacturing, encompassing everything from advanced powder production to sophisticated printing techniques and application development. Their understanding of materials like IN625 and IN718, coupled with robust Quality Management Systems, helps ensure that potential issues are anticipated and addressed proactively through optimized DfAM, meticulous process control, and thorough post-processing. Learning more 私たちについて and our commitment to quality can provide confidence when tackling complex AM projects. By leveraging such partnerships, aerospace companies can significantly de-risk the adoption of AM for critical components like cryogenic valve housings and accelerate their journey towards next-generation manufacturing.
Selecting Your Partner: Choosing the Right Metal AM Service Provider for Aerospace Components
The successful adoption of Additive Manufacturing for mission-critical components like cryogenic rocket valve housings hinges not only on robust design and material selection but, critically, on the capabilities and expertise of your manufacturing partner. Choosing the right Metal AM Service Provider is a strategic decision that significantly impacts quality, reliability, cost, and time-to-market. Given the stringent requirements of the aerospace industry, particularly for components handling cryogenic propellants, the selection process demands careful evaluation beyond just quoting the lowest price. Engineers and procurement managers must look for partners who demonstrate a deep understanding of aerospace standards, materials science, process control, and the entire AM workflow, from design optimization to final inspection.
Key Criteria for Evaluating Metal AM Service Providers for Aerospace:
- Aerospace Certifications & Quality Management System (QMS):
- Requirement: Look for providers holding relevant aerospace certifications, primarily AS9100. This certification demonstrates a robust QMS specifically tailored to the rigorous demands of the aviation, space, and defense industries. It encompasses aspects like traceability, process control, risk management, and continuous improvement.
- Verification: Request copies of certifications and inquire about the maturity and implementation of their QMS. Understand their procedures for material traceability, process documentation, operator training, and non-conformance management.
- Proven Experience with Target Materials & Applications:
- Requirement: Specific experience printing and post-processing the required nickel superalloys (IN625, IN718) for demanding applications is crucial. Aerospace experience is highly preferred over general industrial printing.
- Verification: Ask for case studies, examples of similar components produced, and data demonstrating achievable material properties (tensile strength, yield strength, elongation, fatigue data) for IN625/IN718 built on their machines. Discuss their experience with cryogenic applications specifically, if possible.
- Machine Capabilities, Capacity, and Maintenance:
- Requirement: Ensure the provider has appropriate LPBF machines (e.g., sufficient build volume for the valve housing size, adequate laser power for efficient processing of nickel alloys) and sufficient capacity to meet your prototyping and potential production volume needs. Machines should be well-maintained and calibrated.
- Verification: Inquire about their specific machine models, build envelope dimensions, laser configurations, and maintenance schedules. Understand their production scheduling and capacity planning to ensure they can meet required lead times.
- Powder Quality Control & Traceability:
- Requirement: The provider must have stringent procedures for sourcing, testing, handling, storing, and recycling metal powders to prevent contamination and ensure batch-to-batch consistency. Full traceability from powder batch to final part is essential.
- Verification: Ask about their powder suppliers (do they partner with reputable 高性能金属粉末 manufacturers?), incoming powder inspection processes (chemistry, PSD, morphology, flowability checks), powder handling protocols (inert environments, segregation), and powder lifecycle management (tracking usage, recycling procedures, limits on reuse). Companies like メット3dp, which manufacture their own high-quality powders using advanced atomization techniques, offer inherent advantages in controlling this critical input variable.
- In-House or Managed Post-Processing Capabilities:
- Requirement: As discussed, post-processing (stress relief, heat treatment, HIP, support removal, machining, NDT) is extensive and critical. An ideal partner has strong capabilities in-house or manages these processes seamlessly through certified and trusted external partners.
- Verification: Understand which post-processing steps are performed in-house versus outsourced. If outsourced, verify the quality control and certification standards applied to their subcontractors. Ensure they have proven processes for heat treating IN718 correctly (solution + aging) and access to NADCAP-accredited or equivalent HIP and NDT services.
- Engineering Support & DfAM Expertise:
- Requirement: The provider should offer more than just “print-to-print” services. Look for partners with engineering teams experienced in DfAM who can collaborate on design optimization, support strategy development, build simulation, and troubleshooting.
- Verification: Discuss their DfAM capabilities. Can they provide feedback on your design for manufacturability? Do they utilize simulation tools? Are their engineers accessible for technical discussions? A true Additive Manufacturing Partner collaborates to improve the final product.
- Non-Destructive Testing (NDT) and Inspection:
- Requirement: Robust NDT and dimensional inspection capabilities are non-negotiable for AS9100 Certified AM parts. This includes CT scanning, FPI, CMM, and potentially other methods relevant to valve housings.
- Verification: Review their NDT procedures, equipment list, and operator certifications. Understand their dimensional inspection capabilities and reporting formats. Confirm they can meet the specific inspection requirements outlined in your technical documentation.
- Lead Time Commitments & Communication:
- Requirement: The provider should offer realistic lead time estimates for the entire process (printing and all post-processing) and maintain clear, proactive communication throughout the project lifecycle.
- Verification: Discuss their typical lead times for similar parts. Evaluate their project management approach and communication protocols. Responsiveness and transparency are key indicators of a reliable supplier.
Finding the Right Fit: Beyond the Checklist
While these criteria provide a solid framework, consider the cultural fit and willingness to collaborate. Building complex aerospace components with AM often involves a learning curve and requires a strong partnership between the customer and the supplier. Look for providers who are transparent about challenges, willing to invest in process development if needed, and committed to long-term success.
次のような企業 メット3dp position themselves as comprehensive solution providers, leveraging their deep expertise spanning advanced 金属3Dプリンティング equipment, high-quality powder manufacturing, and application development services. Their focus on critical industries like aerospace, combined with decades of collective expertise, makes them a strong candidate for organizations seeking a reliable partner to manufacture components like cryogenic rocket valve housings. Engaging with potential suppliers, conducting audits (virtual or in-person), and starting with smaller pilot projects can help build confidence before committing to large-scale production. Choosing the right Custom Metal Parts Manufacturer specializing in AM is paramount for aerospace success.
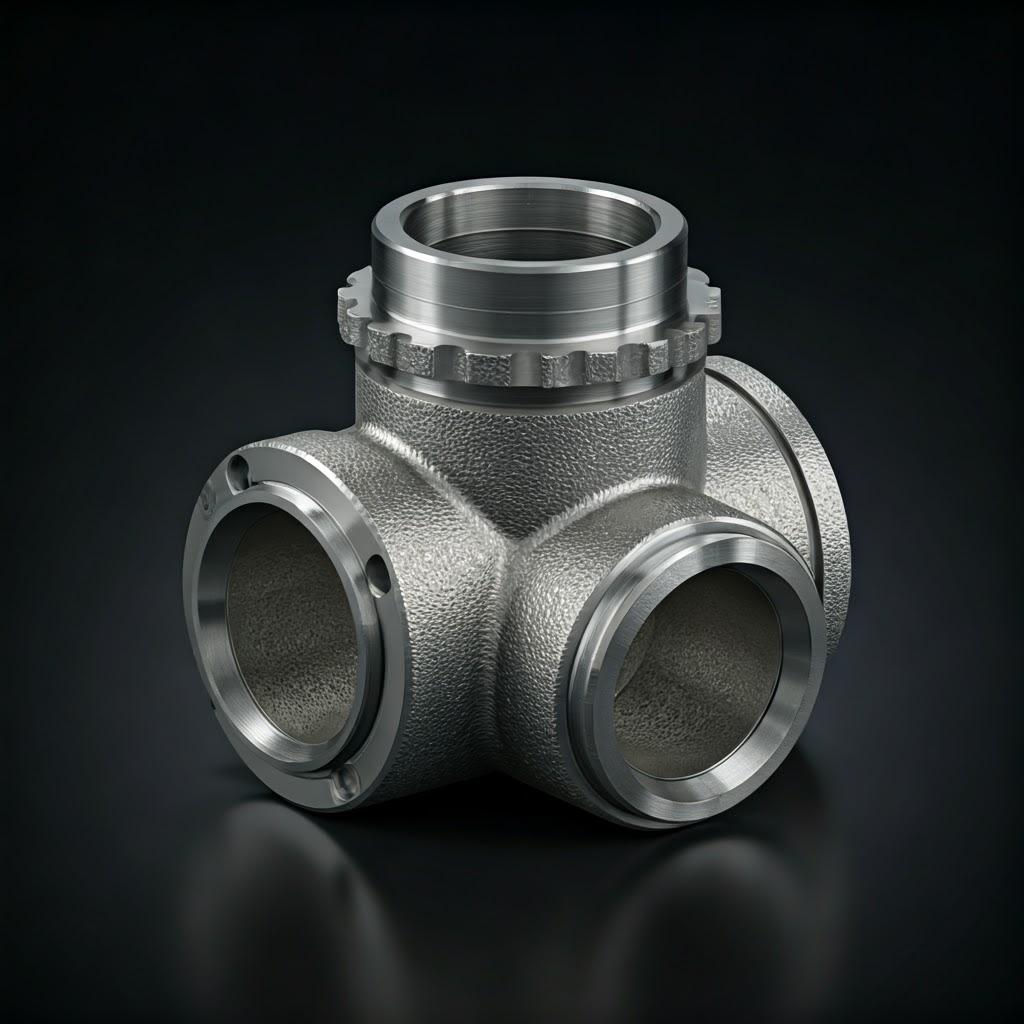
Understanding the Investment: Cost Factors and Lead Times for AM Valve Housings
Additive Manufacturing introduces different cost structures and lead time dynamics compared to traditional methods like casting or machining. Understanding these factors is essential for accurately budgeting projects, making informed decisions about adopting AM, and setting realistic expectations for cryogenic rocket valve housing production. While AM can offer long-term savings through lightweighting and part consolidation, the initial per-part cost, especially for complex superalloy components, requires careful analysis.
Key Factors Influencing the Cost of AM Valve Housings:
- Part Volume & Complexity:
- ボリューム: Larger parts consume more material and require longer build times, directly increasing costs. The bounding box (the smallest cube the part fits into) influences how many parts can fit in a single build.
- 複雑さ: While AM handles complexity well, highly intricate designs may require more extensive support structures, potentially longer build times (due to increased laser scanning), and more intensive post-processing (support removal, inspection), impacting cost. However, complexity achieved through part consolidation can reduce overall system cost by eliminating assembly steps.
- Material Type & Consumption:
- Material Choice: High-performance nickel superalloys like IN625 and IN718 are inherently expensive materials. The cost per kilogram of aerospace-grade, AM-optimized powder is significant.
- Consumption: This includes the material used for the part itself and the material used for support structures. Minimizing support volume through DfAM directly reduces material cost. Efficient nesting of multiple parts within a single build helps amortize powder costs.
- Build Time (Machine Utilization):
- 機械代: Industrial metal AM systems represent a major capital investment. Machine time (cost per hour) is a primary cost driver.
- Factors: Build time is influenced by part height (number of layers), part volume (area to be scanned per layer), layer thickness used, and the specific laser scan strategy employed. Optimizing orientation and nesting to maximize the number of parts per build and minimize build height reduces the per-part machine time cost.
- Support Structure Requirements:
- ボリューム: As mentioned, supports consume material and build time.
- Removal: Labor and specialized processes (e.g., EDM) required for support removal add significant cost, particularly for complex internal supports. DfAM focused on minimizing supports is crucial for cost control.
- Post-Processing Intensity:
- Heat Treatments: Stress relief, solution annealing, and aging require specialized vacuum furnaces and add time and cost.
- HIP: Hot Isostatic Pressing is an expensive batch process requiring specialized equipment; its cost must be factored in, especially when required for fatigue-critical parts.
- 機械加工: Finish machining of critical features adds significant cost due to machine time, tooling, programming, and labor. The extent of machining required directly impacts the final price.
- 表面仕上げ: Polishing, AFM, etc., add further cost based on the area and required smoothness.
- NDT & Inspection: CT scanning, FPI, CMM, etc., require specialized equipment and certified personnel, contributing significantly to the final cost, especially for parts requiring 100% inspection.
- Quality Assurance & Documentation:
- The level of documentation, traceability, process validation, and quality reporting required for aerospace components adds overhead cost compared to standard industrial parts.
- 注文数量:
- Setup Costs: While AM avoids hard tooling, there are still setup costs associated with preparing the build file, machine setup, and post-processing workflow development. These costs are amortized over larger batch sizes.
- ボリュームディスカウント: Wholesale 3D Printing Prices can be negotiated for larger production runs or recurring orders, reflecting efficiencies in machine utilization, powder purchasing, and process optimization.
Typical Lead Times for AM Valve Housings:
Lead times can vary significantly based on complexity, post-processing requirements, and the provider’s backlog. However, AM generally offers advantages, particularly in the prototyping phase.
- プロトタイピング: From final CAD model to an as-built (stress-relieved, support-removed) prototype might take 1~3週間. This rapid turnaround is a major advantage for design iteration.
- Production (including full post-processing): For a fully qualified, heat-treated, HIPed, machined, inspected, and documented valve housing, lead times are longer but often significantly shorter than traditional casting or forging routes, potentially ranging from 4 to 12 weeks, depending heavily on the factors listed above and quantity.
- 比較する: Traditional casting can involve lead times of 3-6 months or more, largely driven by tooling fabrication. Complex machining from billet might fall somewhere in between, depending on complexity and machine availability.
Cost/Lead Time Comparison Summary:
方法 | 金型費用 | 廃棄物 | 部品の複雑さ | 軽量化 | Lead Time (Proto) | Lead Time (Prod) | Per-Part Cost (Low Vol) | Per-Part Cost (High Vol) |
---|---|---|---|---|---|---|---|---|
CNC加工 | Low/Med | 高い | ミディアム | 低い | Med | Med | 高い | Med |
キャスティング | 高い | Med | 高い | Med | 長さ | 長さ | Med | 低い |
鍛造 | 非常に高い | 低い | 低い | 低い | Very Long | 長さ | N/A (High Vol only) | 最低 |
Metal AM (LPBF) | なし | 低い | 非常に高い | 高い | 短い | Med/Short | 非常に高い | Med/High |
Export to Sheets
Conclusion on Investment: 一方 Metal 3D Printing Cost per part for a complex superalloy valve housing might initially seem higher than traditional methods (especially compared to high-volume casting/forging), a total cost of ownership analysis is necessary. When factoring in eliminated tooling costs, reduced material waste, significant weight savings (reducing launch costs), consolidated parts (reducing assembly), and drastically shorter AM Lead Time (accelerating development and improving supply chain responsiveness), AM often presents a compelling value proposition for low-to-medium volume production of critical aerospace components. Evaluating the Additive Manufacturing Pricing requires looking beyond the part itself to its impact on the entire system and lifecycle.
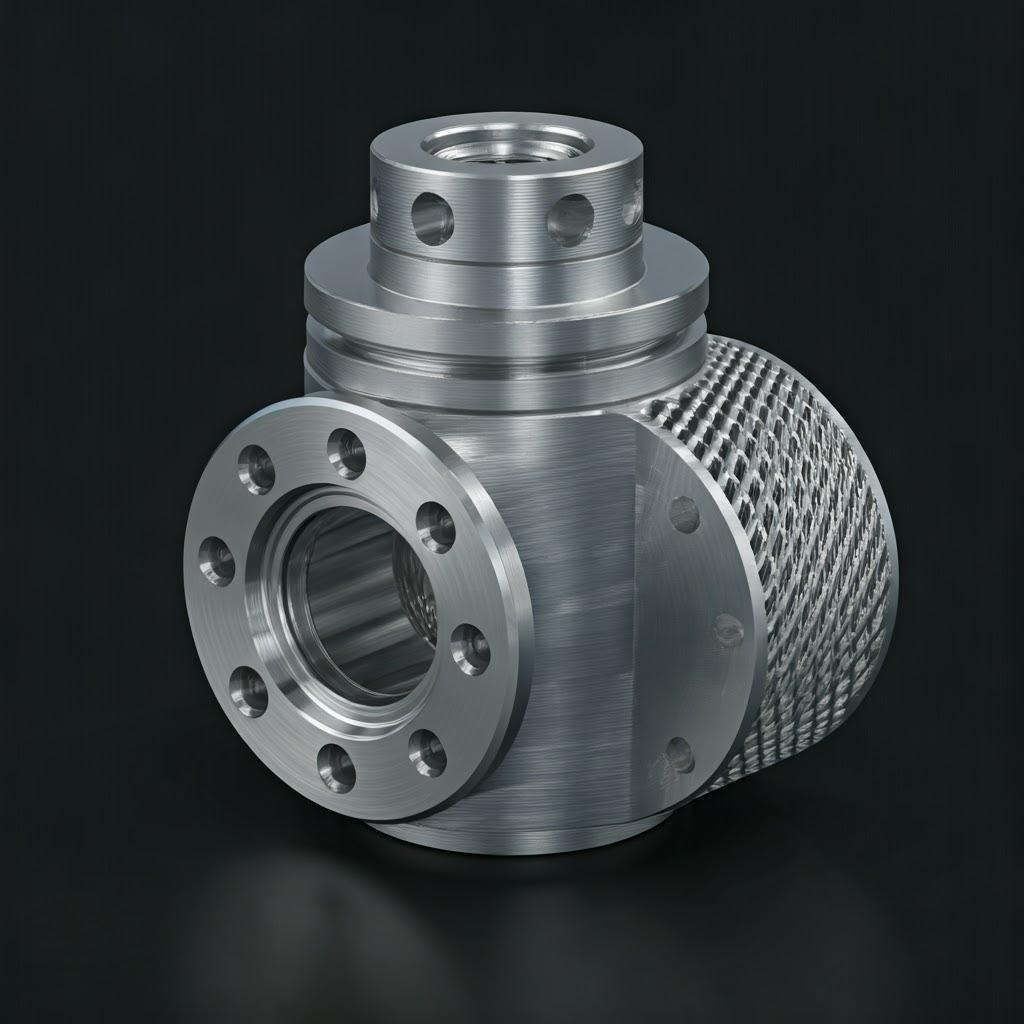
Conclusion: Propelling Future Rocket Systems with Additively Manufactured Valve Housings
The journey to space is paved with technological innovation, demanding components that push the boundaries of performance, reliability, and efficiency. Cryogenic rocket valve housings, operating at the harsh intersection of extreme temperatures and high pressures, exemplify this challenge. As we’ve explored, Metal Additive Manufacturing, particularly Laser Powder Bed Fusion utilizing advanced nickel superalloys like IN625 and IN718, represents a paradigm shift in how these critical components are designed and produced.
AM liberates engineers from the constraints of traditional manufacturing, enabling the creation of valve housings with unparalleled geometric complexity. This translates into tangible benefits: highly optimized internal flow paths for enhanced fluid dynamic performance, integrated features that consolidate multiple parts into one monolithic structure (reducing weight, assembly time, and potential leak paths), and the ability to implement sophisticated lightweighting strategies like topology optimization and lattice structures, which are crucial for reducing launch costs and maximizing payload capacity.
The advantages extend beyond pure performance. The rapid prototyping capabilities inherent in AM drastically shorten development cycles, allowing for faster iteration and validation of new valve designs. Furthermore, the ability to produce parts on-demand without dedicated tooling enhances supply chain resilience and offers unprecedented flexibility – vital attributes in the fast-paced aerospace industry. While challenges related to process control, post-processing complexity, and rigorous quality assurance exist, they are being systematically addressed through advancements in DfAM principles, simulation software, process monitoring, and the expertise of dedicated AM solution providers.
The successful implementation of AM for cryogenic valve housings relies on a holistic approach:
- Intelligent Design (DfAM): Tailoring the component specifically for the additive process.
- 先端材料: Utilizing high-quality, AM-optimized powders like IN625 and IN718 known for their cryogenic performance.
- Robust Processing: Precise control over the LPBF process and meticulous execution of essential post-processing steps like heat treatment, HIP, and machining.
- Strategic Partnership: Collaborating with experienced Metal AM Service Providers who possess the necessary certifications, equipment, and expertise.
次のような企業 メット3dp are at the forefront of this manufacturing revolution, offering integrated solutions that encompass both state-of-the-art printing technology and the production of high-purity metal powders optimized for the most demanding applications. Their commitment to quality and innovation empowers aerospace companies to leverage the full potential of AM Rocket Propulsion technologies. Explore how Met3dp can support your additive manufacturing goals by visiting our ホームページ.
In conclusion, Additive Manufacturing is not merely an alternative production method for cryogenic rocket valve housings; it is an enabling technology driving the future of space exploration. By unlocking new levels of design freedom, performance optimization, and production agility, AM is set to play an increasingly vital role in creating the lighter, faster, more capable, and more cost-effective launch vehicles and spacecraft needed to reach for the stars.
よくある質問(FAQ)
Q1: What are the typical cryogenic temperatures these AM valve housings must withstand?
A1: Rocket valve housings typically manage propellants like Liquid Oxygen (LOX), which boils at -183°C (-297°F), and Liquid Hydrogen (LH2), which boils at -253°C (-423°F). Therefore, the valve housings must maintain structural integrity, avoid brittle fracture, and ensure proper sealing performance at these extremely low operating temperatures. Materials like IN625 and IN718 are chosen specifically for their excellent toughness and strength retention at these cryogenic levels.
Q2: How does the fatigue life of AM IN625/IN718 compare to wrought (traditionally manufactured) materials?
A2: When processed correctly (optimized printing parameters, appropriate heat treatments, and mandatory Hot Isostatic Pressing – HIP), the fatigue life of LPBF-produced IN625 and IN718 can be comparable to, and in some cases even exceed, that of wrought counterparts, especially in specific orientations. HIP is crucial for minimizing internal defects (pores) that typically limit fatigue performance in as-built AM parts. However, factors like surface finish and residual stress also play a role, and thorough testing and qualification specific to the application are always necessary for Metal 3D Printing Properties validation in critical components.
Q3: Can existing valve housing designs (made for casting/machining) be directly printed using AM?
A3: While it’s technically possible to print a legacy design, it’s rarely optimal and fails to leverage AM’s key advantages. Directly printing a design intended for casting or machining often results in excessive support structures, non-optimal orientation, potential printability issues, and unnecessary weight. To fully benefit from AM, a DfAM Redesign is highly recommended. This involves re-evaluating the design based on DfAM principles to optimize topology, consolidate parts, minimize supports, ensure powder removal, and potentially enhance performance beyond the original design.
Q4: What specific quality control measures are essential for AM aerospace parts like valve housings?
A4: Aerospace Quality Control for critical AM parts is multi-faceted and rigorous. Key measures include:
Documentation: Comprehensive traceability records linking powder batch, machine parameters, build data, post-processing records, and inspection results to each unique pa
Powder Control: Strict acceptance testing (chemistry, PSD, flowability), handling protocols, and traceability.
Process Monitoring: In-situ monitoring of the melt pool, laser parameters, and build environment (oxygen levels, temperature).
Post-Processing Validation: Precise control and verification of all heat treatment cycles (using thermocouples on parts), HIP parameters, and machining accuracy.
非破壊検査(NDT): Mandatory CT scanning for internal defects/geometry, FPI for surface defects, and potentially UT/X-ray.
寸法検査: CMM and/or 3D scanning to verify conformance to drawing specifications.
Mechanical Testing: Batch testing of material properties using printed test coupons (tensile, fatigue).
Documentation: Comprehensive traceability records linking powder batch, machine parameters, build data, post-processing records, and inspection results to each unique part.
シェアする
MET3DP Technology Co., LTDは、中国青島に本社を置く積層造形ソリューションのリーディングプロバイダーです。弊社は3Dプリンティング装置と工業用途の高性能金属粉末を専門としています。
関連記事
Met3DPについて
最新情報
製品
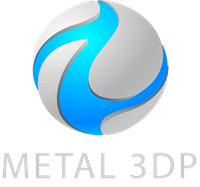
3Dプリンティングと積層造形用金属粉末