3D Printed Surgical Tools with Stainless Steel and Titanium
Inhoudsopgave
Revolutionizing Surgery: The Rise of 3D Printed Surgical Tools in Stainless Steel and Titanium
The operating room is a theatre of precision, where the skill of the surgeon is paramount, but the quality and design of their instruments are equally critical. For centuries, surgical tools have been crafted using traditional methods like forging, casting, and machining. While effective, these techniques often face limitations in producing highly complex geometries, patient-specific designs, or enabling rapid iteration during development. Enter metal additive manufacturing (AM), more commonly known as metal 3D printing – a transformative technology poised to redefine surgical instrument design, manufacturing, and ultimately, patient outcomes.
Metaal 3d printen builds parts layer by layer directly from a digital model using high-performance metal powders. This approach unlocks unprecedented design freedom, allowing for the creation of surgical tools with intricate features, internal channels, lattice structures for weight reduction, and ergonomic forms previously impossible or prohibitively expensive to manufacture. Imagine forceps perfectly contoured to a surgeon’s hand, guides precisely matched to a patient’s unique anatomy derived from CT scans, or complex retractors combining multiple functions into a single, optimized instrument. This is not science fiction; it’s the reality enabled by metal AM.
This technology is particularly impactful in demanding fields like orthopedics, neurosurgery, cardiovascular surgery, and minimally invasive procedures, where standard instruments may not always suffice. The ability to rapidly prototype and produce custom, low-volume, or highly complex instruments offers significant advantages over conventional manufacturing, which often requires expensive tooling and long lead times, especially for specialized designs.
Furthermore, metal AM leverages materials renowned for their suitability in medical applications. Two standouts are 316L stainless steel and Ti-6Al-4V (Grade 5) titanium alloy. Both offer excellent biocompatibility, corrosion resistance, and the mechanical strength required to withstand the rigors of surgery and repeated sterilization cycles. By utilizing advanced powder atomization techniques, providers like Met3dp ensure these materials, in powder form, possess the high sphericity and flowability necessary for producing dense, high-quality printed parts with superior mechanical properties, crucial for mission-critical surgical applications. The integration of metaal 3D printen into the surgical tool supply chain is not just an alternative; it’s an upgrade, promising enhanced performance, personalization, and potentially faster innovation cycles for life-saving devices.
Applications of Additively Manufactured Surgical Instruments: Precision Across Medical Specialties
The versatility of metal 3D printing allows for its application across a wide spectrum of surgical disciplines. Its ability to create complex, customized, and lightweight instruments makes it invaluable where precision and specific functionality are key. Procurement managers and surgical instrument suppliers are increasingly recognizing AM’s potential to address unmet needs and improve procedural efficiency.
- Orthopedics: This field has been an early adopter, leveraging AM for patient-specific surgical guides (PSIs) for knee and hip replacements, ensuring accurate implant placement. Complex drill guides, osteotomes, and customized instrumentation for spinal fusion or trauma surgery are also common applications. The ability to integrate porous or lattice structures can aid in instrument handling and visualization.
- Neurosurgery: The delicate nature of neurosurgery demands exceptionally precise and often miniaturized instruments. AM enables the creation of custom guides for electrode placement in deep brain stimulation, specialized micro-forceps, dissectors, and retractors tailored for navigating complex neural and vascular structures.
- Cardiovascular Surgery: Instruments like custom valve sizers, complex clamps, or specialized delivery system components for minimally invasive procedures benefit from AM’s design freedom. The ability to create tools with integrated features can reduce the number of instruments needed and potentially shorten procedure times.
- Minimally Invasive Surgery (MIS): Laparoscopic and endoscopic procedures rely on long, thin instruments with complex end effectors. AM allows for the creation of ergonomic handles, articulated tool tips, and instruments with internal channels for irrigation, suction, or fiber optics, enhancing surgeon control and procedural capabilities.
- Craniomaxillofacial (CMF) Surgery: Patient-specific cutting guides, repositioning guides, and custom instruments based on CT/MRI data are used for reconstructive surgery, ensuring accuracy in bone cuts and repositioning.
- Dental and Oral Surgery: Custom drill guides for implant placement, specialized extraction tools, and orthodontic instruments can be precisely fabricated using metal AM.
- General Surgery: While many standard instruments suffice, AM finds use in creating specialized retractors, clamps, or forceps for challenging cases or novel surgical approaches.
- Rapid Prototyping and Tool Development: Beyond end-use instruments, AM is invaluable for rapidly iterating designs during the development phase. Surgeons can test different ergonomic forms or functional features quickly and cost-effectively before committing to final production.
Table: Examples of 3D Printed Surgical Tools by Specialty
Medical Specialty | Example AM Applications | Key Benefits Addressed |
---|---|---|
Orthopedie | Patient-Specific Instruments (PSIs), Custom Drill Guides, Spinal Instrumentation | Accuracy, Reduced OR Time, Complex Geometry |
Neurosurgery | Micro-instruments, Custom Electrode Guides, Specialized Retractors | Miniaturization, Precision, Patient-Specific Adaptation |
Cardiovascular | Valve Sizers, Complex Clamps, MIS Delivery System Components | Customization, Integrated Features, Complex Geometries |
Minimally Invasive | Articulating End Effectors, Ergonomic Handles, Instruments with Internal Channels | Functionality, Ergonomics, Miniaturization |
Craniomaxillofacial | Patient-Specific Cutting/Repositioning Guides, Custom Fixation Tools | Accuracy, Predictability, Reduced Surgical Time |
Dental/Oral Surgery | Implant Drill Guides, Specialized Extraction Tools | Precision, Custom Fit, Efficiency |
General Surgery | Specialized Retractors, Custom Clamps for Unique Cases | Niche Applications, Addressing Specific Anatomical Needs |
Tool Development | Functional Prototypes, Ergonomic Models | Speed, Cost-Effectiveness, Design Iteration |
Export to Sheets
The ability to tailor instruments not just to the procedure, but potentially to the individual patient’s anatomy or the surgeon’s preference, marks a significant shift towards personalized surgical care, driven by advancements in additive manufacturing technology.
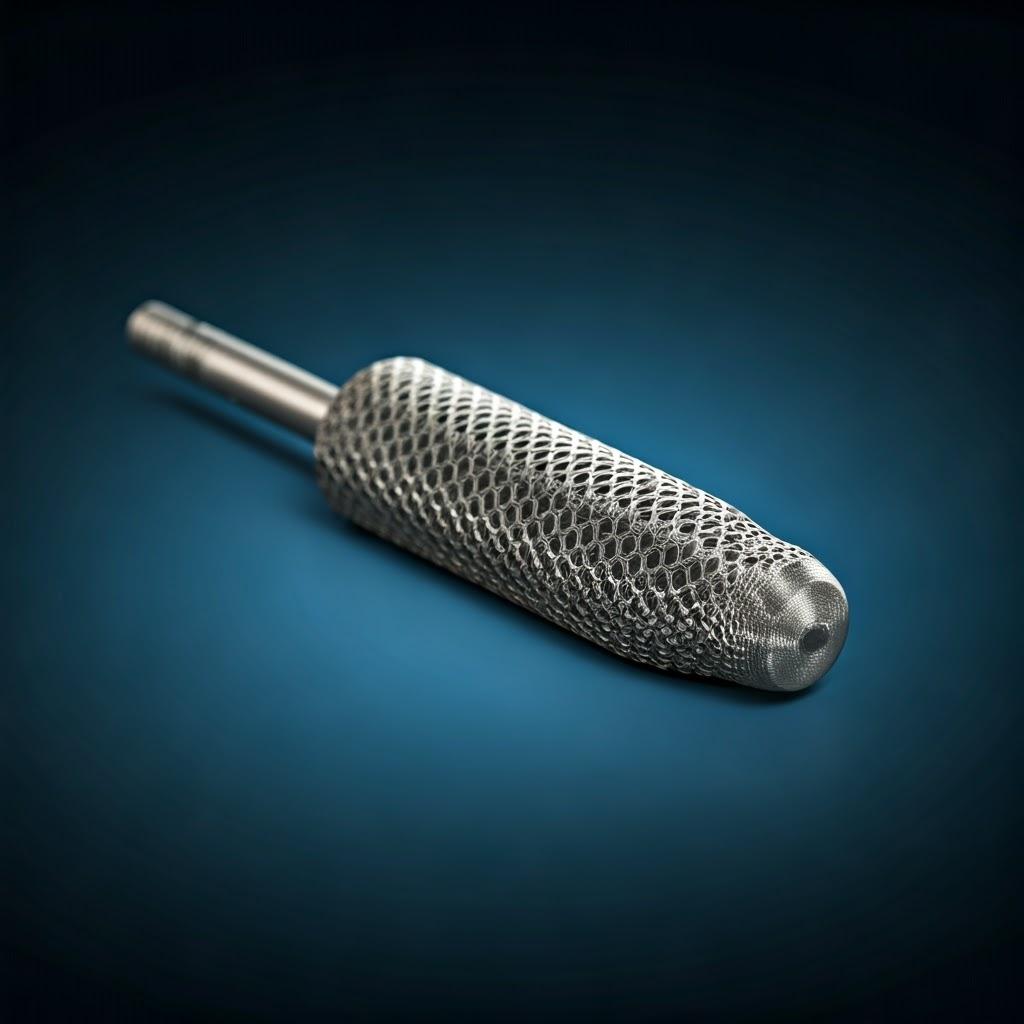
Why Metal 3D Printing is Transforming Surgical Instrument Manufacturing
Traditional surgical instrument manufacturing relies on subtractive methods (machining away material) or formative methods (casting, forging). While mature and reliable for standard instruments, these processes present hurdles that metal additive manufacturing effectively overcomes, particularly for specialized, custom, or complex tools. For wholesale buyers and medical device companies looking for innovative solutions and reliable custom surgical instrument suppliers, understanding these advantages is crucial.
Key Advantages of Metal AM for Surgical Tools:
- Ongeëvenaarde ontwerpvrijheid: AM builds parts layer-by-layer, enabling the creation of geometries impossible with traditional methods. This includes:
- Internal Channels: For irrigation, suction, or housing fiber optics.
- Roosterstructuren: To reduce weight without sacrificing strength, improving ergonomics and reducing surgeon fatigue.
- Complex Curves and Contours: For patient-specific matching or improved handling.
- Deelconsolidatie: Combining multiple components of a traditional assembly into a single printed part, reducing complexity, potential failure points, and assembly time.
- Mass Customization and Patient-Specific Instruments (PSIs): AM excels at producing unique parts directly from digital models (e.g., CT/MRI scans). This allows for:
- Patient-Matched Guides: Improving surgical accuracy in orthopedics, CMF, and neurosurgery.
- Surgeon-Specific Ergonomics: Tools designed for optimal comfort and control for individual surgeons.
- Productie op aanvraag: Manufacturing specialized tools as needed, reducing inventory requirements for rarely used items.
- Rapid Prototyping and Accelerated Innovation: New surgical tool designs can be prototyped, tested, and iterated much faster and more cost-effectively than with traditional methods requiring dedicated tooling. This speeds up the development cycle for novel surgical approaches and devices.
- Materiaalefficiëntie: AM typically uses only the material needed to build the part and its supports, resulting in less material waste compared to subtractive manufacturing, especially for complex designs (buy-to-fly ratio).
- Supply Chain Optimization: Digital manufacturing enables decentralized production. Tool designs can be sent digitally to certified printing facilities closer to the point of use, potentially reducing lead times and logistics complexity, although stringent quality control remains paramount in the medical field.
- Potential for Enhanced Functionality: The design freedom allows for integrating features that improve performance, such as textured surfaces for better grip, optimized cutting edges, or integrated sensing capabilities (in development).
Comparison: Metal AM vs. Traditional Manufacturing for Surgical Tools
Functie | Metaal Additive Manufacturing (AM) | Traditional Manufacturing (Forging, Machining, Casting) |
---|---|---|
Complexiteit | High complexity achievable with minimal cost increase | Complexity significantly increases cost & difficulty |
Maatwerk | Ideal for patient-specific & low-volume custom parts | Expensive and slow for unique/custom parts |
Lead Time (Proto) | Fast (days) | Moderate to Slow (weeks/months for tooling) |
Lead Time (Prod) | Moderate (depends on volume, post-processing) | Fast for high-volume standard parts |
Gereedschapskosten | None (tool-less manufacturing) | Hoge initiële gereedschapskosten |
Materiaal Afval | Generally lower (better buy-to-fly ratio) | Can be significant, especially with machining |
Ontwerpvrijheid | Very High (internal channels, lattices, organic shapes) | Limited by machining/molding constraints |
Deel Consolidatie | Excellent capability | Limited, often requires assembly |
Ideal Volume | Low-to-medium volume, prototypes, custom | High volume, standard designs |
Export to Sheets
While AM won’t replace traditional manufacturing for all surgical tools (especially high-volume, simple instruments where cost per part is lowest with conventional methods), it provides a powerful, complementary pathway for innovation, customization, and solving complex surgical challenges. Companies like Met3dp, with deep expertise in both advanced powder manufacturing and precision printing processes, are key enablers in this transformation.
Biocompatible Materials Spotlight: 316L Stainless Steel vs. Ti-6Al-4V Titanium for Surgical Tools
Material choice is fundamental in surgical instrument design. Instruments must be strong, durable, resistant to corrosion from bodily fluids and harsh sterilization processes, and above all, biocompatible – meaning they do not elicit harmful responses when interacting with biological tissue. For metal 3D printing of surgical tools, two materials dominate: 316L Stainless Steel and Ti-6Al-4V (Grade 5) Titanium alloy. Understanding their properties is essential for engineers and procurement managers specifying materials for medical device additive manufacturing.
316L roestvrij staal:
- Samenstelling: An austenitic stainless steel alloy containing chromium, nickel, and molybdenum. The ‘L’ denotes low carbon content (<0.03%), which improves corrosion resistance, particularly after welding or stress relieving – relevant considerations after the thermal cycles of 3D printing.
- Belangrijkste eigenschappen:
- Uitstekende corrosiebestendigheid: Highly resistant to general corrosion, pitting, and crevice corrosion, crucial for exposure to bodily fluids and sterilization agents (e.g., autoclaving).
- Good Biocompatibility: Widely accepted for temporary and some permanent contact applications within the human body (per standards like ISO 10993).
- High Ductility and Formability: Although less relevant in AM than traditional methods, it indicates good toughness.
- Goede sterkte en hardheid: Sufficient for many surgical applications like retractors, handles, and general instruments.
- Niet-magnetisch: Generally considered non-magnetic in the annealed state, which can be important for MRI compatibility, although some residual magnetism can be induced by cold work or potentially during AM processing.
- Kosteneffectief: Generally less expensive than titanium alloys.
- Well-Established: Long history of use in medical devices, providing extensive data and familiarity.
Ti-6Al-4V (Grade 5) Titanium Alloy:
- Samenstelling: An alpha-beta titanium alloy containing 6% aluminum and 4% vanadium. Often specified as Ti-6Al-4V ELI (Extra Low Interstitials, Grade 23) for critical surgical implants due to improved ductility and fracture toughness, though Grade 5 is common for instruments.
- Belangrijkste eigenschappen:
- Exceptional Biocompatibility: Considered one of the most biocompatible metals, widely used for long-term implants (joints, dental) and instruments. Forms a stable, passive oxide layer.
- Uitstekende corrosiebestendigheid: Superior resistance to bodily fluids and various chemical environments.
- Hoge verhouding sterkte/gewicht: Significantly lighter than steel (approx. 40-45% lower density) but with comparable or higher strength. This is crucial for reducing surgeon fatigue with handheld instruments and for instruments used in weight-sensitive applications.
- Good Fatigue Strength: Important for instruments subjected to repeated stress cycles.
- Non-Magnetic/MRI Compatible: Inherently non-magnetic, making it ideal for instruments used during MRI procedures.
- Osseointegration Potential: While more relevant for implants, the surface can be treated to encourage bone growth if needed for certain specialized devices.
- Hogere kosten: More expensive raw material and potentially more challenging to process compared to 316L.
Table: Comparison of 316L and Ti-6Al-4V for 3D Printed Surgical Tools
Eigendom | 316L roestvrij staal | Ti-6Al-4V Titaniumlegering | Significance for Surgical Tools |
---|---|---|---|
Biocompatibiliteit | Good (ISO 10993) | Excellent (ISO 10993) | Essential for patient safety, Ti-6Al-4V often preferred for implants |
Corrosieweerstand | Uitstekend | Excellent / Superior | Critical for longevity, sterilization resistance |
Dikte | ~8,0 g/cm³ | ~4.4 g/cm³ | Ti-6Al-4V offers significant weight savings (ergonomics) |
Tensile Strength (Typical) | Moderate-High (~550-700 MPa, printed) | Very High (~900-1100 MPa, printed) | Higher strength allows for more delicate/robust designs with Ti |
Stiffness (Young’s Modulus) | High (~193 GPa) | Moderate (~114 GPa) | 316L is stiffer; Ti-6Al-4V is more flexible |
Hardheid | Goed | Goed | Important for wear resistance, maintaining sharp edges |
MRI Compatibility | Generally Non-Magnetic (process dependent) | Fully Non-Magnetic | Crucial for use in MRI environments |
Kosten | Lager | Hoger | Significant factor in material selection |
Typical AM Applications | General instruments, handles, retractors | Implants, high-strength tools, lightweight tools, MRI tools | Matches material properties to application needs |
Export to Sheets
Material Quality Matters: Regardless of the chosen alloy, the quality of the metal powder used in the AM process is critical. Factors like particle size distribution, sphericity, flowability, and purity directly impact the density, mechanical properties, and surface finish of the final printed part. Met3dp utilizes industry-leading gas atomization and Plasma Rotating Electrode Process (PREP) technologies to produce high-quality spherical metal powders, including medical-grade 316L and Ti-6Al-4V, ensuring optimal performance and consistency for demanding surgical tool applications. Choosing a supplier with robust powder production and quality control capabilities is paramount for reliable medical device manufacturing.
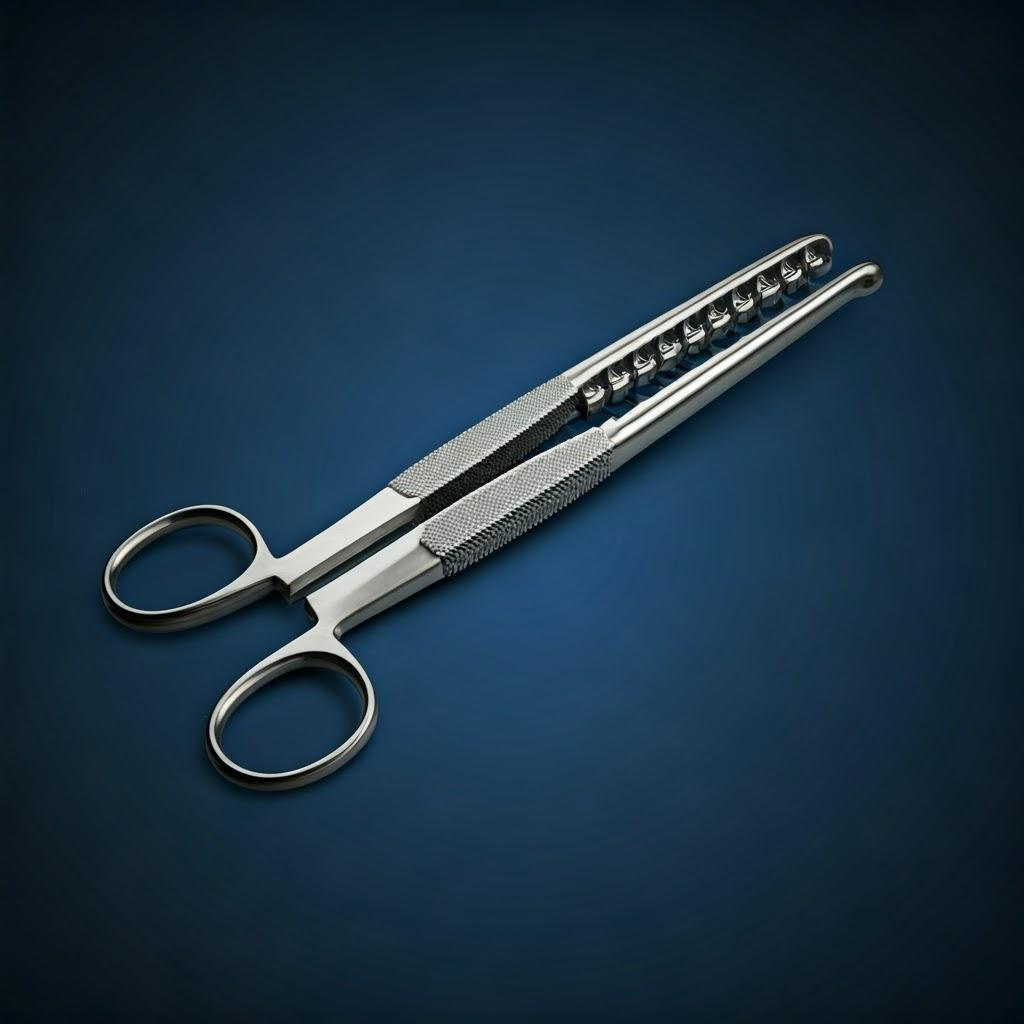
Design for Additive Manufacturing (DfAM) Principles for High-Performance Surgical Tools
Simply replicating a traditionally designed surgical tool using 3D printing rarely unlocks the full potential of additive manufacturing. To truly leverage the benefits of complexity, customization, and performance enhancement, engineers must embrace Design for Additive Manufacturing (DfAM). DfAM involves designing parts with the specific capabilities and constraints of the AM process in mind. For surgical instruments, this means optimizing for functionality, ergonomics, sterilizability, and manufacturing efficiency.
Applying DfAM principles is crucial for creating tools that are not only feasible to print but are also superior in performance and handling. Partnering with an experienced AM provider like Met3dp, who understands the nuances of different afdrukmethoden and materials, can significantly aid in optimizing designs.
Key DfAM Considerations for Surgical Tools:
- Support Structure Optimization: AM processes often require support structures to anchor the part to the build plate and support overhanging features during printing.
- Beperk overstekken tot een minimum: Orient the part on the build plate to reduce steeply angled or horizontal overhangs (>45° often requires support).
- Design Self-Supporting Angles: Utilize chamfers or gentler angles where possible.
- Accessibility for Removal: Ensure supports can be physically reached and removed without damaging delicate features. Consider designing access ports if necessary.
- Impact on Surface Finish: Supported surfaces typically have a rougher finish and may require more intensive post-processing. Design critical surfaces to be self-supporting where possible.
- Wall Thickness and Feature Size: AM processes have minimum printable wall thicknesses and feature sizes.
- Minimum Wall Thickness: Typically ranges from 0.3mm to 0.8mm depending on the technology (e.g., LPBF vs. SEBM) and material. Ensure walls are thick enough for structural integrity and handling.
- Small Feature Resolution: Consider the laser spot size or electron beam diameter when designing very fine features like serrations or pins.
- Hole Diameters: Small holes (<1mm) can be challenging to print accurately and may require post-drilling. Design accordingly or allow for machining.
- Internal Channels and Cavities: AM excels at creating complex internal geometries for functions like fluid delivery, suction, or cable routing.
- Self-Supporting Shapes: Design internal channels with teardrop or diamond shapes to avoid the need for internal supports, which are very difficult or impossible to remove.
- Powder Removal: Include drain holes or access points to ensure all unfused powder can be removed from internal cavities post-printing – critical for biocompatibility.
- Smooth Transitions: Avoid sharp corners internally that could trap debris or hinder flow.
- Lattice Structures and Lightweighting: Incorporating internal lattice or topology-optimized structures can significantly reduce instrument weight, improving ergonomics and reducing surgeon fatigue, while maintaining necessary stiffness and strength. This is particularly beneficial for titanium instruments.
- Deelconsolidatie: Identify opportunities to combine multiple components of a traditional instrument assembly into a single, monolithic printed part. This reduces assembly time, cost, potential failure points, and simplifies the supply chain.
- Surface Texture: Design functional textures directly into the part, such as grips on handles or specific roughness on working ends, potentially reducing post-processing steps.
- Sterilization and Cleaning Considerations: Design instruments with smooth surfaces, rounded edges, and minimal crevices where biological debris could be trapped. Avoid features that make thorough cleaning and sterilization difficult. Ensure powder removal from internal channels is feasible and verifiable.
- Material Selection Integration: Design choices may be influenced by material properties. For instance, titanium’s lower stiffness might require different structural considerations than stainless steel for the same application.
Table: DfAM Guidelines Summary for Surgical Tools
DfAM Principle | Richtlijn | Rationale for Surgical Tools |
---|---|---|
Orientation | Optimize for minimal supports, best surface finish on critical areas | Reduces post-processing, ensures critical surface quality |
Ondersteunt | Minimize overhangs (>45°), ensure removal access | Cost, lead time, surface finish, avoid damage |
Wanddikte | Adhere to process minimums (e.g., >0.4mm), ensure structural integrity | Printability, strength, handling |
Internal Channels | Use self-supporting shapes, design for powder removal | Functionality, prevents trapped powder (biocompatibility risk) |
Lichtgewicht | Utilize lattices/topology optimization where appropriate | Improved ergonomics, reduced surgeon fatigue |
Deel Consolidatie | Combine multiple components into one printed part | Reduced assembly, fewer failure points, simpler supply chain |
Cleanability | Design smooth surfaces, avoid deep crevices, ensure powder removal path | Effective sterilization, prevents bioburden accumulation |
Export to Sheets
By thinking additively from the start, manufacturers can create next-generation surgical tools that offer superior performance, ergonomics, and customization, moving beyond the limitations of traditional manufacturing paradigms.
Achieving Critical Tolerances, Surface Finish, and Accuracy in 3D Printed Surgical Instruments
While design freedom is a major draw, surgical instruments demand precision. Critical dimensions, interfacing features, and appropriate surface characteristics are non-negotiable for functionality and safety. Understanding the achievable levels of tolerance, surface finish, and dimensional accuracy with metal AM is vital for engineers and procurement managers evaluating the technology for medical device manufacturing.
Tolerances:
- General Tolerances: As-printed metal AM parts typically achieve tolerances in the range of ISO 2768 medium (m) or sometimes fine (f). This translates to roughly ±0.1mm to ±0.3mm for smaller dimensions, with tolerance ranges increasing for larger parts due to thermal effects during the build.
- Critical Tolerances: For features requiring tighter tolerances (e.g., mating surfaces, locking mechanisms, precise diameters), post-process machining (CNC milling, turning, grinding) is often necessary. Tolerances comparable to conventional machining (e.g., ±0.01mm to ±0.05mm) can be achieved on specific features via these secondary operations.
- Process Dependency: Tolerances can vary slightly between different AM processes (e.g., Laser Powder Bed Fusion – LPBF vs. Selective Electron Beam Melting – SEBM) and machines. High-end industrial systems, like those utilized by Met3dp, offer better process control and consistency, leading to improved as-printed accuracy.
Surface Finish (Roughness):
- As-Printed: The surface finish of AM parts is inherently rougher than machined surfaces due to the layer-by-layer process and partially melted powder particles adhering to the surface.
- Typical Ra (average roughness) values range from 5 µm to 20 µm, depending on the material, process parameters, particle size distribution, and surface orientation (upward-facing surfaces are generally smoother than downward-facing or supported surfaces).
- Post-Processing for Improvement: For most surgical applications, the as-printed surface is too rough. Various post-processing techniques are employed:
- Abrasive Blasting (Bead/Sand Blasting): Provides a uniform matte finish, removing loose particles (Ra typically 3-6 µm).
- Tumbling/Vibratory Finishing: Uses media to smooth surfaces and edges, effective for batches of parts (Ra can reach 1-3 µm).
- Electropolishing: An electrochemical process that removes a thin layer of material, resulting in a very smooth, bright, clean, and highly corrosion-resistant surface. Often preferred for stainless steel surgical tools (Ra < 0.8 µm, sometimes < 0.4 µm).
- Manual Polishing: For achieving mirror finishes on specific areas, though labor-intensive.
- Bewerking: Creates smooth, precise surfaces on critical features.
- Importance for Surgical Tools: Smooth surfaces are crucial for cleanability, reducing friction, minimizing tissue trauma, and preventing bioburden adhesion. Specific Ra targets are often required depending on the instrument’s function and contact type.
Dimensional Accuracy and Validation:
- Procesbeheersing: Achieving consistent dimensional accuracy requires tight control over the entire AM process, including machine calibration, consistent powder quality (like the high-sphericity powders from Met3dp), optimized process parameters, and controlled thermal management.
- Validation: Dimensional accuracy must be verified using appropriate metrology equipment.
- Calipers/Micrometers: For basic dimensional checks.
- Coordinate Measuring Machines (CMM): For high-accuracy measurement of complex geometries and GD&T (Geometric Dimensioning and Tolerancing) verification.
- 3D Scanning: For comparing the final part geometry against the original CAD model.
- Quality Management System (QMS): A robust QMS (ideally ISO 13485 certified for medical devices) ensures processes are documented, controlled, and validated to consistently produce parts meeting dimensional specifications.
Table: Typical Precision Levels in Metal AM for Surgical Tools
Parameter | As-Printed Range | Post-Processed Potential | Belang |
---|---|---|---|
Tolerantie | ±0.1 to ±0.3 mm (ISO 2768-m/f) | ±0.01 to ±0.05 mm (via Machining) | Fit, function, mating parts |
Surface Finish (Ra) | 5 µm – 20 µm | < 0.8 µm (Electropolishing/Polishing) | Cleanability, biocompatibility, friction, wear |
Eigenschap Resolutie | ~0.3 – 0.8 mm min wall/feature | Defined by AM process | Ability to create fine details |
Validation Method | CMM, 3D Scanning, Calipers | CMM, Surface Profilometry | Ensuring parts meet design specifications & standards |
Export to Sheets
While AM offers incredible design flexibility, achieving the necessary precision for surgical tools often requires a combination of controlled printing and targeted post-processing, guided by rigorous quality control procedures.
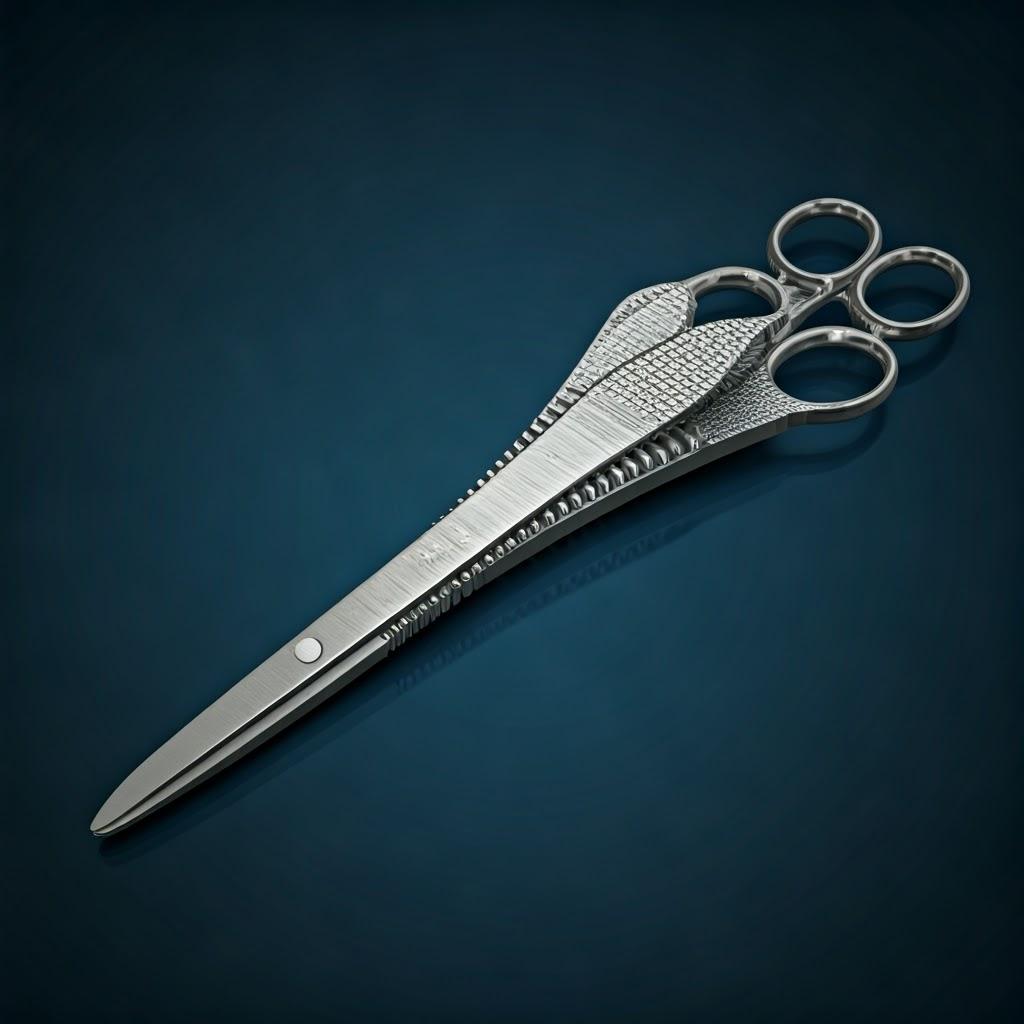
Essential Post-Processing Steps for Functional and Safe 3D Printed Surgical Tools
A metal 3D printed part is rarely ready for use straight out of the machine, especially not for critical applications like surgical instruments. A series of essential post-processing steps are required to transform the raw printed part into a functional, safe, and sterile medical device. These steps address residual stresses, remove temporary structures, achieve the required surface finish and tolerances, and ensure cleanliness. The specific workflow can vary depending on the material, design complexity, and application requirements.
Typical Post-Processing Workflow:
- Stress Relief / Heat Treatment:
- Why: The rapid heating and cooling cycles during AM induce internal stresses within the part. Heat treatment (annealing, stress relieving) at specific temperatures homogenizes the microstructure, relieves these stresses (preventing potential distortion or cracking), and can optimize mechanical properties like ductility and strength according to material standards (e.g., ASTM standards for 316L or Ti-6Al-4V).
- How: Performed in a controlled atmosphere (vacuum or inert gas) furnace following validated protocols specific to the alloy.
- Part Removal from Build Plate:
- How: Parts are typically cut from the build plate using wire EDM (Electrical Discharge Machining) or a bandsaw.
- Draagstructuur verwijderen:
- Why: Supports are necessary during the build but must be removed afterward.
- How: This can be done manually (breaking or cutting), via CNC machining, or sometimes wire EDM. Care must be taken not to damage the part itself. Access can be challenging for complex internal supports.
- Cleaning & Powder Removal:
- Why: All residual metal powder, especially from internal channels or complex features, must be meticulously removed. Trapped powder is a significant biocompatibility risk.
- How: Methods include compressed air blowing, ultrasonic cleaning baths, and potentially micro-blasting. Design features (drain holes) aid this process.
- Afwerking oppervlak:
- Why: To achieve the required smoothness for cleanability, reduced friction, and biocompatibility.
- How: As discussed previously, this involves steps like:
- Abrasive Blasting (e.g., bead blasting) for a uniform matte finish.
- Tumbling/Vibratory Finishing for smoothing edges and surfaces.
- Electropolishing (especially for 316L) for a very smooth, clean, passive surface.
- Manual Polishing for specific high-gloss requirements.
- Machining (If Required):
- Why: To achieve critical tolerances, precise hole diameters, flat mating surfaces, or sharp cutting edges that cannot be reliably produced by AM alone.
- How: CNC milling, turning, grinding, or drilling specific features.
- Final Cleaning and Passivation:
- Why: To remove any residues from machining or handling and, particularly for stainless steel and titanium, to ensure the formation of a stable, passive oxide layer that enhances corrosion resistance and biocompatibility.
- How: Involves validated multi-stage cleaning processes using detergents, solvents, and purified water, potentially followed by a specific passivation treatment (e.g., nitric acid or citric acid bath for stainless steels).
- Inspection and Quality Control:
- Why: To verify dimensional accuracy, surface finish, material properties, and cleanliness meet all specifications.
- How: CMM, visual inspection, surface profilometry, potentially material testing on sample coupons printed alongside the parts.
- Sterilization Validation (Performed by Medical Device Manufacturer):
- Why: Although not typically performed by the AM service provider, the instrument must be compatible with standard sterilization methods (e.g., autoclaving, gamma radiation, EtO). The manufacturing process, including cleaning, must ensure the device can be effectively sterilized. Validation protocols confirm the chosen sterilization method consistently achieves the required sterility assurance level (SAL).
Simplified Post-Processing Flowchart Example:
Code snippet
graph TD
A[3D Print Complete] --> B{Stress Relief / Heat Treatment};
B --> C{Part Removal from Plate};
C --> D{Support Removal};
D --> E{Initial Cleaning / Powder Removal};
E --> F{Surface Finishing (Blasting/Tumbling etc.)};
F --> G{Machining (If Req.)};
G --> H{Final Cleaning & Passivation};
H --> I[Inspection (Dimensions, Surface)];
I --> J(Ready for Packaging/Sterilization);
%% Optional Paths
F --> H; %% Skip Machining
E --> G; %% Machining before general surface finish
Each step requires careful control and validation, especially within the framework of a medical device quality management system like ISO 13485, to ensure the final surgical tool is safe and effective.
Overcoming Challenges in Metal Additive Manufacturing for Surgical Applications
While metal AM offers significant advantages for surgical tool production, it’s not without its challenges. Successfully manufacturing high-quality, reliable medical devices requires anticipating and mitigating potential issues throughout the design, printing, and post-processing stages. Understanding these challenges helps in selecting capable manufacturing partners and setting realistic expectations.
Common Challenges and Mitigation Strategies:
- Residual Stress and Warpage:
- Uitdaging: Rapid heating/cooling cycles create internal stresses that can cause parts to warp or distort during or after printing, or even crack.
- Mitigation:
- Simulation: Using thermal simulation software to predict stress buildup and optimize part orientation and support strategies.
- Process Parameters: Optimizing laser/beam power, scan speed, and layer thickness.
- Platform Heating: Pre-heating the build platform (common in SEBM, possible in some LPBF) reduces thermal gradients.
- Support Strategy: Robust support structures help anchor the part and dissipate heat.
- Verlichting van stress: Post-print heat treatment is crucial for relieving residual stresses.
- Draagstructuur verwijderen:
- Uitdaging: Removing supports, especially from complex internal geometries or delicate features, can be difficult, time-consuming, and risk damaging the part. Supported surfaces are typically rough.
- Mitigation:
- DfAM: Designing for minimal support usage and easy access (see DfAM section).
- Optimized Supports: Using support structures that are strong enough during the build but designed to break away more easily.
- Advanced Removal Techniques: Utilizing wire EDM or precision machining for difficult supports.
- Process Selection: Some processes might require fewer supports for certain geometries.
- Achieving Required Surface Finish and Cleanliness:
- Uitdaging: As-printed surfaces are rough and unsuitable for most surgical applications. Achieving consistent smoothness (<0.8 µm Ra often desired) and ensuring removal of all powder/debris, especially from internal channels, is critical but challenging.
- Mitigation:
- Optimized Parameters: Fine-tuning print parameters can improve as-printed finish slightly.
- Effective Post-Processing: Employing appropriate techniques like electropolishing, tumbling, or blasting, tailored to the material and geometry.
- DfAM for Cleanability: Designing parts without features that trap powder or contaminants.
- Validated Cleaning Protocols: Implementing rigorous, multi-stage cleaning processes with verification steps.
- Porositeitscontrole:
- Uitdaging: Small voids or pores can sometimes form within the printed material due to incomplete melting, gas entrapment, or keyholing effects. Porosity can degrade mechanical properties (especially fatigue strength) and potentially harbor bacteria.
- Mitigation:
- High-Quality Powder: Using powder with consistent sphericity, size distribution, and low gas content, such as those produced via Met3dp’s advanced atomization. Find examples on our productpagina.
- Optimized Process Parameters: Developing and validating parameter sets that ensure full melting and density (>99.5% is common, >99.9% often achievable).
- Process Monitoring: Utilizing in-situ monitoring systems (e.g., melt pool monitoring) to detect potential issues during the build.
- Heet isostatisch persen (HIP): A post-processing step involving high temperature and pressure to close internal pores (often used for critical fatigue-loaded parts, adds cost).
- Process Consistency and Repeatability:
- Uitdaging: Ensuring that every part produced meets the same specifications requires tight control over numerous variables (machine, material, parameters, environment).
- Mitigation:
- Robust QMS: Implementing ISO 13485 or a comparable quality system.
- Machine Calibration & Maintenance: Regular, documented calibration and preventative maintenance.
- Powder Management: Strict control over powder sourcing, handling, storage, and recycling/rejuvenation protocols.
- Process Validation (IQ/OQ/PQ): Rigorous Installation Qualification (IQ), Operational Qualification (OQ), and Performance Qualification (PQ) for machines and processes.
- Statistical Process Control (SPC): Monitoring key process indicators to ensure stability.
- Regulatory Compliance and Validation:
- Uitdaging: Medical devices are highly regulated. Demonstrating that AM processes and resulting parts meet stringent requirements (biocompatibility, mechanical properties, sterility) requires extensive testing and documentation.
- Mitigation:
- Partnering with Experienced Suppliers: Choosing suppliers like Met3dp with experience in medical device manufacturing and appropriate certifications (e.g., ISO 13485).
- Materiaalcertificering: Using certified medical-grade materials with full traceability.
- Thorough Validation: Conducting comprehensive testing (mechanical, chemical, biocompatibility) according to relevant standards (ISO, ASTM).
- Documentation: Maintaining detailed records of all manufacturing and quality control steps.
Overcoming these challenges requires a combination of advanced technology, materials science expertise, rigorous process control, and a deep understanding of medical device requirements. Choosing a manufacturing partner with demonstrated capabilities in these areas is essential for successfully bringing 3D printed surgical tools to market.
Selecting the Right Metal 3D Printing Partner for Medical Device Manufacturing
Choosing the right manufacturing partner is arguably as critical as the design and material selection when developing 3D printed surgical tools. The unique requirements of the medical device industry – stringent regulations, demands for high precision, biocompatibility, and absolute reliability – necessitate partnering with a metal additive manufacturing provider that possesses specific expertise and robust quality systems. Procurement managers and engineering teams should evaluate potential suppliers based on a comprehensive set of criteria.
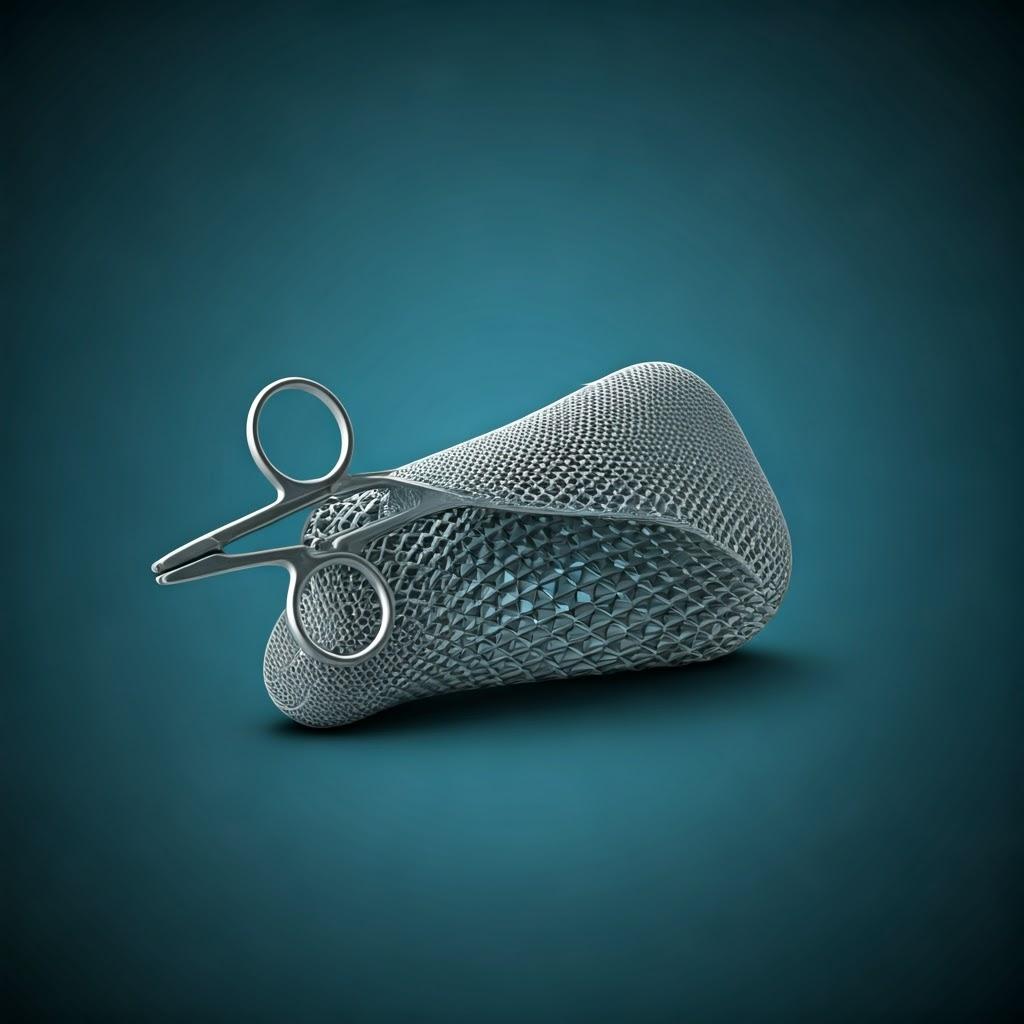
Key Criteria for Evaluating Metal AM Suppliers for Surgical Tools:
- Quality Management System (QMS) and Certifications:
- ISO 13485 Certification: This is the international standard for quality management systems for medical devices. Certification demonstrates the supplier has established processes for design controls, risk management, traceability, process validation, and corrective/preventive actions tailored to the medical industry. This should be a primary requirement.
- ISO 9001 Certification: A general QMS standard, often held alongside ISO 13485, indicating broader quality process control.
- Medical Device Experience:
- Proven Track Record: Does the supplier have demonstrable experience manufacturing medical components, specifically surgical instruments or implants? Ask for case studies, examples (under NDA if necessary), and references.
- Understanding of Regulations: Familiarity with FDA regulations (e.g., 21 CFR Part 820), EU MDR, and other relevant regulatory landscapes is crucial.
- Material Expertise and Handling:
- Certified Materials: Do they source and use certified medical-grade metal powders (e.g., 316L, Ti-6Al-4V ELI) with full traceability?
- Powder Quality Control: What are their processes for incoming powder inspection, storage, handling, and recycling/rejuvenation to prevent contamination and ensure consistency? Companies like Met3dp, which manufacture their own high-quality powders using advanced atomization techniques, offer significant advantages in material control and traceability. You can learn more about our commitment to quality on our Over ons pagina.
- Range of Materials: Capability to work with the specific alloys required (316L, Ti-6Al-4V) is essential.
- Technology and Equipment:
- Appropriate AM Technology: Do they operate well-maintained, industrial-grade metal AM systems (e.g., LPBF, SEBM) suitable for the required precision and material?
- Build Volume and Capacity: Can they accommodate the size and quantity of parts required?
- Process Monitoring: Do their machines incorporate in-situ monitoring capabilities for enhanced quality control?
- Post-Processing Capabilities:
- In-House vs. Outsourced: Do they offer the necessary post-processing steps (heat treatment, support removal, machining, polishing, cleaning, passivation) in-house or through qualified partners? In-house capabilities often provide better control and potentially faster lead times.
- Validated Processes: Are their post-processing steps, particularly cleaning and passivation, validated for medical applications?
- Technical Expertise and Support:
- DfAM Support: Can they provide expert guidance on optimizing designs for additive manufacturing?
- Engineering Collaboration: Are their engineers accessible and collaborative throughout the project lifecycle?
- Problem-Solving: Do they have the expertise to troubleshoot potential manufacturing challenges?
- Inspection and Metrology:
- Uitrusting: Do they possess calibrated CMMs, profilometers, scanners, and other necessary metrology equipment?
- Reporting: Can they provide comprehensive inspection reports documenting dimensional accuracy, surface finish, and compliance with specifications?
- Confidentiality and IP Protection:
- NDAs: Are they willing and accustomed to working under Non-Disclosure Agreements to protect sensitive intellectual property?
Table: Supplier Evaluation Checklist
Criteria | Key Questions to Ask | Why It Matters for Surgical Tools |
---|---|---|
ISO 13485 Certification | Is the supplier certified? Can they provide the certificate? | Demonstrates medical device QMS compliance |
Medical Experience | What specific medical parts have you manufactured? Can you share case studies/examples? | Ensures understanding of medical requirements |
Material Control | How do you source, test, handle, and trace medical-grade powders (316L, Ti-6Al-4V)? | Guarantees material biocompatibility & properties |
Technology & Capacity | What AM machines do you use? What is the build volume/accuracy? Do you have capacity for my project? | Ensures technical capability & timely delivery |
Nabewerking | What steps are done in-house? Are cleaning/passivation processes validated? | Critical for final part properties, safety, finish |
Technische ondersteuning | Do you offer DfAM review? How do you handle technical challenges? | Optimizes design, ensures smooth production |
Inspection & QC | What metrology equipment do you have? What level of inspection reporting is standard/available? | Verifies parts meet critical specifications |
Confidentiality | What procedures are in place to protect intellectual property? | Protects sensitive design information |
Export to Sheets
Choosing a partner like Met3dp, with decades of collective expertise spanning advanced metal powder production, precision SEBM printing technology, and comprehensive application development services, provides a strong foundation for developing and manufacturing high-quality, reliable 3D printed surgical tools.
Understanding Cost Structures and Lead Times for 3D Printed Surgical Tools
While metal AM enables innovation, understanding the associated costs and production timelines is essential for project planning, budgeting, and procurement. Unlike traditional mass production where tooling amortization dominates, AM costs are driven by different factors.
Key Cost Drivers for 3D Printed Surgical Tools:
- Material Type and Consumption:
- Material Choice: Titanium alloys (Ti-6Al-4V) are significantly more expensive than stainless steels (316L), both in terms of raw powder cost and often processing requirements.
- Part Volume: The actual volume of material used in the part itself. Larger/denser parts cost more.
- Ondersteunende structuren: Material used for supports adds to consumption and requires removal time/cost. Optimized orientation and DfAM can minimize this.
- Powder Waste/Recycling: Inefficient powder handling or excessive waste increases costs.
- Machine Time (Build Time):
- Part Height (Z-Height): Build time is primarily determined by the number of layers, meaning taller parts take longer, regardless of how many fit on the plate. Nesting multiple parts efficiently across the build plate is key for reducing per-part machine time cost.
- Deel Complexiteit: Very intricate features might require slower printing parameters.
- Machine Hourly Rate: Varies depending on the machine technology, size, and operator costs.
- Complex ontwerp:
- DfAM Optimization: Well-optimized designs minimize support needs and potentially print time. Poorly designed parts may require excessive supports or complex orientations, increasing cost.
- Internal Features: Complex internal channels requiring difficult powder removal can add to post-processing time and cost.
- Vereisten voor nabewerking:
- Intensity: The number and complexity of steps significantly impact cost. Basic support removal and blasting are less expensive than multi-axis CNC machining, electropolishing, and extensive manual finishing.
- Tolerance/Surface Finish: Tighter tolerances and finer surface finishes require more intensive (and costly) post-processing.
- Quality Assurance and Inspection:
- Level of Inspection: Basic dimensional checks are less costly than full CMM reports, material testing (if required per batch), and detailed surface analysis.
- Documentation: Extensive documentation required for regulatory compliance adds overhead.
- Bestelhoeveelheid:
- Setup Costs: While there’s no tooling, there are setup costs for preparing the build file, machine setup, and post-processing workflows. These costs are amortized over larger quantities, reducing the per-part price.
- Build Plate Efficiency: Higher quantities allow for more efficient nesting of parts on the build plate, reducing machine time per part.
Table: Summary of Cost Factors
Cost Driver | Primary Influence | How to Optimize |
---|---|---|
Materiaal | Alloy type (Ti vs. Steel), Part volume, Support volume | Choose appropriate material, DfAM for lightweighting |
Machine Time | Part height (Z), Build plate nesting efficiency | Optimize orientation, Maximize build plate utilization |
Complex ontwerp | Support needs, Internal features | Apply DfAM principles, Simplify where possible |
Nabewerking | Number/type of steps, Tolerance/Finish requirements | Specify only necessary steps, Design for easier finishing |
Kwaliteit | Inspection level, Documentation needs | Define clear, appropriate QC requirements |
Hoeveelheid | Amortization of setup costs, Nesting efficiency | Order in batches where feasible |
Export to Sheets
Doorlooptijden:
- Prototypen: For single pieces or very small batches with standard post-processing, lead times can be relatively short, often ranging from 5 to 15 business days, depending on complexity and supplier backlog.
- Production (Low-to-Medium Volume): For validated production parts requiring full post-processing and quality assurance, lead times are typically longer, ranging from 3 to 8 weeks. This accounts for scheduling, build time (which can be several days for a full plate), heat treatment cycles, multi-step post-processing, and thorough inspection.
- Factors Influencing Lead Time: Complexity, quantity, post-processing intensity, material availability, supplier capacity, and required validation/documentation all impact the final delivery schedule.
Clear communication with the AM provider regarding requirements and expectations is key to obtaining accurate cost estimates and realistic lead time projections.
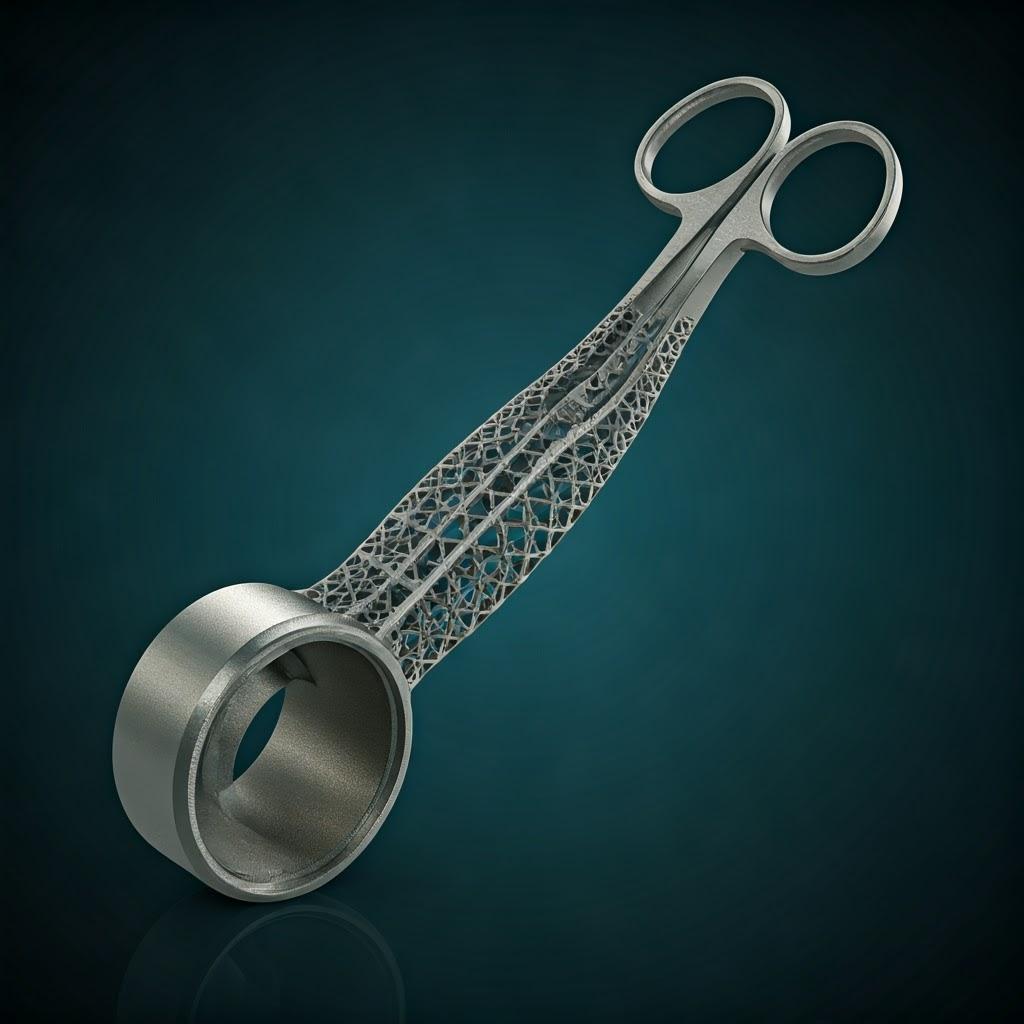
Frequently Asked Questions (FAQ) about 3D Printed Surgical Tools
Here are answers to some common questions regarding the use of 316L stainless steel and Ti-6Al-4V titanium for 3D printed surgical instruments:
- Are 3D printed surgical tools made from 316L and Ti-6Al-4V truly biocompatible? Yes, provided they are manufactured correctly. Both 316L stainless steel and Ti-6Al-4V (especially ELI grade) are widely recognized and used biocompatible materials conforming to standards like ISO 10993. However, biocompatibility depends not just on the raw material but critically on the entire process: using certified medical-grade powder, achieving high density (>99.5%), ensuring complete powder removal, utilizing appropriate post-processing (like passivation), and implementing validated cleaning protocols. Partnering with an ISO 13485 certified provider experienced in medical manufacturing is essential to ensure biocompatibility requirements are met.
- How does the strength and durability of 3D printed surgical tools compare to traditionally manufactured ones? The mechanical properties (strength, hardness, fatigue resistance) of metal AM parts made from 316L and Ti-6Al-4V can be comparable, and sometimes even superior, to those made by traditional methods like forging or casting, especially when considering wrought or annealed states. Proper heat treatment after printing is crucial to achieve optimal properties specified by ASTM or ISO standards. Furthermore, the design freedom of AM allows for optimizing geometries (e.g., using topology optimization) to enhance stiffness or strength where needed, potentially creating stronger or lighter tools than traditional counterparts.
- Can 3D printed stainless steel and titanium surgical tools be sterilized using standard hospital methods? Absolutely. Both 316L and Ti-6Al-4V exhibit excellent resistance to common sterilization methods used in healthcare facilities, including:
- Autoclaving (Steam Sterilization): The most common method; these materials withstand the high temperatures and pressures involved.
- Ethylene Oxide (EtO) Sterilization: Compatible.
- Gamma Radiation: Compatible.
- Low-Temperature Hydrogen Peroxide Gas Plasma: Compatible. The key considerations are that the instrument’s design must allow sterilant penetration and contact with all surfaces (DfAM for cleanability), and the parts must be thoroughly cleaned before sterilization to remove any bioburden or manufacturing residues.
- What information do I need to provide to get a quote for a 3D printed surgical tool? To receive an accurate quote, you typically need to provide:
- 3D CAD-model: A high-quality model in a standard format (e.g., STEP, IGES).
- Material Specification: Clearly state the desired material (e.g., 316L Stainless Steel or Ti-6Al-4V).
- Hoeveelheid: Number of identical parts required.
- Critical Tolerances: Indicate any specific dimensions requiring tighter tolerances than standard AM capabilities.
- Vereisten voor oppervlakteafwerking: Specify the desired surface roughness (Ra) or finish type (e.g., matte blasted, electropolished).
- Behoeften voor nabewerking: Detail any required heat treatment, machining, or specific finishing steps.
- Application Context: Briefly describe the intended use (helps the provider understand functional requirements).
- Required Certifications/Documentation: Specify any necessary material certs or inspection reports.
Conclusion: The Future of Surgery is Personalized and Precise with Metal AM
Metal additive manufacturing is rapidly evolving from a prototyping technology into a viable and often superior production method for complex, high-value components, particularly within the demanding field of surgery. The ability to use proven biocompatible materials like 316L stainless steel and Ti-6Al-4V titanium, combined with the unparalleled design freedom offered by AM, empowers medical device engineers and surgeons to create instruments previously thought impossible.
From patient-specific surgical guides enhancing procedural accuracy to lightweight, ergonomic tools reducing surgeon fatigue, and consolidated instruments simplifying complex procedures, metal 3D printing delivers tangible benefits. It enables faster innovation cycles, facilitates mass customization, and opens new avenues for improving surgical techniques and patient outcomes.
However, realizing these benefits requires careful attention to Design for Additive Manufacturing principles, stringent process control, validated post-processing techniques, and rigorous quality assurance. The selection of high-quality metal powders and a knowledgeable, experienced manufacturing partner with appropriate medical certifications (like ISO 13485) is paramount to success.
As the technology continues to mature and costs potentially decrease, the adoption of 3D printed surgical tools is set to accelerate, further personalizing surgery and driving advancements across medical specialties.
Ready to explore how metal additive manufacturing can revolutionize your surgical instrument designs? Leverage the expertise and cutting-edge capabilities of Met3dp. As a leader in both advanced metal powder production and precision metaal 3D printoplossingen, we provide comprehensive support from design optimization to final part production. Contact Met3dp today to discuss your project requirements and discover how our capabilities can power your organization’s additive manufacturing goals.
Delen op
Facebook
Twitteren
LinkedIn
WhatsAppen
E-mail
MET3DP Technology Co, LTD is een toonaangevende leverancier van additieve productieoplossingen met hoofdkantoor in Qingdao, China. Ons bedrijf is gespecialiseerd in 3D printapparatuur en hoogwaardige metaalpoeders voor industriële toepassingen.
Onderzoek om de beste prijs en een op maat gemaakte oplossing voor uw bedrijf te krijgen!
gerelateerde artikelen
Over Met3DP
Recente update
Ons product
NEEM CONTACT MET ONS OP
Nog vragen? Stuur ons nu een bericht! Na ontvangst van uw bericht behandelen wij uw verzoek met een heel team.
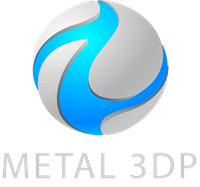
Metaalpoeders voor 3D printen en additieve productie
BEDRIJF
PRODUCT
contact informatie
- Qingdao-stad, Shandong, China
- [email protected]
- [email protected]
- +86 19116340731