Rocket Fuel Nozzle Tips 3D Printed in Superalloys
Оглавление
Introduction: Revolutionizing Rocket Propulsion with 3D Printed Fuel Nozzle Tips
The relentless pursuit of more efficient, powerful, and reliable space exploration hinges on continuous innovation in rocket engine technology. At the heart of these sophisticated propulsion systems lies a component seemingly small yet fundamentally critical: the fuel nozzle tip. This intricate piece dictates the precise mixing and injection of propellants into the combustion chamber, directly influencing engine performance, stability, and overall mission success. Traditionally manufactured through complex casting and machining processes, fuel nozzle tips present significant challenges due to their complex geometries, demanding material requirements, and the extreme operating conditions they endure – temperatures exceeding thousands of degrees Celsius, immense pressures, and exposure to highly corrosive propellants.
Enter metal additive manufacturing (AM), or 3D-печать. This transformative technology is rapidly reshaping the landscape of aerospace component production, offering unprecedented design freedom, accelerated development cycles, and the ability to create highly optimized parts that were previously impossible or prohibitively expensive to manufacture. For rocket fuel nozzle tips, metal AM, particularly using high-performance nickel-based superalloys like Inconel 718 (IN718) and Inconel 625 (IN625), unlocks a new realm of possibilities. It enables the creation of integrated cooling channels, complex internal features for enhanced mixing, and consolidated designs that reduce part count and potential failure points.
The implications for aerospace engineering and procurement managers are profound. Metal AM offers a pathway to lighter, more efficient rocket engines, potentially reducing launch costs and enabling more ambitious missions. Furthermore, the digital nature of AM facilitates faster design iteration and on-demand production, streamlining supply chains and reducing reliance on traditional manufacturing bottlenecks. Companies seeking qualified aerospace component suppliers and reliable metal AM service providers are increasingly turning to additive manufacturing to gain a competitive edge.
Met3dp, headquartered in Qingdao, China, stands at the forefront of this technological revolution. As a leading provider of comprehensive решения для аддитивного производства металлов, Met3dp specializes in both advanced 3D printing equipment and the production of high-performance metal powders crucial for demanding applications like rocket fuel nozzle tips. Our deep expertise in materials science, particularly with superalloys like IN718 and IN625, coupled with our state-of-the-art gas atomization and Plasma Rotating Electrode Process (PREP) powder production technologies, ensures that engineers and procurement professionals receive components meeting the stringent requirements of the aerospace industry. This blog post delves into the specifics of utilizing metal 3D printing for rocket fuel nozzle tips, exploring the critical functions, material choices, design considerations, and manufacturing processes involved, highlighting how Met3dp empowers the next generation of space propulsion. We will explore why IN718 and IN625 are the materials of choice, sourced from expert superalloy powder manufacturers like Met3dp, and how AM overcomes the limitations of conventional methods.
The Critical Role of Fuel Nozzle Tips in Rocket Engine Performance
To fully appreciate the impact of additive manufacturing on rocket fuel nozzle tips, one must first understand their indispensable function within the complex ecosystem of a rocket engine. These components are far more than simple conduits for fuel; they are precision instruments operating under some of the most extreme conditions engineered by humankind. Their primary responsibilities include:
- Propellant Atomization: The nozzle tip must break down the incoming liquid fuel (and sometimes oxidizer, depending on the injector design) into a fine spray of minuscule droplets. This dramatically increases the surface area of the propellant, enabling rapid vaporization and efficient mixing with the oxidizer. Effective atomization is paramount for stable and complete combustion. Poor atomization leads to combustion instability, reduced efficiency, and potential engine damage.
- Precise Fuel Injection: The nozzle must deliver the atomized propellant into the combustion chamber at a specific flow rate, spray pattern (e.g., conical, fan), and angle. This precise delivery ensures optimal mixing dynamics with the oxidizer, leading to controlled combustion and maximizing thrust generation. Deviations can cause localized hot spots, incomplete burning, and thrust vector misalignments.
- Creating Specific Mixing Patterns: In many advanced injector designs (like pintle or coaxial swirl injectors), the fuel nozzle tips are integral parts of the injector assembly, working in concert with oxidizer passages to generate specific turbulent mixing patterns. These patterns enhance combustion efficiency and stability, particularly under varying thrust levels.
- Enduring Extreme Environments: This is arguably the most challenging aspect. Fuel nozzle tips are situated at the threshold of the combustion chamber, exposing them to:
- Экстремальные температуры: Often exceeding 3000∘C (5432∘F) in the combustion zone, although the nozzle material itself is kept cooler through design and regenerative cooling. Still, the material faces intense thermal gradients and high heat flux.
- Высокое давление: Combustion chambers operate at pressures ranging from tens to hundreds of atmospheres (bars). The nozzle tip must maintain structural integrity under these immense loads.
- Corrosive Propellants: Rocket fuels (like RP-1, methane, hydrogen) and oxidizers (like liquid oxygen) can be highly corrosive, especially at elevated temperatures.
- Vibration and Acoustics: The combustion process generates intense vibrations and acoustic energy that the nozzle tip must withstand without fatigue failure.
- Thermal Cycling: Engines undergo rapid heating during startup and cooling during shutdown, inducing thermal stresses.
The performance characteristics directly influenced by the fuel nozzle tip include:
- Combustion Efficiency (c∗): A measure of how effectively the chemical energy of the propellants is converted into thermal energy. Optimal atomization and mixing driven by the nozzle design are key.
- Thrust: The force generated by the engine. Efficient combustion translates directly to higher thrust for a given propellant flow rate.
- Specific Impulse (Isp): A measure of engine efficiency (thrust per unit of propellant consumed per second). Higher combustion efficiency generally leads to higher Isp.
- Combustion Stability: Smooth, predictable burning is crucial. Poor nozzle performance can lead to instabilities like “chugging” or high-frequency “screaming” combustion, which can destroy an engine.
- Engine Durability and Life: Nozzle designs that mitigate thermal stresses and resist erosion/corrosion contribute to longer engine life and reusability.
Given these critical functions and harsh operating conditions, the design and manufacture of fuel nozzle tips demand exceptional precision and the use of materials capable of retaining their strength and integrity at extreme temperatures. Any compromise in quality or performance can have catastrophic consequences for the engine and the mission. This underscores the importance of selecting appropriate материалы аэрокосмического класса and partnering with experienced aerospace component manufacturers who understand the nuances of producing these mission-critical parts. Met3dp’s commitment to producing high-purity, spherical metal powders using advanced techniques like gas atomization ensures the foundational material quality required for such demanding applications.
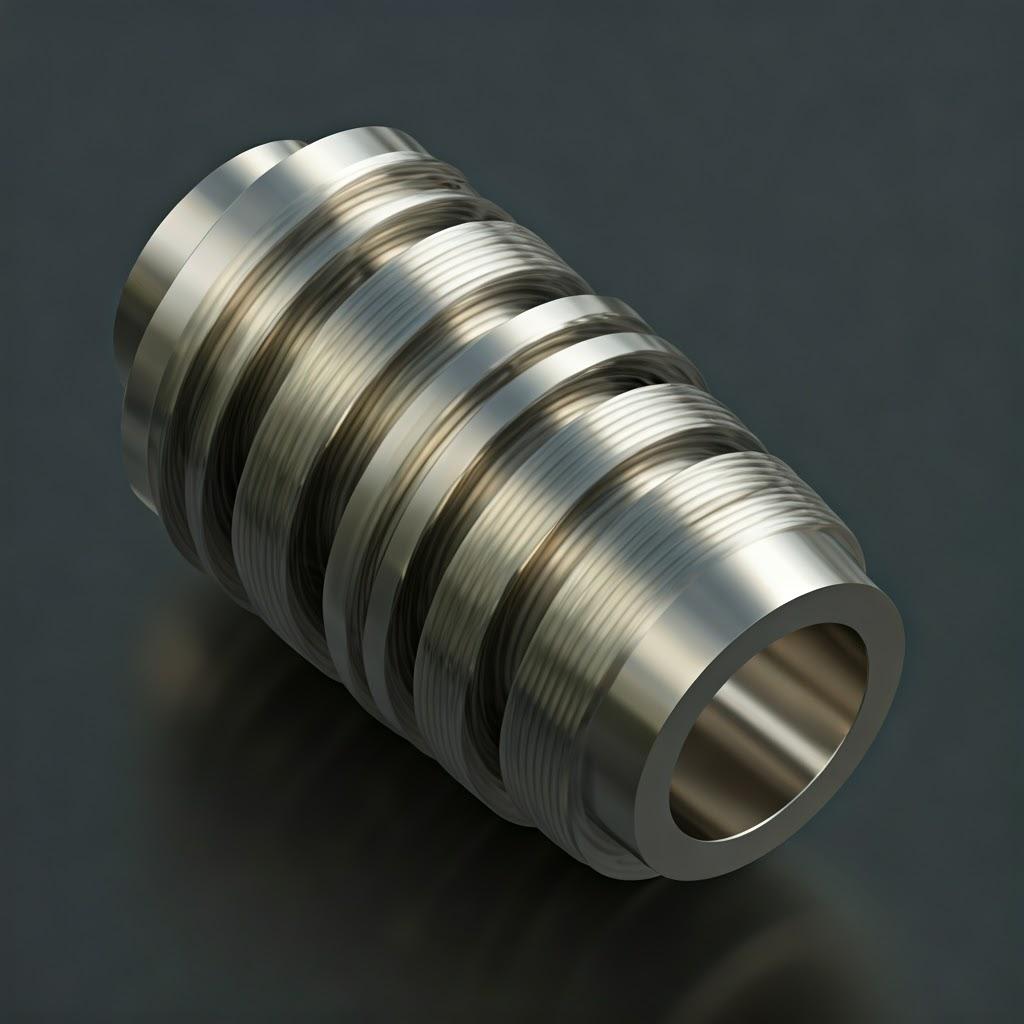
Why Metal Additive Manufacturing is Ideal for Complex Rocket Fuel Nozzles
Traditional manufacturing methods for rocket fuel nozzle tips, such as multi-axis CNC machining, electrical discharge machining (EDM), and investment casting, have served the industry for decades. However, they inherently possess limitations, particularly when engineers strive for designs that maximize performance and efficiency. Additive manufacturing fundamentally overcomes many of these constraints, making it an increasingly preferred method for producing advanced fuel nozzles.
Limitations of Traditional Manufacturing:
- Geometric Constraints: Machining struggles with creating complex internal features like curved cooling channels, intricate mixing geometries, or hollow structures. Investment casting allows more complexity but often requires multi-part assemblies and can have limitations in feature resolution and wall thickness.
- Part Consolidation Challenges: Complex nozzles often need to be fabricated in multiple pieces and then joined (e.g., brazing, welding). These joints introduce potential weak points, increase weight, add manufacturing steps, and complicate quality control.
- Отходы материалов: Subtractive processes like machining start with a larger block of expensive superalloy material and remove significant portions, leading to high “buy-to-fly” ratios and increased material cost.
- Long Lead Times & Tooling Costs: Creating tooling for casting or complex fixtures for machining can be time-consuming and expensive, especially for low-volume production or prototyping. Design iterations become slow and costly.
- Design Iteration Difficulty: Modifying a design often requires significant changes to tooling or machining programs, hindering rapid optimization cycles.
Advantages of Metal Additive Manufacturing (AM) for Fuel Nozzles:
Metal AM, particularly Laser Powder Bed Fusion (L-PBF), builds parts layer by layer directly from a digital model using high-quality metal powders. This approach offers compelling advantages for rocket fuel nozzle production:
- Беспрецедентная свобода дизайна:
- Complex Internal Geometries: AM excels at creating intricate internal cooling channels that conform precisely to the nozzle’s outer surfaces (conformal cooling). This allows for highly efficient heat removal, enabling higher combustion temperatures or longer nozzle life.
- Optimized Mixing Features: Engineers can design sophisticated internal structures to enhance propellant atomization and mixing far beyond what’s achievable with traditional methods.
- Облегчение: Topology optimization and lattice structures can be integrated into the design to reduce mass without compromising structural integrity – crucial for improving payload capacity.
- Консолидация частей: AM enables the printing of what were previously multi-part assemblies as a single, monolithic component. This eliminates joints, reduces weight, simplifies assembly, shortens the supply chain, and improves overall reliability by removing potential failure points. A single, integrated nozzle tip is inherently more robust.
- Accelerated Development & Prototyping: Designs can be iterated rapidly. A modified CAD model can be sent directly to the printer, allowing engineers to test multiple design variations much faster and at a lower cost than traditional prototyping, significantly speeding up the engine development cycle.
- Эффективность материала: AM is an additive process, using only the material necessary to build the part (plus supports). This drastically reduces material waste compared to subtractive methods, especially important when working with expensive superalloys like IN718 and IN625. Lower buy-to-fly ratios are a significant cost benefit.
- Повышенная производительность: The ability to implement optimized cooling and mixing features directly translates to tangible performance gains: higher combustion efficiency, improved thermal management, greater stability, and potentially longer component life.
Comparison Table: AM vs. Traditional Manufacturing for Fuel Nozzles
Характеристика | Metal Additive Manufacturing (L-PBF) | Traditional Manufacturing (Machining/Casting) |
---|---|---|
Сложность конструкции | Very High (complex internal channels, lattices, topology opt.) | Moderate to High (limited by tooling/access) |
Консолидация частей | Excellent (monolithic parts possible) | Limited (often requires assembly/joining) |
Материальные отходы | Low (near-net shape) | High (subtractive machining) / Moderate (casting runners) |
Lead Time (Prototype) | Fast (days/weeks) | Slow (weeks/months, requires tooling/fixtures) |
Стоимость оснастки | Никто | High (casting molds) / Moderate (machining fixtures) |
Small Batch Cost | Экономичный | High (due to setup/tooling amortization) |
Cooling Channels | Highly optimized, conformal channels easily integrated | Difficult/impossible to create complex internal channels |
Облегчение | Excellent potential via topology optimization & lattices | Limited potential |
Development Cycle | Rapid iteration possible | Slower iteration due to tooling/programming changes |
Export to Sheets
Partnering with a knowledgeable metal AM service provider like Met3dp ensures access to not only the printing technology but also the crucial expertise in process optimization and material science needed to fully leverage these advantages. Met3dp’s experience with aerospace applications and its focus on producing high-quality, AM-optimized superalloy powders make it an ideal partner for companies looking to procure advanced, 3D-printed rocket fuel nozzle tips.
IN718 & IN625 Superalloys: The Premier Material Choices for Rocket Nozzle Tips
The selection of material for a rocket fuel nozzle tip is non-negotiable; it must withstand the brutal operating environment while maintaining dimensional stability and structural integrity. Nickel-based superalloys have emerged as the gold standard for these demanding high-temperature applications, with Inconel 718 (IN718) and Inconel 625 (IN625) being two of the most widely adopted choices, particularly for additive manufacturing. Their suitability stems from an exceptional combination of properties:
- High Temperature Strength: They retain significant strength and resist deformation (creep) at temperatures where many other metals would weaken considerably. This is crucial for preventing nozzle distortion under thermal and pressure loads.
- Отличная коррозионная стойкость: They exhibit outstanding resistance to oxidation and corrosion caused by hot combustion gases and aggressive propellants over a wide temperature range.
- Good Fatigue Life: Rocket engines undergo cyclical loading (thermal and mechanical), making high fatigue strength essential for durability and reusability.
- Weldability/Printability: Both IN718 and IN625 generally exhibit good characteristics for additive manufacturing processes like L-PBF, although process parameters must be carefully controlled to manage residual stresses and avoid defects.
Let’s examine each alloy in more detail:
Inconel 718 (IN718 / Alloy 718)
- Состав: Primarily Nickel-Chromium, with significant additions of Iron, Niobium, and Molybdenum, plus smaller amounts of Aluminum and Titanium.
- Strengthening Mechanism: Precipitation hardening. It gains its exceptional strength through heat treatment processes (solution annealing followed by double aging) that precipitate strengthening phases (primarily Gamma Double Prime – γ′′) within the metal matrix.
- Основные свойства:
- Excellent mechanical strength (tensile, yield, creep-rupture) up to around 700∘C (1300∘F).
- Good corrosion and oxidation resistance.
- Relatively good weldability/printability for a precipitation-hardenable superalloy due to its slower aging kinetics.
- Widely used and well-characterized in the aerospace industry (often considered the workhorse).
- AM Considerations: Requires careful control of L-PBF parameters (laser power, scan speed, layer thickness) and post-build heat treatments (stress relief, solutionizing, aging) to achieve optimal microstructure and mechanical properties, comparable to wrought counterparts. Sourcing high-quality, pre-alloyed IN718 powder with consistent spherical morphology and low oxygen content, like those produced by Met3dp’s advanced gas atomization systems, is critical for successful printing.
Inconel 625 (IN625 / Alloy 625)
- Состав: Primarily Nickel-Chromium, with significant additions of Molybdenum and Niobium. Lower Iron content than IN718.
- Strengthening Mechanism: Solid-solution strengthening. Molybdenum and Niobium atoms dissolve into the Nickel-Chromium matrix, distorting the crystal lattice and impeding dislocation movement, which provides strength. It does not require precipitation hardening heat treatments for strengthening, although annealing is used.
- Основные свойства:
- Excellent fabricability and weldability/printability.
- Outstanding resistance to a wide range of corrosive environments, both oxidizing and reducing, often superior to IN718 in certain aggressive media (e.g., pitting, crevice corrosion).
- Very good strength and toughness from cryogenic temperatures up to around 815∘C (1500∘F), maintaining strength at slightly higher temperatures than IN718, though its peak strength might be lower than fully heat-treated IN718 at moderate temperatures.
- Excellent fatigue strength.
- AM Considerations: Generally considered easier to print than IN718 due to its solid-solution nature, resulting in lower residual stresses and reduced susceptibility to cracking during the build. Post-build stress relief or annealing is typically required. Again, consistent, high-purity IN625 powder is essential. Met3dp’s expertise extends to producing specialized powders like IN625, ensuring aerospace material suppliers and end-users receive powder optimized for additive processes.
Material Selection Comparison: IN718 vs. IN625 for Fuel Nozzles
Недвижимость | IN718 (Heat Treated) | IN625 (Annealed) | Considerations for Fuel Nozzles |
---|---|---|---|
Max Operating Temp. | Good strength up to ~ 700∘C (1300∘F) | Good strength up to ~ 815∘C (1500∘F) | IN625 may offer a slight edge if peak material temps are higher, but cooling design is key. |
Strength (Moderate Temp) | Generally Higher (due to γ′′ precipitation) | Lower than peak IN718 | IN718 often preferred for max strength if temps allow. |
Коррозионная стойкость | Очень хорошо | Excellent (often superior, esp. pitting/crevice) | Depends on specific propellant chemistry; IN625 might be better for highly corrosive mixtures. |
Возможность печати | Good (requires careful control & heat treat) | Excellent (less prone to cracking, simpler heat treat) | IN625 can simplify the AM process and reduce risk, potentially lowering производственные затраты. |
Термообработка | Complex (Stress Relief + Solution + Double Age) | Simpler (Stress Relief / Anneal) | IN718 requires more extensive post-processing. |
Industry Database | Extensive (workhorse alloy) | Обширный | Both are well-understood materials in aerospace. |
Стоимость | Generally comparable, can vary based on market & form | Generally comparable, can vary based on market & form | Powder quality and consistency are major factors. |
Export to Sheets
The Met3dp Advantage in Superalloy Powders:
Choosing the right alloy is only part of the equation. The quality of the metal powder used in the L-PBF process is paramount to achieving the desired material properties and defect-free components. This is where Met3dp’s capabilities become crucial for procurement managers and engineers sourcing materials for bulk additive manufacturing or critical components.
- Передовое производство порошков: Met3dp utilizes industry-leading Gas Atomization (GA) and Plasma Rotating Electrode Process (PREP) technologies. Our GA equipment features unique nozzle and gas flow designs optimized to produce powders with:
- Высокая сферичность: Ensures excellent powder bed density and flowability within the AM machine, leading to more uniform layers and predictable melting.
- Low Porosity: Minimizes internal voids within powder particles, reducing potential defects in the final part.
- Controlled Particle Size Distribution (PSD): Tailored PSD for specific AM processes ensures optimal packing and melting behavior.
- Высокая чистота: Minimizing contaminants (like Oxygen and Nitrogen) is critical for achieving the desired mechanical properties and corrosion resistance in superalloys.
- Контроль качества: Rigorous testing and characterization ensure batch-to-batch consistency, a vital requirement for aerospace applications where process repeatability is key.
- Портфель материалов: Beyond IN718 and IN625, Met3dp offers a wide range of high-performance powders, including Titanium alloys (Ti6Al4V, TiNi, TiTa, TiAl, TiNbZr), CoCrMo, stainless steels, and other superalloys, positioning us as a comprehensive поставщик металлического порошка for diverse industrial needs. Explore Met3dp’s range of high-performance metal powders.
By leveraging AM with high-quality IN718 or IN625 powders from a trusted supplier like Met3dp, aerospace companies can produce rocket fuel nozzle tips with superior performance, enhanced reliability, and potentially lower overall system costs, driving the future of space propulsion technology.
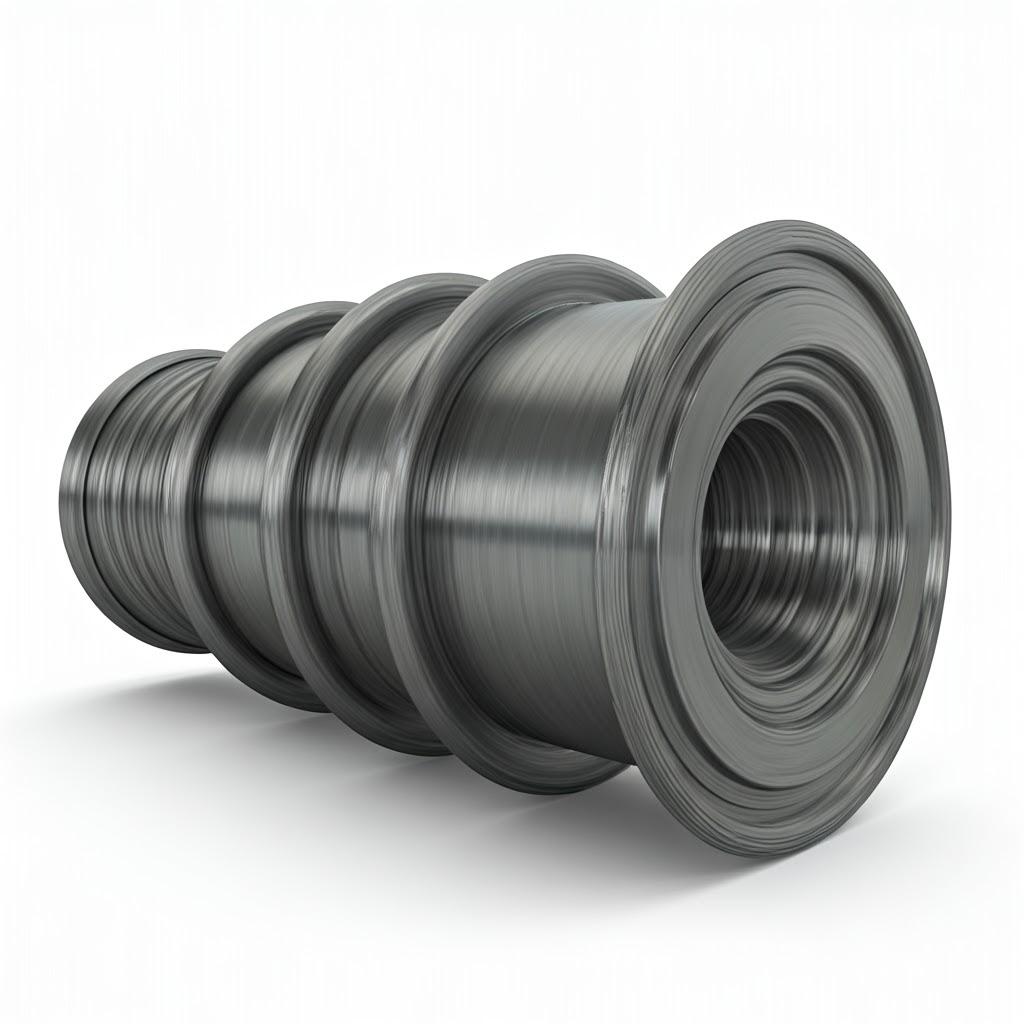
Design for Additive Manufacturing (DfAM) Strategies for Optimized Fuel Nozzle Performance
Simply replicating a design originally intended for traditional manufacturing using additive methods often fails to capture the true potential of 3D printing. To fully harness the benefits outlined previously – complex geometries, part consolidation, lightweighting, and enhanced performance – engineers must embrace Design for Additive Manufacturing (DfAM). DfAM is not just a set of guidelines; it’s a paradigm shift in thinking about component design, considering the unique capabilities and constraints of the layer-by-layer AM process from the outset. For a component as intricate and critical as a rocket fuel nozzle tip, employing DfAM strategies is essential for maximizing performance, reliability, and manufacturability.
Effective DfAM for fuel nozzles involves several key strategies:
- Exploiting Geometric Freedom for Thermal Management (Conformal Cooling):
- Concept: Traditional cooling channels are often straight lines drilled into the component, leading to uneven cooling and potential hot spots. AM allows the creation of cooling channels that precisely follow the contours of the nozzle’s heated surfaces (conformal channels).
- Преимущества: This results in much more uniform and efficient heat extraction from critical areas, like the nozzle throat or face. Benefits include:
- Reduced peak material temperatures, increasing component life and safety margins.
- Lower thermal gradients, minimizing thermal stress and distortion.
- Potential to push engine performance limits (higher combustion temperatures/pressures).
- Reduced coolant flow requirements, saving weight elsewhere in the system.
- Implementation: DfAM involves designing channel paths, cross-sectional shapes (e.g., optimizing for heat transfer and pressure drop), and inlet/outlet manifolds directly into the CAD model, leveraging the capabilities of L-PBF to build these intricate internal networks.
- Optimizing Internal Flow Paths and Mixing Features:
- Atomization & Mixing: AM allows for the design of highly complex internal geometries within the nozzle orifice itself – features like optimized swirl generators, uniquely shaped outlets, or intricate passages designed to enhance propellant atomization and mixing efficiency far beyond what machining or casting could achieve.
- Smooth Passages & Thin Walls: Designers can create smooth, aerodynamically efficient internal passages to minimize pressure drops and ensure precise fuel delivery. AM also enables the creation of thin, yet structurally sound, walls where needed, contributing to lightweighting and optimal thermal performance. Wall thicknesses need careful consideration based on pressure loads, material properties, and AM process resolution.
- Leveraging Part Consolidation:
- Concept: As mentioned earlier, AM enables the integration of multiple components into a single, monolithic part. A traditionally fabricated fuel nozzle assembly might consist of a body, internal swirlers, retention nuts, and sealing elements, all requiring complex assembly and joining.
- DfAM Approach: Engineers can redesign the entire assembly as a single printable unit. This involves integrating mounting features, internal flow elements, and even parts of the injector face into one piece.
- Преимущества: Eliminates joints (potential leak paths and failure points), significantly reduces assembly time and labor, lowers overall part count simplifying procurement and supply chain management, and often reduces weight.
- Strategic Design for Support Structures:
- Necessity: L-PBF typically requires support structures for overhanging features (usually below 45 degrees from the horizontal) and to anchor the part to the build plate, managing thermal stresses.
- DfAM Considerations:
- Minimization: Orienting the part on the build plate to minimize the need for supports (self-supporting angles). Designing features with chamfers or fillets instead of sharp overhangs.
- Доступность: Ensuring supports, especially internal ones (e.g., inside cooling channels), are accessible for removal post-printing. This might involve designing specific access ports that are later sealed or considering the limitations of removal tools.
- Support Type: Designing supports that are strong enough to prevent warpage but optimized for easier removal (e.g., conical supports, perforated structures).
- Влияние: Support structures add print time, consume material, and necessitate post-processing steps for removal and surface finishing. Effective DfAM minimizes these impacts.
- Implementing Topology Optimization and Lattice Structures:
- Topology Optimization: Using software algorithms to remove material from areas where it is not structurally required, based on defined load cases and constraints. This can significantly reduce the weight of mounting flanges or non-critical structural elements of the nozzle assembly, crucial for improving the overall thrust-to-weight ratio of the engine.
- Решетчатые структуры: Replacing solid volumes with internal lattice or cellular structures can further reduce weight while maintaining required stiffness or facilitating fluid flow or heat dissipation. These complex structures are only feasible through AM.
- Considering Build Orientation:
- Влияние: The orientation in which the nozzle is printed affects surface finish (stair-stepping effect on curved surfaces), the location and amount of support structures needed, residual stress distribution, build time, and potentially mechanical properties due to the anisotropic nature of AM processes.
- DfAM Strategy: Selecting the optimal build orientation is a critical DfAM step, balancing factors like surface quality requirements for specific features (e.g., sealing surfaces), minimizing internal supports, managing thermal stresses, and aligning critical features with the best resolution axes of the printer.
Successfully implementing DfAM requires close collaboration between design engineers, materials scientists, and AM process specialists. Companies like Met3dp, with deep expertise in both современные металлические порошки and additive manufacturing processes, can provide invaluable input during the design phase, ensuring that the fuel nozzle design is fully optimized for printability, performance, and reliability. Engaging with an experienced aerospace AM partner early is key to leveraging DfAM effectively.
Achieving Aerospace-Grade Precision: Tolerance, Surface Finish, and Dimensional Accuracy in AM Nozzles
Rocket fuel nozzle tips are precision components where minute deviations in dimensions or surface characteristics can significantly impact engine performance and safety. Achieving the required level of precision with additive manufacturing, particularly using superalloys like IN718 and IN625, demands careful process control, understanding of material behavior, and often, supplementary post-processing steps. Engineers and procurement managers must have realistic expectations about the capabilities of L-PBF and plan accordingly.
Dimensional Accuracy and Tolerances:
- As-Built Tolerances: The dimensional accuracy achievable directly from the L-PBF process (as-built) typically falls within the range of ±0.1 mm to ±0.3 mm (±0.004” to ±0.012”) for general dimensions on well-controlled industrial machines. Smaller features or tighter tolerances might be achievable but often depend heavily on feature geometry, location, orientation, and rigorous process optimization.
- Factors Influencing Accuracy:
- Machine Calibration: Precision of the laser scanning system, layer thickness control, and overall machine stability are fundamental. Met3dp utilizes industry-leading equipment known for accuracy and reliability.
- Process Parameters: Laser power, scan speed, hatch spacing, and layer thickness directly influence melt pool size and stability, affecting dimensional control.
- Thermal Effects: The rapid heating and cooling inherent in L-PBF induce thermal stresses, which can lead to warpage and distortion if not properly managed through support strategies and optimized scan patterns. Superalloys with high thermal expansion coefficients require careful management.
- Качество порошка: Consistent particle size distribution, morphology (sphericity), and flowability of the metal powder (like Met3dp’s gas atomized IN718/IN625) contribute to uniform powder bed density and predictable melting, enhancing dimensional consistency.
- Part Size and Geometry: Larger parts or parts with significant variations in cross-section can be more prone to thermal distortion.
- Support Strategy: Supports play a crucial role in anchoring the part and managing heat dissipation, influencing final dimensions.
- Post-Machining for Critical Tolerances: For features requiring tolerances tighter than what L-PBF can reliably achieve as-built (e.g., sealing surfaces, interfacing diameters, critical orifice dimensions often requiring ±0.01 mm to ±0.05 mm or better), post-process CNC machining is typically employed. DfAM principles should account for adding stock material in these critical areas for subsequent machining operations.
Surface Finish (Roughness):
- As-Built Surface Finish: The surface finish, typically measured as average roughness (Ra), of as-built L-PBF parts is influenced by several factors:
- Толщина слоя: Thinner layers generally result in smoother surfaces.
- Размер частиц: Finer powders can contribute to smoother finishes but may affect flowability.
- Orientation:
- Vertical Walls: Tend to have relatively good finishes, influenced by the powder particles partially adhering to the sides.
- Up-facing Surfaces: Generally the smoothest, directly shaped by the laser melting. Typical Ra values might be 5-15 µm.
- Down-facing (Overhanging) Surfaces: Tend to be the roughest due to the necessary contact with support structures or the ‘stair-stepping’ effect on shallow angles. Ra values can exceed 15-20 µm or more.
- Process Parameters: Laser parameters and scan strategies affect melt pool dynamics and surface texture.
- Impact on Performance: Surface roughness inside the nozzle can affect fluid dynamics (pressure drop, flow separation) and heat transfer. Rough external surfaces might be less critical unless they are sealing interfaces.
- Улучшение качества поверхности: When the as-built finish is insufficient, various post-processing techniques are used (detailed in the next section), including machining, polishing, abrasive flow machining (AFM), or electrochemical polishing (ECP), especially for internal channels where smooth flow is critical.
Metrology and Quality Assurance:
Ensuring that the final fuel nozzle meets the stringent aerospace tolerance requirements necessitates advanced metrology. Coordinate Measuring Machines (CMMs) are used for precise dimensional checks, while optical scanners and CT scanning can provide comprehensive 3D comparisons to the original CAD model, including verification of internal channel dimensions and detection of defects. Partnering with an AM provider like Met3dp, which emphasizes process control and utilizes high-quality materials and equipment, provides a strong foundation for achieving aerospace-grade precision. Understanding the nuances of different способы печати and their achievable precision is vital for setting realistic expectations.
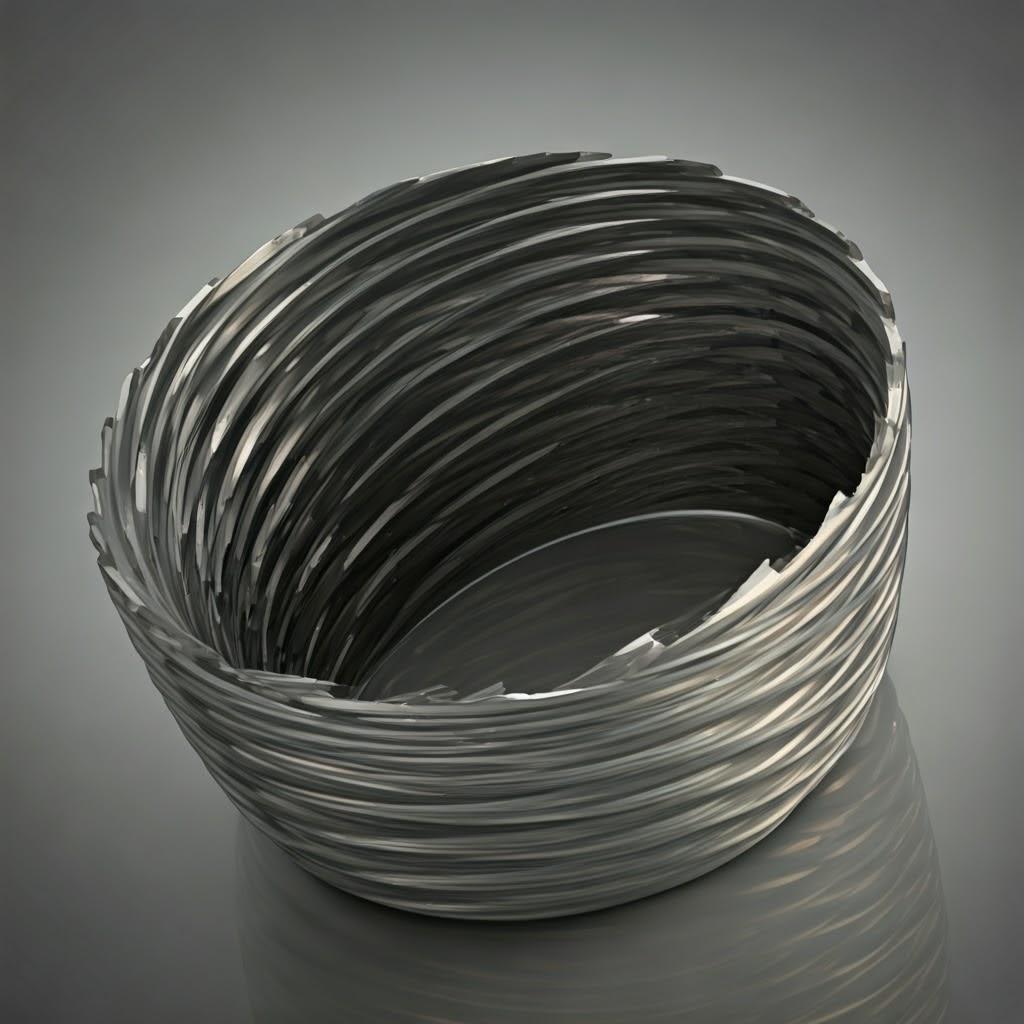
Essential Post-Processing Steps for 3D Printed Rocket Fuel Nozzle Tips
Creating a fuel nozzle tip via Laser Powder Bed Fusion is a sophisticated process, but the journey from digital file to flight-ready hardware rarely ends when the printer stops. For mission-critical components made from superalloys like IN718 and IN625, a sequence of carefully controlled post-processing steps is essential to relieve stress, achieve the required material properties, ensure dimensional accuracy, remove supports, refine surfaces, and verify integrity. These steps are often complex, time-consuming, and require specialized equipment and expertise, significantly contributing to the overall cost and lead time of the finished part. Procurement managers must factor these requirements into project planning and supplier evaluation.
Key post-processing stages typically include:
- Снятие стресса:
- Цель: The rapid, localized heating and cooling during L-PBF create significant residual stresses within the printed part. These stresses can cause distortion or cracking when the part is removed from the build plate or during subsequent processing. Stress relief heat treatment is performed before removing the part from the build plate.
- Процесс: The entire build plate with the attached part(s) is heated in a controlled atmosphere furnace (vacuum or inert gas like Argon to prevent oxidation) to a specific temperature below the aging or annealing temperature, held for a period, and then slowly cooled. Typical cycles for IN718/IN625 might involve heating to 850−1000∘C (1560−1830∘F).
- Importance: This is a mandatory first step for most superalloy AM parts to ensure dimensional stability and prevent subsequent failure.
- Снятие со строительной плиты:
- Method: Once stress-relieved, the part needs to be separated from the build plate. This is commonly done using Wire Electrical Discharge Machining (Wire EDM) or a band saw. Care must be taken to avoid damaging the part itself.
- Демонтаж опорной конструкции:
- Вызов: Supports, essential during the build, must now be removed. This can be labor-intensive, especially for complex geometries or internal supports.
- Методы: Depending on the design and accessibility, supports may be removed manually (breaking, cutting), using CNC machining, or potentially grinders. Internal supports within channels pose a significant challenge and might require advanced techniques like Abrasive Flow Machining (AFM) if access allows, or careful DfAM planning to minimize them. Incomplete or improper support removal can leave undesirable surface artifacts or stress concentrators.
- Heat Treatment (Solution Annealing & Aging / Annealing):
- Цель: To homogenize the microstructure developed during the rapid solidification of AM and to achieve the target mechanical properties (strength, ductility, hardness, creep resistance) specified for the alloy.
- Process for IN718 (Precipitation Hardening): Typically involves:
- Отжиг раствора: Heating to a high temperature (e.g., 950−1050∘C or 1740−1920∘F) to dissolve existing precipitates and homogenize the microstructure, followed by rapid cooling.
- Лечение старения: A two-step lower temperature heat treatment (e.g., 720∘C/1325∘F followed by 620∘C/1150∘F) to precipitate the strengthening γ′ and γ′′ phases. Precise time and temperature control is critical.
- Process for IN625 (Solid Solution Strengthened): Typically involves an Отжиг step (e.g., 870−1175∘C or 1600−2150∘F, specific temperature depends on desired properties) to relieve stress, recrystallize the grain structure, and ensure niobium is in solution, followed by controlled cooling. No aging step is required for strengthening.
- Atmosphere: All high-temperature heat treatments for superalloys must be performed in a vacuum or high-purity inert atmosphere to prevent oxidation, which can degrade material properties.
- Горячее изостатическое прессование (HIP):
- Цель: To eliminate internal microporosity (like gas porosity or small lack-of-fusion voids) that might remain after the AM process. This significantly improves fatigue life, ductility, fracture toughness, and overall material consistency, which is often mandatory for critical aerospace components (fracture-critical или mission-critical parts).
- Процесс: The part is subjected to high temperature (below melting point but often near solution annealing temperatures) and high inert gas pressure (typically Argon, at 100-200 MPa or 15,000-30,000 psi) simultaneously in a specialized HIP vessel. The pressure collapses internal voids, diffusion bonding the material across the void interface.
- Consideration: HIP can cause slight dimensional changes and may affect surface finish, sometimes requiring subsequent machining.
- Обработка:
- Цель: To achieve final tolerances on critical dimensions, create specific interface features (threads, sealing faces), or obtain very smooth surface finishes (e.g., Ra < 1 µm) that are unattainable by AM or other polishing methods alone.
- Процесс: Standard multi-axis CNC machining techniques are used, but machining superalloys requires appropriate tooling, speeds, and feeds due to their high strength and work-hardening characteristics.
- Отделка поверхности:
- Цель: To improve surface smoothness beyond the as-built or post-HIP state, particularly for internal channels or external surfaces requiring specific aerodynamic or sealing properties.
- Методы:
- Abrasive Blasting (Grit/Bead Blasting): Provides a uniform matte finish, removes minor imperfections.
- Tumbling/Vibratory Finishing: Uses media to smooth surfaces and deburr edges, suitable for batches of smaller parts.
- Abrasive Flow Machining (AFM): Forces abrasive putty through internal channels or across external surfaces to polish and radius edges. Effective for inaccessible internal passages.
- Electrochemical Polishing (ECP): Removes material electrochemically, resulting in a very smooth, bright finish. Can be adapted for internal channels.
- Manual Polishing: For achieving mirror finishes on accessible surfaces.
- Non-Destructive Testing (NDT) and Inspection:
- Цель: To verify the integrity of the final part and ensure it meets all specifications without damaging it. This is a critical step for aerospace quality assurance.
- Методы:
- Visual Inspection: Basic check for obvious flaws.
- Dimensional Metrology: CMM, 3D scanning.
- X-Ray Computed Tomography (CT Scanning): Essential for detecting internal defects (porosity, inclusions, cracks) and verifying internal geometry in complex AM parts.
- Fluorescent Penetrant Inspection (FPI): Detects surface-breaking cracks or porosity.
- Ultrasonic Testing (UT): Can detect subsurface flaws.
- Material Analysis: Verifying chemical composition and microstructure (often done destructively on test coupons printed alongside the part).
The specific sequence and combination of these post-processing steps depend heavily on the nozzle’s design complexity, the chosen alloy (IN718 vs. IN625), and the stringent requirements of the aerospace application. Effectively managing this complex workflow requires significant expertise and investment in specialized facilities, highlighting the value of partnering with experienced metal AM solution providers.
Overcoming Common Challenges in 3D Printing Superalloy Fuel Nozzles
While metal additive manufacturing offers tremendous advantages for producing rocket fuel nozzle tips, working with high-performance superalloys like IN718 and IN625 in a process involving rapid melting and solidification presents unique challenges. Understanding these potential issues and implementing mitigation strategies is crucial for consistently producing high-quality, reliable components. Aerospace engineers and procurement managers should be aware of these challenges when specifying AM parts and selecting suppliers.
1. Residual Stress and Warpage:
- Вызов: The intense, localized heat input from the laser and subsequent rapid cooling create steep thermal gradients, leading to internal stresses within the part. These stresses can cause the part to warp during the build, detach from supports, or distort after removal from the build plate. Superalloys, with their relatively high thermal expansion and strength at temperature, are particularly susceptible.
- Mitigation Strategies:
- Optimized Scan Strategies: Using techniques like dividing the layer into smaller segments (‘island’ or ‘checkerboard’ scanning) and varying the scan vector between layers helps distribute heat more evenly and reduce stress accumulation.
- Robust Support Structures: Well-designed supports anchor the part securely to the build plate and act as heat sinks, effectively managing thermal stresses. DfAM plays a key role here.
- Process Simulation: Thermal-mechanical simulation software can predict stress distribution and warpage, allowing for optimization of orientation and support strategies before printing.
- Parameter Optimization: Fine-tuning laser power, scan speed, and layer thickness can influence the thermal experience.
- Immediate Stress Relief: Performing the stress relief heat treatment immediately after the build, before removing the part from the plate, is critical.
2. Cracking (Solidification, Liquation, Strain Age):
- Вызов: Superalloys, especially precipitation-hardenable ones like IN718, can be susceptible to various forms of cracking during or after the AM process. Solidification cracking occurs in the melt pool, liquation cracking occurs in partially melted zones near the melt pool, and strain age cracking can occur during post-build heat treatment in IN718 if residual stresses are too high.
- Mitigation Strategies:
- Качество порошка: Using high-purity powder with low levels of crack-susceptible elements (like Sulfur, Phosphorus) is essential. Met3dp’s advanced powder manufacturing processes focus on achieving high purity and controlled chemistry.
- Parameter Optimization: Careful control over energy input (laser power, scan speed) to manage melt pool dynamics and cooling rates.
- Горячее изостатическое прессование (HIP): Can help close small cracks, although it’s primarily aimed at porosity.
- Appropriate Heat Treatment: Correct stress relief and aging cycles are crucial, especially for IN718, to avoid strain age cracking.
- Alloy Selection: IN625 is generally less prone to cracking during printing than IN718 due to its solid-solution strengthening mechanism.
3. Porosity (Gas and Lack-of-Fusion):
- Вызов: Small voids within the printed material can act as stress concentrators, degrading mechanical properties, particularly fatigue life.
- Gas Porosity: Caused by gas (e.g., Argon shield gas, dissolved gases in powder) becoming trapped in the rapidly solidifying melt pool.
- Lack-of-Fusion (LoF) Porosity: Caused by insufficient energy input, leading to incomplete melting and bonding between powder particles or layers.
- Mitigation Strategies:
- High-Quality Powder: Using powder with low internal gas content and good sphericity/flowability (like Met3dp’s) minimizes trapped gas and ensures a dense powder bed.
- Optimized Process Parameters: Ensuring sufficient energy density (laser power, scan speed, layer thickness) to achieve complete melting and fusion, without overheating which can increase gas porosity.
- Inert Atmosphere Control: Maintaining a high-purity inert gas atmosphere (Argon) in the build chamber minimizes contamination.
- Горячее изостатическое прессование (HIP): Highly effective at closing both gas and LoF porosity, often considered mandatory for critical applications.
4. Support Removal Difficulty:
- Вызов: Removing support structures, especially from complex internal channels common in fuel nozzles, can be difficult, time-consuming, and risk damaging the part. Residual support material can obstruct flow or act as initiation sites for failure.
- Mitigation Strategies:
- DfAM: Designing for minimal support use (self-supporting angles >45°), optimizing orientation, and designing access for removal tools.
- Specialized Removal Techniques: Utilizing CNC machining, EDM, AFM, or ECP where appropriate and feasible.
- Material Choice: Supports made from the same material can be challenging; research into dissimilar, easily removable support materials is ongoing but less mature for superalloys.
5. Anisotropy:
- Вызов: Due to the layer-by-layer build process and directional solidification, AM parts can exhibit anisotropic mechanical properties, meaning properties (like strength or ductility) can differ depending on the direction of testing relative to the build direction (X, Y, vs. Z). This must be accounted for in design and qualification.
- Mitigation Strategies:
- Характеристика: Thoroughly testing material properties in different orientations using representative coupons printed alongside parts.
- Design Consideration: Orienting the part so that the most critical stresses align with the strongest build direction.
- Heat Treatment & HIP: Can help homogenize the microstructure and reduce, but not always eliminate, anisotropy.
6. Surface Roughness:
- Вызов: As-built surfaces, particularly down-facing and steeply angled ones, can be rougher than required for fluid flow or sealing applications.
- Mitigation Strategies:
- Orientation Optimization: Prioritizing smooth surfaces on critical features during orientation selection.
- Optimized Parameters: Using specific ‘contour’ or ‘skin’ parameters for outer surfaces.
- Постобработка: Employing machining or various polishing techniques (AFM, ECP, tumbling, manual).
7. Quality Control and Process Consistency:
- Вызов: Ensuring that every part produced meets the stringent aerospace specifications requires robust quality control throughout the entire process, from powder management to final inspection. Maintaining consistency from build to build is paramount.
- Mitigation Strategies:
- Powder Control: Rigorous testing and handling of incoming powder batches. Met3dp provides certified powder quality.
- Process Monitoring: Utilizing in-situ monitoring tools (e.g., melt pool monitoring, thermal imaging) to detect anomalies during the build.
- Standardized Procedures: Implementing qualified manufacturing procedures under a robust Quality Management System (QMS), ideally certified to standards like AS9100 for aerospace.
- Comprehensive NDT: Employing appropriate NDT methods (especially CT scanning) for defect detection and geometric verification.
- Partnering with Experts: Working with experienced metal AM service providers like Met3dp, who possess deep process knowledge and a commitment to quality, is crucial for navigating these challenges effectively.
By proactively addressing these potential issues through careful design, high-quality materials, optimized process control, thorough post-processing, and rigorous inspection, the challenges of printing superalloy fuel nozzles can be successfully overcome, unlocking the full potential of additive manufacturing for next-generation rocket propulsion.
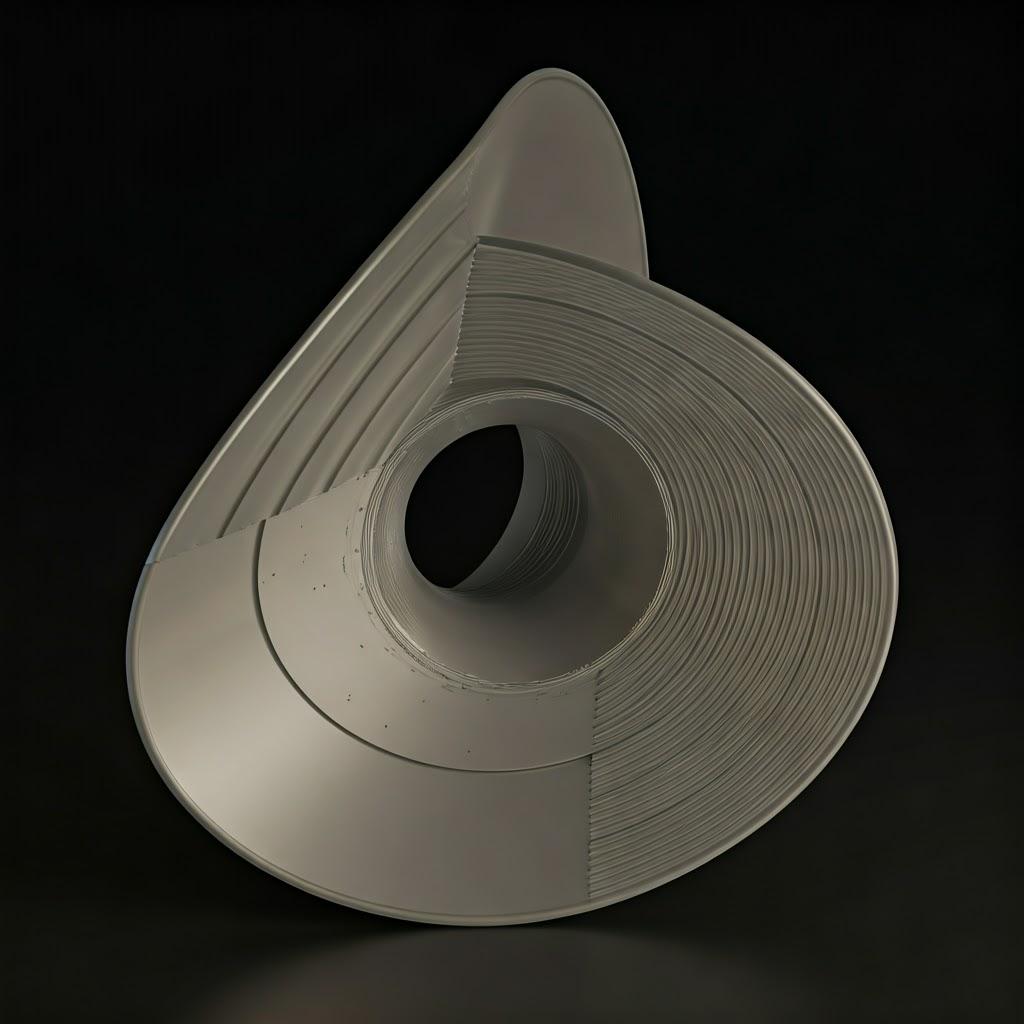
Selecting the Right Metal AM Partner: Evaluating Suppliers for Aerospace Components
The decision to leverage additive manufacturing for critical aerospace components like fuel nozzle tips is significant. However, equally critical is the selection of the right additive manufacturing partner. This is not merely a transactional supplier relationship; it’s a strategic partnership requiring trust, technical alignment, and a shared commitment to quality and reliability. For procurement managers and engineers navigating the complex landscape of metal AM service providers, a thorough evaluation process is paramount to ensure project success and component integrity.
Choosing an unsuitable partner can lead to delays, cost overruns, subpar component quality, and potentially catastrophic failures in application. Here are key criteria to consider when evaluating potential metal AM suppliers for aerospace-grade fuel nozzles:
1. Technical Expertise and Proven Experience:
- Alloy Specialization: Does the supplier have demonstrable experience printing with the specific superalloy required (IN718 or IN625)? Ask about their process development for these materials.
- Process Proficiency: Verify their expertise in the relevant AM process, typically Laser Powder Bed Fusion (L-PBF) for these applications. Understand their control over process parameters.
- Application Track Record: Have they successfully produced similar aerospace components, particularly those involving complex internal features, high temperatures, or stringent quality requirements? Request non-proprietary case studies or examples of relevant work. Aerospace component manufacturing requires specific domain knowledge.
2. Equipment, Technology, and Facilities:
- Industrial-Grade Machinery: Ensure they utilize modern, well-maintained industrial L-PBF systems known for accuracy and repeatability. Understand the machine manufacturer and model.
- Process Monitoring: Inquire about their use of in-situ process monitoring capabilities (e.g., melt pool monitoring, thermal imaging) which can provide valuable data for quality assurance.
- Facility Environment: Assess the cleanliness, organization, and environmental controls (humidity, temperature) of their production facility, especially regarding powder handling areas.
3. Material Quality, Sourcing, and Control:
- Powder Sourcing: Where do they source their IN718/IN625 powder? Do they manufacture it themselves or buy from qualified suppliers? Understanding the powder’s origin and quality is crucial.
- Powder Management: How do they handle, store, test, and recycle metal powders to ensure purity, prevent contamination, and maintain batch traceability? Improper handling can compromise part quality.
- Сертификация материала: Can they provide material certifications (e.g., Certificates of Conformance) verifying the powder’s chemical composition and properties? This is often a requirement for aerospace material procurement.
- Met3dp Advantage: This is where Met3dp’s unique position shines. As a manufacturer of high-performance metal powders using advanced Gas Atomization and PREP technologies, Met3dp has direct control over powder quality, consistency, and characteristics, ensuring an optimal starting point for critical AM builds. Our powders are specifically optimized for AM processes.
4. Comprehensive Post-Processing Capabilities:
- Integrated Workflow: Does the supplier offer the necessary post-processing steps in-house (stress relief, heat treatment, support removal, basic finishing)? Or do they manage a network of qualified subcontractors for specialized processes like HIP, advanced machining, certified NDT (CT scanning, FPI), and specific surface treatments?
- Expertise Across Steps: Ensure they (or their partners) possess the required expertise and certifications for each critical post-processing step, particularly heat treatment and NDT for aerospace standards. Understanding the full manufacturing chain is vital.
5. Robust Quality Management System (QMS):
- Сертификаты: This is non-negotiable for aerospace. Look for suppliers certified to AS9100 (the aerospace industry QMS standard) or at minimum ISO 9001 with demonstrated aerospace experience. Certification provides assurance of documented processes, traceability, quality control, and continuous improvement.
- Quality Procedures: Inquire about their specific procedures for process validation, part inspection, non-conformance management, and documentation practices.
- Traceability: Can they provide full traceability from raw powder batch to finished, inspected component?
6. Engineering, DfAM, and Application Support:
- Collaborative Approach: Do they offer Design for Additive Manufacturing (DfAM) support? Can their engineers collaborate with your team to optimize the nozzle design for printability, performance, and cost-effectiveness?
- Simulation Capabilities: Do they utilize process simulation tools to predict and mitigate risks like warpage or residual stress?
- Solutions Provider: Look for a partner who acts as a solutions provider, offering insights and support beyond just printing services. Met3dp prides itself on its decades of collective expertise in metal AM, providing comprehensive solutions that span equipment, materials, and услуги по разработке приложений.
7. Capacity, Lead Times, and Scalability:
- Meeting Demand: Assess their current capacity and ability to meet your project’s timeline and potential future production volume requirements.
- Realistic Lead Times: Obtain clear and realistic lead time estimates that account for the entire workflow, including all post-processing and inspection steps.
- Масштабируемость: Can they support a transition from prototyping to low-rate or even mid-rate production if required?
8. Communication, Location, and Logistics:
- Responsiveness: Evaluate their communication responsiveness and clarity.
- Location Factors: Consider the implications of the supplier’s location regarding shipping costs, transit times, communication across time zones, and any potential export control regulations for sensitive aerospace components. Met3dp, based in Qingdao, China, serves a global clientele and has experience navigating international logistics.
9. Cost vs. Value:
- Detailed Quotes: Ensure quotes are comprehensive, clearly outlining all included process steps, materials, quality assurance measures, and certifications.
- Value Proposition: Evaluate the cost not in isolation, but in the context of the supplier’s expertise, quality, reliability, and the overall value they bring to the project. For mission-critical parts, choosing the cheapest metal AM supplier is often a false economy.
Supplier Evaluation Checklist Summary:
Критерии | Основные вопросы | Importance (Aerospace) |
---|---|---|
Техническая экспертиза | Alloy/Process/Application experience? Case studies? | Критический |
Equipment & Technology | Machine type/condition? Process monitoring? | Высокая |
Material Quality/Control | Powder source? Handling procedures? Certifications? (Met3dp Advantage) | Критический |
Постобработка | In-house/Managed capabilities? Expertise in Heat Treat, HIP, NDT? | Критический |
Quality System (QMS) | AS9100 / ISO 9001 certified? Documented procedures? Traceability? | Mandatory |
Engineering/DfAM Support | DfAM expertise? Simulation? Collaborative approach? (Met3dp Advantage) | Очень высокий |
Capacity & Lead Time | Meets project schedule/volume? Realistic timelines? Scalability? | Высокая |
Communication/Logistics | Responsive? Location factors? Export controls? | От умеренного до высокого |
Cost vs. Value | Detailed quote? Price justified by quality/reliability/service? | Высокая |
Export to Sheets
Selecting the right partner requires due diligence but investing time in this evaluation process significantly increases the likelihood of receiving high-quality, reliable 3D printed fuel nozzle tips that meet the demanding requirements of aerospace propulsion systems.
Understanding Cost Drivers and Lead Times for Additive Manufactured Fuel Nozzles
While additive manufacturing offers significant advantages in design freedom and potential performance gains, understanding the factors that influence the cost and lead time of producing components like fuel nozzle tips is essential for effective project planning, budgeting, and procurement. AM costing differs significantly from traditional manufacturing, and lead times often involve more than just the print duration.
Key Cost Drivers for AM Fuel Nozzles:
- Стоимость материала:
- Alloy Price: Nickel-based superalloys like IN718 and IN625 are inherently expensive raw materials compared to standard steels or aluminum alloys.
- Качество порошка: Aerospace-grade powder, characterized by high purity, specific particle size distribution, and excellent sphericity (like those produced by Met3dp), commands a premium but is necessary for quality.
- Material Consumption: Includes the material in the final part plus any support structures and potentially wasted powder (though L-PBF is relatively efficient). Reducing support volume through DfAM helps lower costs.
- Machine Time (Build Time):
- Part Volume & Height: The primary driver is the time the AM machine is occupied. This depends on the overall volume of the part(s) being printed (bounding box) and, more significantly, the total build height (determining the number of layers).
- Сложность: Highly complex parts or builds with dense support structures increase print time.
- Machine Rate: Based on the capital cost of the industrial AM system, maintenance, consumables (filters, wipers), energy consumption, and inert gas usage.
- Расходы на оплату труда:
- Skilled Operators: Requires trained technicians for build setup, machine operation, monitoring, powder handling, part excavation, and removal.
- Post-Processing Labor: Significant labor is involved in support removal (often manual), part finishing, inspection setup, and operation of post-processing equipment.
- Engineering/Quality: Time spent on build preparation, quality checks, documentation, and project management.
- Design Complexity & DfAM Impact:
- While AM enables complexity, extremely intricate designs можно increase print time and support requirements. However, effective DfAM often leads to экономия средств through part consolidation (reducing assembly labor and supply chain complexity) and performance improvements that provide downstream value, potentially offsetting higher initial part costs.
- Расходы на постобработку:
- This is often a major component of the final part cost. Each step adds expense:
- Stress Relief/Heat Treatment: Furnace time, energy, controlled atmosphere costs.
- HIP: Specialized equipment, long cycles, batch processing costs.
- Обработка: CNC machine time, tooling (superalloys cause high tool wear), programming, setup.
- Удаление опоры: Labor-intensive, potentially specialized tooling (EDM, AFM).
- Отделка поверхности: Equipment, consumables, labor depending on the method.
- NDT/Inspection: High cost of equipment (especially CT scanners), certified inspectors, time per part.
- This is often a major component of the final part cost. Each step adds expense:
- Quality Assurance & Certification:
- The rigorous testing, documentation, and process controls required for aerospace quality assurance (e.g., AS9100 compliance) add overhead and cost but are essential for reliability.
- Объем заказа:
- While AM avoids traditional tooling costs, setup costs (build preparation, machine setup, powder loading/unloading) still exist. These setup costs are amortized over the number of parts in a build. Therefore, printing multiple parts simultaneously or placing bulk AM orders generally reduces the cost per part compared to single-piece orders, though the economies of scale are typically less dramatic than in mass production techniques like casting or injection molding.
Typical Lead Time Breakdown:
Lead time for a fully processed and inspected AM fuel nozzle is often driven more by post-processing and queuing than the print itself. A typical workflow might look like this:
- Order Processing & Preparation (1-5 days): File review, DfAM checks (if needed), build simulation, support generation, build file creation, scheduling.
- Machine Queue Time (Variable): Depends on supplier’s machine availability (can range from days to weeks).
- Printing (1-7+ days): Highly dependent on part size, height, complexity, and number of parts per build. Fuel nozzles might take 24 hours to several days to print.
- Post-Print Cooldown & Depowdering (0.5-1 day): Allowing the build chamber and parts to cool safely, careful removal of excess powder.
- Stress Relief (1-2 days): Including furnace loading, cycle time (often 8-24 hours including ramp-up/cool-down), unloading.
- Part Removal & Support Removal (1-3 days): Depending on method (saw/EDM) and complexity of supports. Can be a bottleneck.
- Heat Treatment (Solution/Age or Anneal) (2-4 days): Furnace time (can be long cycles), controlled cooling, potential for batching delays.
- HIP (3-7 days): Often involves batching and scheduling with specialized HIP providers; cycle times are long.
- Machining (Variable): Depends on complexity, setup time, machine availability (days to weeks if extensive).
- Finishing & Inspection (2-5 days): Surface finishing, comprehensive NDT (CT scanning can take time for setup, scanning, and analysis), final dimensional checks, documentation package preparation.
- Shipping (Variable): Depends on location and shipping method.
Total Lead Time: Adding these stages, typical lead times for complex superalloy AM components like fuel nozzles, requiring full post-processing and aerospace qualification, can range from 3 to 10 weeks, sometimes longer depending on complexity and supplier backlogs.
Главный вывод: When requesting quotes for AM fuel nozzles, ensure the supplier provides a detailed breakdown of included steps and associated costs and lead times. Understanding these drivers allows for better procurement planning, realistic scheduling, and informed decisions about the total cost of ownership, considering both manufacturing expenses and the potential performance benefits enabled by additive manufacturing.
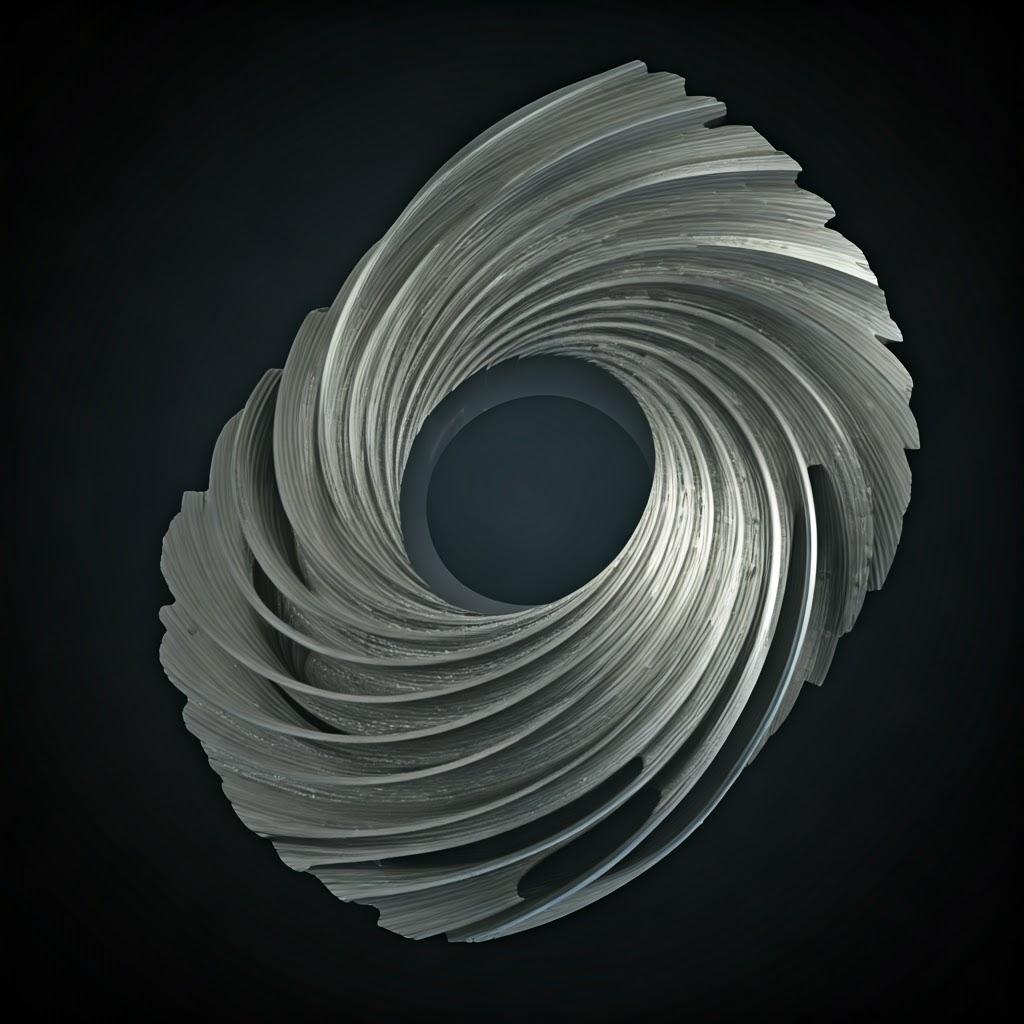
Frequently Asked Questions (FAQ) about 3D Printed Rocket Fuel Nozzle Tips
Here are answers to some common questions regarding the additive manufacturing of rocket fuel nozzle tips using superalloys:
Q1: Are 3D printed IN718 / IN625 fuel nozzles as strong and reliable as traditionally manufactured ones (e.g., wrought, cast)?
A: Yes, when designed, printed, and post-processed correctly, AM fuel nozzles made from IN718 or IN625 can exhibit mechanical properties (like tensile strength, yield strength, ductility, and importantly, fatigue life) that meet or even exceed those of conventionally manufactured counterparts. The fine-grained microstructure often achieved in L-PBF can be particularly beneficial for fatigue resistance. Key factors include using high-quality powder (like Met3dp’s), optimized print parameters, appropriate heat treatments (solutionizing, aging for IN718; annealing for IN625), and Hot Isostatic Pressing (HIP) to eliminate internal porosity. Rigorous qualification testing against established aerospace standards is essential to validate performance and reliability for any specific application.
Q2: What determines the lifespan or number of reuses for a 3D printed fuel nozzle tip?
A: The operational life and reusability depend on a complex interplay of factors, not solely the manufacturing method. Key elements include the specific engine design, its operating cycle (thrust levels, duration, number of starts), the propellant combination, the effectiveness of the cooling system (where AM’s conformal channels offer potential advantages), the chosen material (IN718 vs. IN625 properties), and the established inspection and refurbishment protocols. While AM enables designs potentially more resistant to certain failure modes (e.g., thermal fatigue due to better cooling), the actual lifespan is determined through extensive ground testing, flight data, and periodic non-destructive inspections (like CT scanning or FPI) between uses to detect any degradation or damage.
Q3: Can Met3dp manage the entire process, from supplying the powder to delivering a fully finished and inspected fuel nozzle?
A: Met3dp excels in producing high-purity, AM-optimized superalloy powders (IN718, IN625, etc.) using our advanced atomization technologies and offers state-of-the-art metal 3D printing equipment and services. We provide comprehensive решения для аддитивного производства and application development support, working closely with clients on DfAM and printing optimization. While we manage the core AM process, the extensive post-processing chain required for aerospace components (like certified heat treatment, HIP, specialized NDT such as CT scanning, precision machining to tight tolerances) often involves leveraging a network of trusted, accredited partners who specialize in these areas. Met3dp can coordinate and manage this workflow or recommend qualified partners, ensuring clients receive a fully vetted and certified component according to their specifications. Our focus is on providing a seamless path to high-quality AM parts, leveraging the best expertise at each step.
Q4: Is metal 3D printing a cost-effective solution for producing fuel nozzles, especially compared to traditional methods?
A: The cost-effectiveness of AM for fuel nozzles depends heavily on the specific application and production volume. AM shines brightest where:
- Complexity is High: Designs incorporate intricate internal features (conformal cooling, complex mixing geometries) that are difficult or impossible to achieve traditionally.
- Part Consolidation Offers Value: Reducing assembly complexity, weight, and potential failure points.
- Performance Gains are Significant: Optimized designs lead to measurable improvements in engine efficiency, thrust, or component life, justifying a potentially higher per-part cost.
- Development Time is Critical: Rapid prototyping and iteration cycles accelerate engine development.
- Volumes are Low to Medium: AM avoids high upfront tooling costs associated with casting, making it competitive for smaller production runs or customized designs. For very simple nozzle designs produced in high volumes, traditional methods like casting might remain cheaper on a per-part basis. However, a “total cost of ownership” analysis, considering development speed, assembly savings, and performance benefits, often reveals AM to be highly advantageous for advanced aerospace components.
Q5: What information is typically required to get an accurate quote for 3D printing a fuel nozzle tip from a supplier like Met3dp?
A: To provide an accurate quote, suppliers generally need the following:
- 3D CAD-модель: A high-quality model in a standard format (e.g., STEP, IGES).
- Material Specification: Clearly state the required alloy (e.g., IN718 or IN625) and any specific material standard (e.g., AMS specifications).
- Количество: Number of parts required (for the current order and potentially forecasted volumes).
- Critical Tolerances & Dimensions: A 2D drawing or annotated model highlighting critical dimensions, required tolerances, and datum features.
- Требования к чистоте поверхности: Specify required Ra values for critical internal and external surfaces.
- Required Post-Processing: List all mandatory steps (e.g., specific heat treatment cycle, HIP requirement, machining operations, surface treatments).
- Quality & Inspection Requirements: Specify necessary NDT methods (FPI, CT scan, etc.), required quality standards (e.g., compliance with AS9100), and any required documentation (material certs, inspection reports, certificate of conformance). Providing comprehensive information upfront enables suppliers like Met3dp to deliver accurate quotes and realistic lead times tailored to your specific needs.
Conclusion: Propelling the Future of Space Exploration with Met3dp’s Additive Manufacturing Solutions
The rocket fuel nozzle tip, operating at the fiery interface between propellant delivery and combustion, represents one of the most challenging components within a rocket engine. Its intricate design must ensure precise fuel atomization and injection under extreme temperatures, pressures, and corrosive conditions. Traditional manufacturing methods, while established, often struggle to produce the highly optimized geometries needed to push the boundaries of engine performance and efficiency.
Metal additive manufacturing, particularly utilizing high-performance superalloys like IN718 and IN625, has emerged as a transformative solution. AM unshackles designers from conventional constraints, enabling the creation of fuel nozzles with unparalleled geometric complexity. Features like integrated conformal cooling channels, consolidated assemblies reducing part count and failure points, and topology-optimized lightweight structures are now achievable, leading directly to tangible benefits: enhanced thermal management, improved combustion stability and efficiency, longer component life, and reduced overall engine mass.
However, realizing these benefits demands more than just access to a 3D printer. It requires a holistic approach encompassing rigorous Design for Additive Manufacturing (DfAM) principles, the use of exceptionally high-quality, AM-optimized metal powders, tightly controlled printing processes, and a comprehensive suite of specialized post-processing steps – including stress relief, heat treatment, Hot Isostatic Pressing (HIP), precision machining, and meticulous non-destructive testing – all governed by stringent aerospace quality standards.
Met3dp stands as a key enabler in this advanced manufacturing ecosystem. With our deep roots in materials science, demonstrated by our industry-leading Gas Atomization and PREP powder production technologies, we supply the high-purity, highly spherical IN718, IN625, and other critical alloy powders that form the foundation of reliable aerospace components. Our expertise extends to providing cutting-edge metal AM systems and comprehensive application development support, partnering with aerospace innovators to turn ambitious designs into flight-ready hardware. We understand the challenges and intricacies involved in producing mission-critical parts and are committed to delivering solutions that meet the highest levels of quality and performance.
As humanity continues to reach further into space, the demand for more powerful, efficient, and reliable propulsion systems will only grow. Metal additive manufacturing is not just an alternative production method; it is a critical enabling technology for this future. By leveraging the power of AM with expert partners like Met3dp, aerospace companies can accelerate innovation, optimize performance, and continue to propel the future of space exploration.
Ready to explore how Met3dp’s additive manufacturing capabilities can revolutionize your aerospace components? Contact us today to discuss your specific requirements with our team of experts.
Поделиться
Facebook
Twitter
LinkedIn
WhatsApp
Электронная почта
MET3DP Technology Co., LTD - ведущий поставщик решений для аддитивного производства со штаб-квартирой в Циндао, Китай. Наша компания специализируется на производстве оборудования для 3D-печати и высокопроизводительных металлических порошков для промышленного применения.
Сделайте запрос, чтобы получить лучшую цену и индивидуальное решение для вашего бизнеса!
Похожие статьи
О компании Met3DP
Последние обновления
Наш продукт
CONTACT US
Есть вопросы? Отправьте нам сообщение прямо сейчас! После получения Вашего сообщения мы всей командой выполним Ваш запрос.
Получите информацию о Metal3DP
Брошюра о продукции
Получить последние продукты и прайс-лист
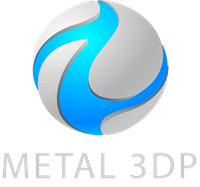
Металлические порошки для 3D-печати и аддитивного производства
КОМПАНИЯ
ПРОДУКТ
ИНФОРМАЦИЯ О КОНТАКТЕ
- Город Циндао, Шаньдун, Китай
- [email protected]
- [email protected]
- +86 19116340731