3D Printed Dental Frameworks Using Cobalt-Chrome
Spis treści
Introduction: The Precision Revolution in Dental Restorations with 3D Printed Cobalt-Chrome Frameworks
The field of restorative dentistry is constantly evolving, driven by the pursuit of greater precision, efficiency, and patient satisfaction. At the heart of many successful dental restorations, such as crowns, bridges, and removable partial dentures, lies the dental framework. This underlying structure provides the necessary support, stability, and retention for the final prosthetic, ensuring both functionality and longevity. Traditionally, crafting these intricate components involved labor-intensive lost-wax casting techniques, a process venerable yet often fraught with potential inaccuracies and limitations. Today, however, the dental industry stands at the cusp of a significant transformation, largely propelled by the advent of metal additive manufacturing (AM), more commonly known as 3D printing.
Specifically, the use of Drukowanie 3D technology to fabricate dental frameworks from Cobalt-Chrome (CoCr) alloys represents a paradigm shift. CoCr alloys have long been a material of choice in dentistry, valued for their excellent mechanical strength, corrosion resistance, and biocompatibility. Combining this proven material with the capabilities of Selective Laser Melting (SLM) or Direct Metal Laser Sintering (DMLS) technologies unlocks unprecedented levels of precision, design freedom, and production efficiency. This technological synergy allows dental laboratories and manufacturers to produce frameworks with micron-level accuracy, ensuring a passive fit that minimizes chair-side adjustment time for clinicians and enhances comfort for patients.
This technological advancement is not merely an incremental improvement; it’s a revolutionary step enabling the creation of complex geometries previously impossible or impractical to achieve through casting. Thin, delicate clasps for partial dentures, intricate substructures for multi-unit bridges, and customized bars for implant-retained prosthetics can now be designed and manufactured with remarkable fidelity. The digital workflow inherent in AM—from intraoral scanning and CAD design to direct 3D printing—streamlines the production process, reduces material waste, and offers unparalleled consistency from one framework to the next.
This comprehensive guide is designed for key stakeholders within the dental ecosystem, including forward-thinking Dental Laboratory Owners and Technicians, Prosthodontists seeking the best restorative solutions, Dental Procurement Managers evaluating new technologies and suppliers, and Wholesale Dental Suppliers looking to offer cutting-edge components. We will delve deep into the world of 3D printed CoCr dental frameworks, exploring their diverse applications, comparing the advantages of AM over traditional methods, examining the critical role of high-quality CoCrMo and CoCrW powders, and providing actionable insights into design considerations, achievable tolerances, post-processing requirements, and supplier selection. Understanding this technology is crucial for businesses aiming to stay competitive and deliver superior dental restorations in the modern era. Companies like Met3dp, with their expertise in both advanced metal powders and additive manufacturing systems, are instrumental in driving this transformation, providing the foundational technology for high-quality dental component production.
Applications: Where are 3D Printed CoCr Dental Frameworks Utilized?
The versatility, strength, and precision offered by 3D printed Cobalt-Chrome frameworks have led to their adoption across a wide spectrum of dental restorative applications. The ability to translate complex digital designs directly into highly accurate metal components makes this technology particularly suitable for patient-specific solutions where fit and function are paramount. Key utilization areas include:
- Removable Partial Denture (RPD) Frameworks: This is arguably one of the most significant applications for 3D printed CoCr. RPDs rely on a precisely fitting metal framework that engages remaining teeth for support and retention while providing a base for artificial teeth.
- Challenges with Casting: Traditional casting of RPD frameworks is notoriously complex and technique-sensitive. Achieving passive fit (without stressing abutment teeth) and crafting thin, yet strong, clasps and rests requires exceptional skill and can lead to inconsistencies. Porosity within cast clasps can also lead to premature fracture.
- AM Advantages: 3D printing allows for:
- Highly Accurate Fit: Directly printing from a digital design derived from an intraoral scan or model scan minimizes inaccuracies inherent in impression materials, stone models, waxing, investing, and casting. This leads to a superior passive fit, reducing adjustment needs.
- Intricate Clasp Design: AM enables the creation of complex, thin, and anatomically contoured clasps (e.g., I-bars, wrought wire style clasps) that provide excellent retention and aesthetics while minimizing tooth coverage. The rapid solidification during printing can result in a fine grain structure, potentially enhancing clasp fatigue resistance compared to some cast structures.
- Uniform Thickness: Consistent and controlled layer-by-layer fabrication ensures uniform thickness in major connectors and other structural elements, optimizing strength-to-weight ratios.
- Digital Design Integration: Seamless integration with CAD software allows for virtual articulation, survey line analysis, and precise placement of components like rests, guide planes, and retention grids.
- Target Industry: High-volume Dental Laboratories, specialized RPD manufacturers, and wholesale dental suppliers benefit significantly from the repeatability, speed, and precision of AM for RPD framework production.
- Substructures for Crowns and Bridges (Porcelain-Fused-to-Metal – PFM): While zirconia and other all-ceramic restorations are popular, PFM restorations built on metal substructures remain a reliable and widely used option, especially for multi-unit bridges requiring high strength and predictable long-term performance.
- Role of Substructure: The CoCr framework provides the load-bearing core, onto which porcelain is layered for aesthetics. A precise fit of the substructure over the prepared tooth (or teeth) is critical for marginal integrity and longevity.
- AM Advantages:
- Marginal Accuracy: 3D printing can achieve highly accurate margins (typically within 50-100 microns), reducing the risk of microleakage and recurrent decay.
- Optimized Coping Thickness: Consistent and minimal coping thickness can be achieved, allowing more space for aesthetic porcelain layering while maintaining strength.
- Complex Bridge Designs: Fabricating long-span or complex curvature bridge frameworks is more predictable and accurate with AM compared to casting, which can suffer from distortion during the cooling process.
- Improved Porcelain Bond (Potentially): The surface texture created by some AM processes, potentially combined with specific post-processing like oxidation firing, can be optimized for reliable porcelain adhesion.
- Target Industry: Dental Labs specializing in crown and bridge work, cosmetic dentists, and dental component distributors catering to fixed prosthodontics.
- Implant-Supported Bars and Frameworks: For implant-retained overdentures or fixed hybrid prostheses, a rigid and passively fitting bar or framework connecting multiple implants is essential for distributing occlusal forces and ensuring long-term implant stability.
- Critical Requirement: Passive Fit: Any misfit in an implant framework can induce strain on the implants and surrounding bone, potentially leading to complications like screw loosening, component fracture, or even implant failure. Achieving passive fit with multi-implant restorations using traditional casting methods is exceptionally challenging due to cumulative errors.
- AM Advantages:
- Unsurpassed Passive Fit: This is where AM truly excels. By printing directly from a precise digital model incorporating implant positions (obtained via scan bodies), 3D printing significantly minimizes the processing errors associated with casting. This results in frameworks that seat passively onto the implants with minimal strain.
- Geometrie złożone: AM allows for the design and fabrication of intricate bar shapes (e.g., Hader bars, Dolder bars, custom wrap-around designs) with integrated retention features for the denture or prosthetic teeth.
- Material Homogeneity: Printed frameworks exhibit greater material density and homogeneity compared to cast frameworks, reducing the likelihood of internal defects that could compromise strength.
- Wydajność: For complex multi-implant cases, the digital workflow and direct printing offered by AM can be significantly faster and more predictable than traditional multi-stage casting and soldering/welding techniques.
- Target Industry: Implant Dentists, Prosthodontists, specialized Implant Laboratories, dental implant manufacturers, and B2B suppliers of implant components.
Functional Benefits Summary Table:
Cecha | Korzyści | Relevant Application(s) |
---|---|---|
Precision Fit | Reduced chair time, patient comfort, longevity, passive implant seating | RPDs, Crown & Bridge, Implants |
Biokompatybilność | Patient safety, reduced allergic reactions, tissue health | All Applications |
Wysoka wytrzymałość | Durability, resistance to fracture under chewing forces | RPDs, Bridges, Implant Bars |
Odporność na korozję | Stability in the oral environment, longevity | All Applications |
Swoboda projektowania | Optimized function, aesthetics, retention (e.g., complex clasps) | RPDs, Implants, Complex Bridges |
Spójność | Predictable quality, reliable performance batch-to-batch | High-volume Labs, Suppliers |
Export to Sheets
Understanding these diverse applications highlights the transformative impact of 3D printed CoCr technology. It empowers dental professionals and manufacturers to deliver restorations that are not only functional and durable but also fabricated with unprecedented levels of accuracy and efficiency, directly benefiting both the provider and the patient.
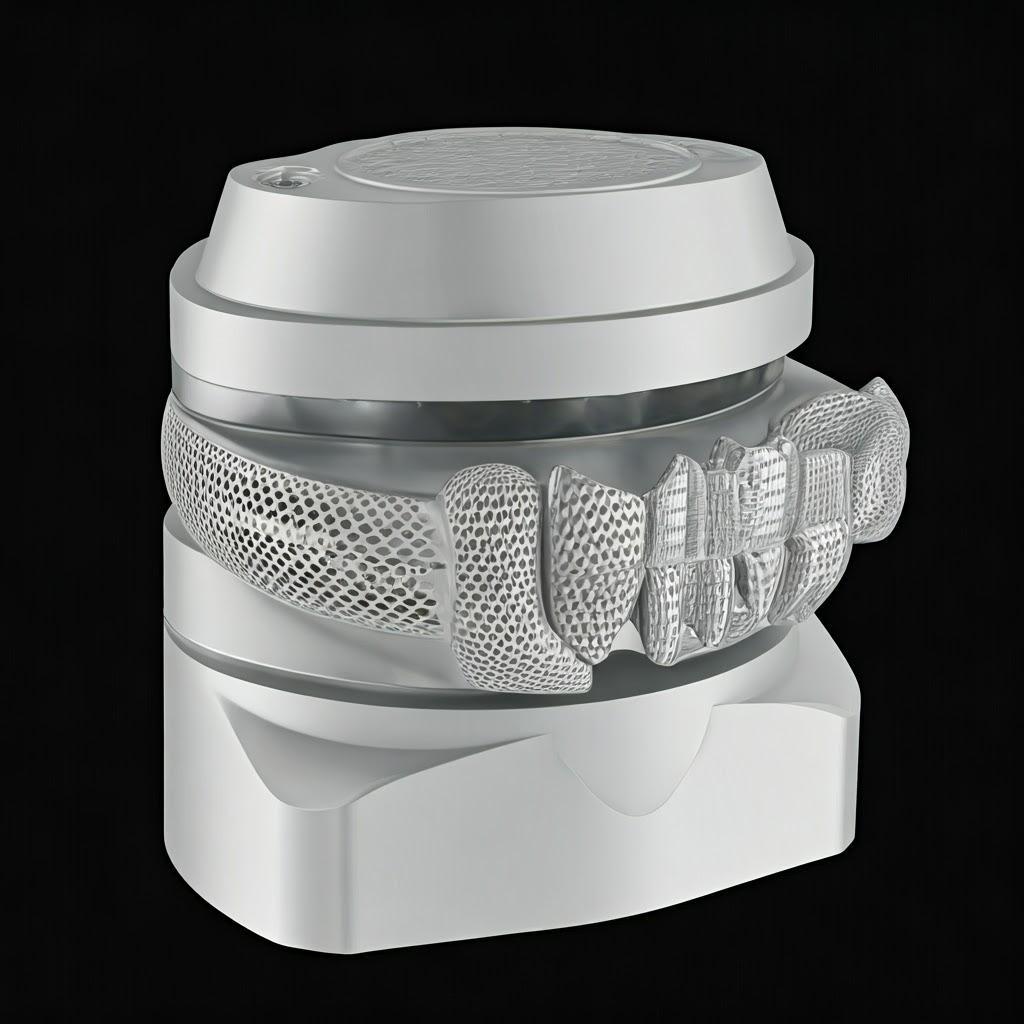
Advancing Dentistry: Why Choose Metal 3D Printing for Dental Frameworks Over Casting?
For decades, the lost-wax casting technique has been the cornerstone of fabricating metal dental frameworks. This multi-step process, involving wax pattern creation, investment, burnout, melting alloy, casting, divesting, and extensive finishing, requires significant manual dexterity, time, and is susceptible to cumulative errors at each stage. While skilled technicians can achieve excellent results, the inherent variability and limitations of casting are increasingly being addressed by the adoption of metal additive manufacturing (AM), specifically Selective Laser Melting (SLM) or Direct Metal Laser Sintering (DMLS). The advantages of using AM for producing CoCr dental frameworks are compelling, offering significant benefits to dental labs, clinicians, wholesale dental suppliers, and ultimately, patients.
Direct Comparison: Additive Manufacturing (SLM/DMLS) vs. Traditional Lost-Wax Casting
Cecha | Additive Manufacturing (SLM/DMLS) | Lost-Wax Casting | Advantage of AM |
---|---|---|---|
Przepływ pracy | Digital (Scan -> CAD -> Print -> Post-Process) | Analog/Manual (Impression -> Model -> Wax -> Invest -> Cast -> Finish) | Streamlined, less manual intervention, reduced potential for human error. |
Accuracy & Fit | High (typically ±50−100μm), excellent passive fit | Variable, dependent on multiple steps, potential for distortion | Superior fit, less chair-side adjustment, critical for implant cases. |
Złożoność projektu | High freedom, intricate details, thin sections, internal channels possible | Limited by waxing/investing process, difficult thin sections | Enables optimized designs, complex clasps, lightweight structures. |
Odpady materiałowe | Low (unused powder typically recyclable) | Moderate to High (sprues, casting button, finishing debris) | More sustainable, cost-effective material utilization (important for B2B). |
Spójność | High repeatability batch-to-batch (with process control) | Lower, dependent on technician skill and process variables | Predictable quality, reliable for bulk/wholesale dental framework production. |
Porowatość | Low to negligible (near full density achievable) | Potential for gas/shrinkage porosity (can weaken structure) | Improved mechanical integrity, reduced fracture risk. |
Czas realizacji | Potentially faster, especially for complex or multiple units | Can be lengthy due to multiple manual steps | Faster delivery for labs and dental distributors, improved patient scheduling. |
Labor Requirement | Less manual labor for fabrication, more for digital prep/post-proc | Highly labor-intensive throughout the process | Potential for cost savings and resource reallocation in labs. |
Właściwości materiału | Fine grain structure due to rapid cooling, potentially enhanced properties | Coarser grain structure, standard properties | Potentially higher strength and fatigue resistance (dependent on alloy/post-proc). |
Export to Sheets
Key Advantages of AM Elaborated:
- Unmatched Geometric Complexity and Design Freedom: AM builds parts layer by layer directly from a 3D CAD model. This liberates designers from the constraints imposed by traditional manufacturing methods. Dental technicians can design frameworks with:
- Thinner, More Anatomical Clasps: Creating clasps that are less bulky, follow tooth contours precisely, and provide optimal retention without compromising strength.
- Intricate Retention Features: Designing precise meshwork or beads for acrylic/composite bonding in RPDs or implant frameworks.
- Optimized Connectors: Creating strong yet minimally invasive connectors for bridges and partials.
- Internal Cooling Channels (Experimental): Research is exploring complex designs not feasible with casting.
- Superior Accuracy and Fit: The digital thread from scan to print minimizes the cumulative errors common in the analog casting workflow (impression distortion, model expansion, wax shrinkage, investment expansion, casting shrinkage). This results in frameworks, especially critical multi-unit implant structures, that fit the model or patient anatomy with exceptional precision, drastically reducing the need for time-consuming and potentially damaging chair-side adjustments. This precision is a key selling point for dental framework suppliers targeting quality-conscious labs and clinics.
- Enhanced Material Efficiency and Sustainability: AM, particularly powder bed fusion processes like SLM/DMLS, is inherently additive. Material is only solidified where needed, layer by layer. While support structures are necessary, the overall material usage is often significantly less than casting, which requires sprues, risers, and a casting button, much of which becomes scrap. Furthermore, unused metal powder in the build chamber can typically be sieved and reused multiple times (with proper quality control), making AM a more sustainable and resource-efficient process, an increasingly important factor for environmentally conscious businesses and procurement managers.
- Accelerated Turnaround Times and Digital Workflow Efficiency: While a single casting might be relatively quick po the wax-up is complete, the entire end-to-end process is lengthy. The digital workflow of AM allows for rapid design iterations. Once the design is finalized, multiple unique frameworks can often be nested and printed simultaneously within a single build cycle, typically running overnight. This parallel processing capability can significantly shorten overall production lead times, especially for dental laboratories or wholesale dental framework manufacturers handling high volumes or complex cases. This speed advantage allows for faster case completion and improved service levels.
- Unparalleled Consistency and Repeatability: Once optimal process parameters are established and validated for a specific machine and powder combination, AM processes offer remarkable consistency. Each part produced will be virtually identical to its digital blueprint, assuming proper machine calibration and quality control are maintained. This level of repeatability is difficult to achieve consistently with manual casting techniques, making AM ideal for manufacturers and suppliers who need to guarantee predictable quality across large batches of dental frameworks.
- Potential for Enhanced Mechanical Properties: The extremely rapid heating and cooling cycles inherent in SLM/DMLS result in a very fine-grained microstructure within the solidified CoCr alloy. This fine microstructure can, in some cases and particularly after appropriate heat treatment, lead to improved mechanical properties such as yield strength and fatigue resistance compared to the coarser grain structure typically found in cast components. This can be advantageous for delicate components like RPD clasps.
While casting remains a viable option, particularly for simpler cases or in settings without access to AM technology, the clear advantages of metal 3D printing in terms of precision, design freedom, efficiency, and consistency position it as the superior technology for manufacturing high-quality, complex Cobalt-Chrome dental frameworks. Investing in or partnering with providers of druk 3D z metalu services allows dental businesses to elevate the standard of care and optimize their production workflows.
Material Focus: Cobalt-Chrome Alloys (CoCrMo, CoCrW) for Dental Additive Manufacturing
The success of any dental restoration hinges significantly on the material used. For 3D printed dental frameworks, Cobalt-Chrome (CoCr) alloys, specifically formulations containing Molybdenum (CoCrMo) or Tungsten (CoCrW), are the dominant materials of choice. These alloys have a long history of safe and effective use in medical and dental applications, primarily due to their exceptional combination of biocompatibility, mechanical strength, and corrosion resistance. The transition to additive manufacturing has necessitated the development and optimization of CoCr powders specifically designed for processes like SLM and DMLS.
Introducing CoCrMo and CoCrW Powders for AM:
- CoCrMo: This is the most widely used formulation for dental applications. The addition of Molybdenum primarily enhances pitting corrosion resistance and contributes to strength through solid solution strengthening. It typically contains around 26-30% Chromium, 5-7% Molybdenum, and minor additions of other elements like Silicon, Manganese, and sometimes Nitrogen, with Cobalt making up the balance.
- CoCrW: This variation includes Tungsten (often alongside Molybdenum or partially replacing it). Tungsten primarily increases the alloy’s hardness, rigidity (elastic modulus), and high-temperature strength, although it can sometimes make the alloy slightly more challenging to finish and polish. The choice between CoCrMo and CoCrW might depend on the specific application requirements (e.g., rigidity needed for long-span bridges) and the specific powder/process validation performed by the AM service provider or dental framework manufacturer.
Key Properties and Why They Matter for Dental Frameworks:
Nieruchomość | Opis | Importance in Dental Applications | Typical Values (AM CoCrMo)* |
---|---|---|---|
Biokompatybilność | The ability of the material to exist within the body without eliciting adverse local or systemic responses. | Essential for patient safety. Prevents allergic reactions, inflammation, or toxicity. Compliance with standards like ISO 10993 is critical for dental suppliers. | Meets ISO 10993 requirements |
Odporność na korozję | Resistance to degradation due to chemical reactions with the oral environment (saliva, food, fluids). | Prevents metal ion release (potential allergen/toxicity), maintains structural integrity and aesthetics, ensures longevity. Crucial for B2B supply chain trust. | Excellent; passive layer formation |
Wysoka wytrzymałość | Ability to withstand chewing forces without permanent deformation (Yield Strength) or fracture (Ultimate Tensile Strength). | Ensures durability of frameworks, especially thin sections like clasps or connectors. Prevents failure under normal function. | Yield: >550 MPa, UTS: >850 MPa |
Rigidity/Stiffness | Resistance to bending or flexing under load (Elastic Modulus / Young’s Modulus). | Important for supporting restorations (crowns/bridges) and distributing forces evenly, especially in long-span bridges or implant bars. | Approx. 200-220 GPa |
Twardość | Resistance to scratching and wear. | Contributes to long-term durability against wear from opposing teeth or cleaning. | > 300 HV (Vickers Hardness) |
Odporność na zużycie | Ability to resist material loss due to friction. | Important for components like rests and clasps that contact natural teeth or other restorations. | Dobry |
Gęstość | Mass per unit volume. | Relatively high compared to titanium or resins; influences the overall weight of the prosthesis. AM allows for optimized, lighter designs. | ~ 8.3 – 8.6 g/cm³ |
Weldability/ Solderability | Ability to be joined using welding or soldering techniques. | Can be important for labs if minor repairs or additions are needed post-fabrication (though less common with precise AM parts). | Generally good |
Export to Sheets
*Note: Exact properties depend significantly on the specific powder composition, AM process parameters (e.g., laser power, scan speed, layer thickness), build orientation, and post-processing heat treatments. These values are indicative.
The Critical Role of Powder Quality:
The quality of the CoCrMo or CoCrW metal powder used in the AM process is paramount to achieving dense, defect-free, and reliable dental frameworks. Inferior powder can lead to printing failures, porosity, poor surface finish, and inconsistent mechanical properties. Key powder characteristics targeted by high-quality dental powder suppliers include:
- Wysoka sferyczność: Spherical powder particles flow easily and pack densely in the powder bed. This ensures uniform layers and consistent melting, minimizing the risk of voids or porosity in the final part. Companies like Met3dp utilize advanced gas atomization techniques, employing unique nozzle and gas flow designs specifically engineered to produce metallic spheres with exceptional sphericity.
- Optimized Particle Size Distribution (PSD): A controlled PSD ensures good powder bed density and predictable melting behavior. Too many fine particles can hinder flowability and pose safety hazards, while too many coarse particles can lead to poor surface finish and resolution. Typical PSD for SLM/DMLS is in the range of 15-53 microns.
- Good Flowability: Closely related to sphericity and PSD, good flowability ensures the recoater mechanism can spread thin, uniform layers of powder across the build platform consistently. Poor flowability leads to defects.
- High Purity / Controlled Chemistry: The powder must adhere strictly to the specified chemical composition (e.g., ASTM F75 standards for CoCrMo). Contaminants or variations in alloying elements can negatively impact biocompatibility, corrosion resistance, and mechanical properties. Stringent quality control during powder manufacturing, handling, and recycling is essential.
- Low Moisture and Oxygen Content: Excess moisture or oxygen can lead to increased porosity during printing. Powders must be manufactured, stored, and handled in controlled environments.
Choosing a 3D printing service provider or a wholesale dental framework manufacturer that utilizes high-quality, traceable CoCr powders from reputable sources is crucial. Met3dp, for instance, not only manufactures advanced AM systems but also specializes in producing high-performance metal powders, including CoCr alloys optimized for dental applications through techniques like gas atomization and Plasma Rotating Electrode Process (PREP). Their commitment to quality control, from powder production using industry-leading technologies to final part verification, ensures that dental frameworks meet the stringent requirements for biocompatibility, strength, and precision demanded by the dental industry. Partnering with suppliers who prioritize material quality provides confidence in the final product’s performance and safety.
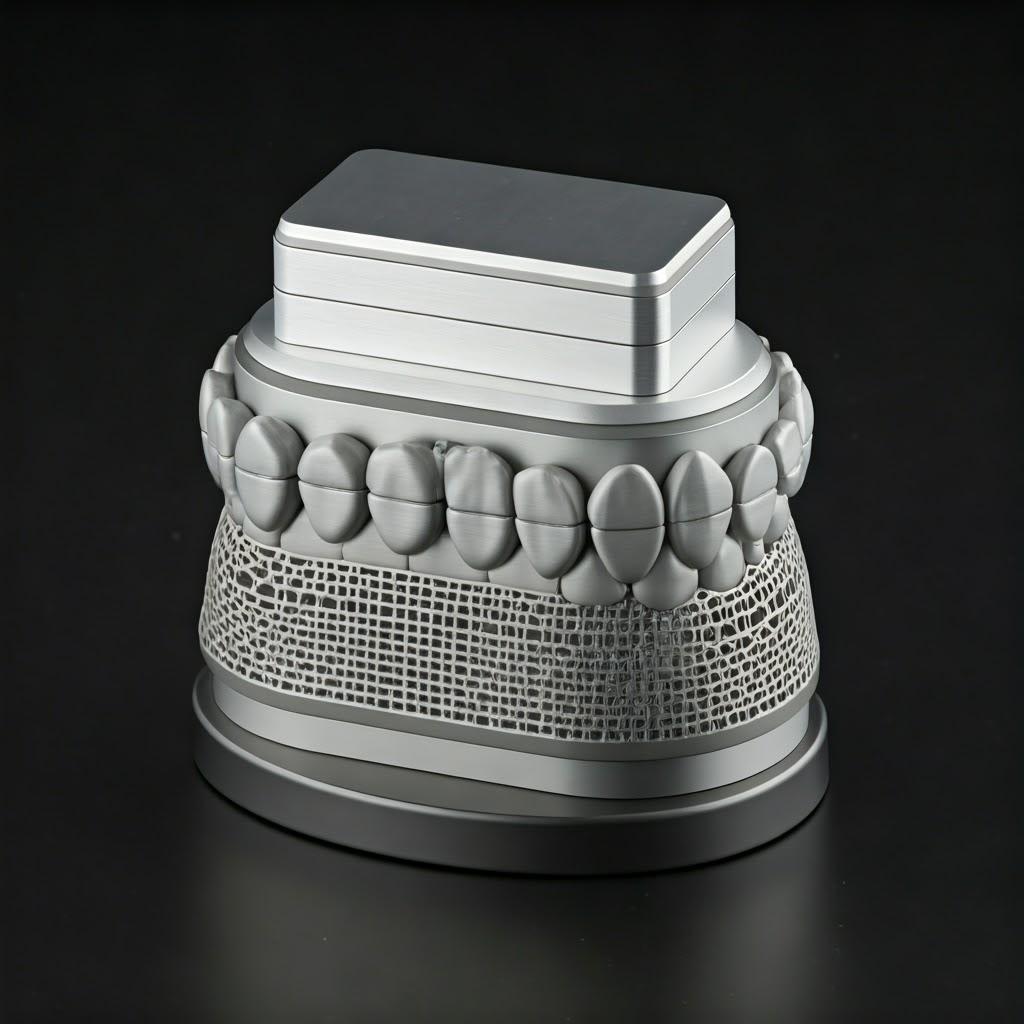
Design for Additive Manufacturing (DfAM): Optimizing CoCr Dental Frameworks for 3D Printing
Transitioning from traditional casting to metal additive manufacturing for Cobalt-Chrome dental frameworks isn’t just about swapping production methods; it necessitates a fundamental shift in design philosophy. Designing for Additive Manufacturing (DfAM) is crucial to fully leverage the benefits of 3D printing, ensuring functional success, manufacturability, and cost-effectiveness. Simply replicating a design intended for casting often fails to capitalize on AM’s strengths and can even introduce printing challenges. Dental technicians, designers, and engineers collaborating with dental framework manufacturers must embrace DfAM principles tailored to the specific nuances of processes like Selective Laser Melting (SLM).
Why DfAM Matters for Dental Frameworks:
- Unlocking Geometric Freedom: AM builds layer by layer, allowing for complex shapes, internal channels (though less common in frameworks), and fine features difficult or impossible to cast. DfAM encourages designers to think beyond traditional constraints.
- Minimizing Support Structures: Support structures are often necessary in SLM to anchor the part to the build plate, support overhangs, and manage thermal stress. However, they consume material, increase print time, require removal (adding labor and potentially damaging surfaces), and can limit design access. Effective DfAM aims to minimize reliance on supports through intelligent orientation and feature design (e.g., using self-supporting angles).
- Controlling Thermal Effects: The intense localized heat of the laser can cause thermal stresses, potentially leading to warping or distortion, especially in large or thin structures like RPD frameworks or long-span bridges. DfAM incorporates strategies to mitigate these stresses, such as optimizing orientation, adding sacrificial stiffening features, or adjusting geometry.
- Ensuring Manufacturability: Certain features might be challenging to print reliably (e.g., extremely thin walls below process capability, features smaller than the laser spot size, or deep, narrow channels where powder removal is difficult). DfAM ensures the design is compatible with the chosen AM process parameters and material.
- Optimizing for Post-Processing: Design decisions impact subsequent post-processing steps. For instance, designing support structures for easy access and removal, ensuring sufficient material for machining critical interfaces, or designing surfaces intended for porcelain bonding requires forethought during the CAD phase.
Key DfAM Principles for CoCr Dental Frameworks:
- Minimum Feature Size and Wall Thickness:
- Understanding Limits: Every SLM machine and parameter set has limitations on the smallest positive feature (e.g., a retention bead) and the thinnest stable wall it can reliably produce. For CoCr, minimum printable wall thickness is often around 0.3-0.5 mm, though practical design might dictate thicker sections for strength and rigidity.
- Zastosowanie: Clasps, connectors, and marginal areas must respect these minimums. Designing features below the limit can lead to incomplete formation or fragility. Conversely, AM allows for thinner, more uniform walls than casting in many areas, enabling lighter-weight designs.
- Orientation Strategy:
- Uderzenie: How a part is oriented on the build plate profoundly affects support requirements, surface finish (undersides supported areas are rougher), print time, and potential for distortion.
- Considerations for Dental:
- RPDs: Often printed at an angle (e.g., 45 degrees) to minimize supports under clasps and connectors and reduce the cross-sectional area per layer, mitigating stress. Critical rests and guide planes might dictate specific orientations.
- Crown/Bridge Substructures: Often oriented with the margin opening angled upwards to ensure marginal accuracy and avoid supports near the critical fit surface. Occlusal or connector surfaces might be oriented for optimal strength.
- Implant Bars: Orientation must prioritize passive fit at implant interfaces and minimize distortion over the span of the bar. Supporting the bar effectively without interfering with connection points is key.
- Collaboration: Orientation is often best determined collaboratively between the dental lab/designer and the AM service provider or dental framework supplier, leveraging their machine-specific expertise.
- Support Structure Design and Minimization:
- Self-Supporting Angles: Features angled above a certain threshold relative to the build plate (typically 40-45 degrees for CoCr) can often print without supports. Designers should utilize fillets, chamfers, and angled transitions where possible to create self-supporting geometries.
- Strategic Support Placement: Where supports are unavoidable (e.g., overhangs, starting points of clasps, large flat areas parallel to the build plate), they must be designed for:
- Stabilność: Adequately anchoring the part and resisting thermal stresses.
- Easy Removal: Using minimal contact points (e.g., perforated or cone supports), placing them on non-critical surfaces where possible, and ensuring physical access for removal tools.
- Heat Dissipation: Acting as heat sinks to draw heat away from critical areas.
- Software Tools: AM preparation software includes sophisticated tools for generating various types of support structures (block, line, cone, tree).
- Designing for Porcelain Adhesion (PFM Restorations):
- Sufficient Space: Ensure adequate, uniform reduction for the desired porcelain thickness (typically 1-2 mm).
- Supportive Design: Avoid sharp internal angles in the substructure where stress could concentrate in the porcelain. Provide smooth, rounded contours.
- Retention Features (Optional): While often achieved through surface roughening/oxidation post-processing, some designs might incorporate microscopic retention beads or grooves, easily achievable with AM, although their necessity is debated.
- Managing Shrinkage and Distortion:
- Predictive Compensation: AM software can sometimes apply scaling factors to compensate for anticipated thermal shrinkage, although this is complex.
- Design for Stiffness: Incorporating temporary bracing or tie-bars (to be removed during post-processing) in large or flexible frameworks (like RPDs or long bridges) can help maintain dimensional stability during printing and heat treatment.
- Stress Distribution: Avoiding large, flat sections parallel to the build plate and using variable thickness or ribbed structures can help distribute thermal stress more evenly.
Software and Collaboration:
The process typically involves designing the framework in specialized dental CAD software (e.g., Exocad, 3Shape, Dental Wings) based on scan data. This initial design, often created with casting in mind, must then be evaluated and potentially modified for AM using build preparation software (e.g., Materialise Magics, Netfabb). This software allows for optimal orientation, support generation, slicing the model into layers, and assigning laser parameters. Effective collaboration between the dental technician providing the initial design and the AM specialist operating the printing equipment is vital. Forward-thinking dental framework manufacturers and suppliers often provide DfAM guidelines or offer design consultation services to ensure optimal results.
By consciously applying DfAM principles, dental professionals and their manufacturing partners can move beyond simply replicating cast designs and truly harness the power of additive manufacturing printing methods to create superior, highly accurate, and optimized Cobalt-Chrome dental frameworks.
Achieving Precision: Tolerance, Surface Finish, and Dimensional Accuracy in 3D Printed Frameworks
One of the primary drivers for adopting metal 3D printing in dentistry is the pursuit of superior precision compared to traditional techniques. For dental frameworks, achieving tight tolerances, controlled surface finishes, and high dimensional accuracy is not just desirable – it’s essential for clinical success. Whether it’s the passive fit of an implant bar, the marginal seal of a crown substructure, or the comfortable seating of an RPD, precision dictates performance. Understanding the levels of accuracy achievable with SLM/DMLS for CoCr alloys and the factors influencing it is critical for dental labs, procurement managers sourcing from dental component distributors, and clinicians evaluating restoration quality.
Tolerances in SLM/DMLS Printed CoCr:
- General Achievability: State-of-the-art SLM/DMLS systems, when properly calibrated and operated with validated parameters, can typically achieve dimensional tolerances in the range of ±50 to ±100 microns (μm) for well-designed CoCr dental components. In some cases, particularly for smaller features or critical dimensions with careful process control, tolerances closer to ±20 to ±50 microns might be attainable.
- Comparison to Casting: This level of precision generally surpasses that achievable with traditional lost-wax casting, where the cumulative errors from impressions, models, waxing, investing, and casting often lead to tolerances in the range of ±100 to ±200 microns or sometimes greater, especially for complex or long-span structures.
- Factors Influencing Tolerance:
- Machine Calibration: Regular calibration of the laser scanner system, optics, and build platform leveling is fundamental.
- Process Parameters: Laser power, scan speed, layer thickness, hatch spacing, and scan strategy all influence melt pool dynamics and final part accuracy. Validated parameter sets are crucial.
- Jakość proszku: Consistent particle size distribution, sphericity, and flowability (as provided by quality-focused suppliers like Met3dp) contribute to uniform powder layers and predictable melting, enhancing accuracy.
- Zarządzanie temperaturą: Effective heat dissipation, appropriate support strategies, and optimized build orientation minimize thermal stress-induced warping and distortion.
- Part Design (DfAM): Complex geometries, thin walls, and large overhangs inherently present greater challenges for achieving tight tolerances.
- Przetwarzanie końcowe: Stress relief heat treatment can cause minor dimensional changes (shrinkage/growth), which must be anticipated. Machining or grinding steps obviously affect final dimensions.
Surface Finish (Roughness):
- As-Printed Surface: Parts directly off the SLM machine have a characteristic surface texture resulting from the layer-by-layer fusion process and partially melted powder particles adhering to the surface. Surface roughness (typically measured as Ra – arithmetic average roughness) varies depending on orientation:
- Upward-facing surfaces: Generally smoother, Ra values might range from 6-12 μm.
- Side-facing (vertical) surfaces: Can show layer lines, Ra values might be slightly higher, 8-15 μm.
- Downward-facing (supported) surfaces: Significantly rougher where support structures were attached, Ra values can exceed 15-20 μm.
- Post-Processed Surface: The as-printed surface is rarely acceptable for the final dental application (except perhaps internal fitting surfaces in some cases). Post-processing significantly alters the surface finish:
- Sandblasting/Bead Blasting: Creates a uniform, matte finish, removing loosely attached particles. Ra might be reduced slightly or remain similar but more uniform (e.g., Ra 5-10 μm). Often used for internal surfaces or prior to polishing/porcelain bonding.
- Tumbling/Mass Finishing: Can smooth surfaces and edges, especially for RPD frameworks, reducing Ra values.
- Manual Grinding/Polishing: Can achieve highly smooth, mirror-like finishes required for external surfaces of RPDs or metal collars. Ra values can be brought down to < 0.5 μm.
- Electropolishing: Can selectively remove material peaks, resulting in a smoother, brighter, and potentially more corrosion-resistant surface.
- Relevance:
- Fit: Smoother internal surfaces generally contribute to better fit and seating.
- Hygiene: Polished external surfaces are less prone to plaque accumulation.
- Estetyka: High polish is required for visible metal components.
- Porcelain Bonding: A specific surface texture (often achieved by blasting and controlled oxidation) is needed for micromechanical retention of porcelain.
Dimensional Accuracy and Its Importance:
Dimensional accuracy refers to how closely the final manufactured part conforms to the original digital design dimensions. In dental frameworks, this is paramount:
- Passive Fit: As discussed, especially for implant bars and bridges, the framework must seat perfectly onto implants or abutments without inducing stress. High dimensional accuracy across the entire span of the restoration is the only way to achieve this. AM’s digital workflow provides the best foundation for achieving passive fit.
- Marginal Integrity: For crown and bridge substructures, the accuracy of the margin determines the seal against the prepared tooth, preventing leakage and ensuring longevity. AM allows for consistently accurate margins.
- RPD Seating: Accurate adaptation of rests, guide planes, and clasps to the teeth ensures the RPD seats fully and comfortably, providing the planned support and retention without stressing the abutment teeth.
Quality Control and Metrology:
Reputable dental framework manufacturers and AM service providers employ rigorous quality control measures to verify precision:
- Coordinate Measuring Machines (CMMs): Tactile probes measure specific points to verify critical dimensions, especially for implant interfaces.
- 3D Optical Scanning: Non-contact scanners compare the final part geometry against the original CAD model, providing a comprehensive deviation map.
- Fit Checks: Testing the framework fit on the original printed model or, in some workflows, directly on a master cast or verification jig.
Achieving the required precision for dental frameworks is a synergistic effort involving optimized design (DfAM), high-quality materials, precise AM machinery like those offered by Met3dp, validated process parameters, meticulous post-processing, and robust quality control. Dental procurement managers and lab owners should inquire about a potential supplier’s capabilities and procedures in all these areas to ensure they can consistently deliver frameworks meeting the stringent accuracy demands of modern dentistry.
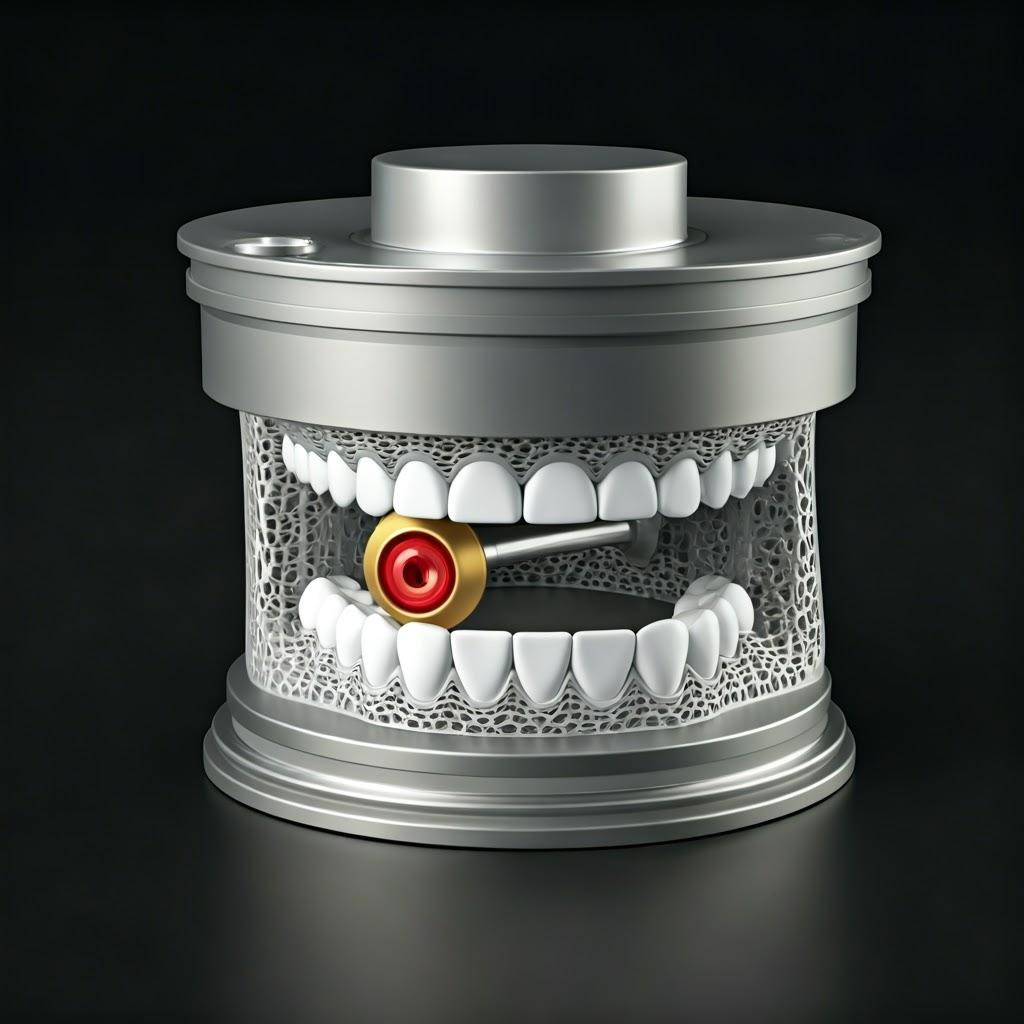
Beyond Printing: Essential Post-Processing Steps for CoCr Dental Frameworks
The journey of a 3D printed Cobalt-Chrome dental framework doesn’t end when the SLM machine cycle finishes. The “green part” removed from the build chamber requires a series of crucial post-processing steps to transform it into a functional, biocompatible, and aesthetically acceptable dental restoration component. These steps are not optional; they are integral to achieving the desired mechanical properties, dimensional accuracy, surface finish, and overall quality. Understanding this workflow is essential for dental labs managing production and for procurement managers evaluating the capabilities of potential dental contract manufacturing partners or wholesale dental framework suppliers.
Breakdown of Common Post-Processing Requirements:
- Stress Relief Heat Treatment:
- Cel: This is arguably the most critical post-processing step. The rapid heating and cooling cycles during SLM induce significant internal stresses within the printed CoCr part. If not relieved, these stresses can cause warping/distortion (especially after removal from the build plate or during subsequent heating like porcelain firing) and can negatively impact fatigue life and ductility. Stress relief homogenizes the microstructure to some extent.
- Proces: Parts (often while still attached to the build plate via supports) are heated in a controlled atmosphere furnace (typically argon or vacuum to prevent oxidation) to a specific temperature (e.g., 650°C – 850°C for CoCr, depending on the exact alloy and desired properties, sometimes even higher up to 1150°C for homogenization), held for a duration (e.g., 1-2 hours), and then slowly cooled. Precise parameters are alloy and application-specific and must be validated.
- Importance: Skipping or improperly performing stress relief significantly compromises the part’s dimensional stability and mechanical integrity.
- Removal from Build Plate & Support Structure Removal:
- Proces: Once heat-treated (ideally), the build plate is removed from the printer. The frameworks are then separated from the plate, typically using:
- Wire Electrical Discharge Machining (Wire EDM): Precise and clean cut, often preferred for minimizing stress on the part.
- Band Saw / Hand Tools: Can be used but require more care to avoid damaging the part.
- Usuwanie wsparcia: Supports must be carefully detached from the framework itself. This is often done manually using pliers, cutters, grinders, or rotary tools. Automated or semi-automated methods are less common but developing.
- Wyzwania: Support removal can be labor-intensive, time-consuming, and risks damaging delicate features (like clasps) or marring critical surfaces if not done skillfully. Design for easy support removal (DfAM) is key. The small contact points used in optimized supports help minimize surface blemishes.
- Proces: Once heat-treated (ideally), the build plate is removed from the printer. The frameworks are then separated from the plate, typically using:
- Surface Cleaning and Texturing:
- Sandblasting / Grit Blasting: Typically performed after support removal using abrasive media (e.g., aluminum oxide, glass beads) under high pressure.
- Cel: Removes any remaining partially sintered powder particles, eliminates minor surface imperfections left by supports, creates a uniform matte surface texture, and can improve bonding surfaces for subsequent layers (e.g., porcelain). The choice of media and pressure affects the resulting roughness.
- Rozważania: Must be done carefully to avoid over-abrading thin sections or critical margins.
- Fitting, Grinding, and Smoothing:
- Cel: To refine the fit, smooth surfaces where supports were attached, adjust contours, and prepare for polishing.
- Proces: This involves skilled manual work using dental laboratory handpieces with various burs, stones, and abrasive wheels. Technicians check the fit against the model and make micro-adjustments as needed.
- Importance: Essential for achieving passive fit, removing any sharp edges, and ensuring patient comfort.
- CNC Machining (If Required):
- Cel: For achieving the highest level of precision on critical interfaces, particularly for implant-supported frameworks. Connection points to implant abutments or analogs often require tolerances tighter than achievable directly via printing (±10−20μm).
- Proces: The printed and heat-treated framework is mounted in a high-precision CNC milling machine, and the critical interfaces are milled to exact specifications based on the original CAD data.
- Rozważania: Adds cost and complexity but may be necessary for demanding implant applications to guarantee passive fit. Requires careful fixture design.
- Polerowanie:
- Cel: To achieve a smooth, high-luster finish on external surfaces for aesthetics, hygiene (reduced plaque accumulation), and patient comfort.
- Proces: A multi-stage process involving progressively finer abrasives (rubber wheels, brushes, polishing compounds) applied with manual handpieces or potentially mass finishing techniques like tumbling or electropolishing for certain applications.
- Areas: Typically applied to visible metal surfaces of RPDs, metal collars on crowns/bridges. Fitting surfaces are usually left blasted or minimally finished.
- Surface Treatments for Porcelain Bonding (PFM):
- Cel: To create a stable, adherent oxide layer on the CoCr substructure that promotes a strong chemical bond with the opaque porcelain layer.
- Proces: After final shaping and cleaning (often sandblasting), the framework undergoes a controlled oxidation cycle in a porcelain furnace (de-gassing or oxidation firing) at high temperatures (e.g., 950-980°C) before porcelain application begins. Specific protocols depend on the porcelain system used.
Impact and Supplier Capability:
The extent and complexity of post-processing significantly impact the final cost and lead time of the 3D printed dental framework. Each step requires specific equipment, consumables, and skilled labor. When selecting a metal AM service provider or a wholesale dental framework supplier, it’s crucial to assess their in-house post-processing capabilities. A partner offering a comprehensive suite of validated post-processing services, from heat treatment and CNC machining to expert polishing, ensures better quality control, potentially faster turnaround times, and a single point of accountability. Outsourcing different steps to various vendors increases logistical complexity and risks compromising quality. Dental procurement managers should prioritize suppliers who demonstrate robust, integrated post-processing workflows tailored for dental applications.
Navigating Challenges: Common Issues in 3D Printing CoCr Frameworks and Mitigation Strategies
While metal 3D printing offers transformative advantages for producing Cobalt-Chrome dental frameworks, the technology is not without its challenges. Achieving consistent, high-quality results requires a deep understanding of the SLM/DMLS process, meticulous attention to detail, and robust quality control measures. Awareness of potential pitfalls allows dental labs, manufacturers, and their B2B partners to implement effective mitigation strategies.
Common Challenges and How to Address Them:
- Warping and Distortion:
- Cause: Intense localized heating and rapid cooling during SLM create steep thermal gradients and internal stresses. As layers build up, these stresses can accumulate, causing the part (especially large, thin, or asymmetric frameworks like RPDs or long bridges) to warp or distort, pulling away from supports or deviating from the intended geometry. This is exacerbated after removal from the build plate if stress relief is inadequate.
- Mitigation Strategies:
- Optimized Orientation: Angling parts to reduce the cross-sectional area per layer can lower peak stresses.
- Robust Support Structures: Well-designed supports act as anchors and heat sinks, restraining the part and drawing heat away. Strategic placement is key.
- Optymalizacja parametrów procesu: Fine-tuning laser power, scan speed, and scan strategy (e.g., island scanning) can minimize localized heat buildup. Using reliable equipment with consistent energy delivery, like the systems developed by Met3dp, is beneficial.
- DfAM: Incorporating temporary stiffening features, avoiding large flat areas, and designing for thermal stability.
- Mandatory Stress Relief: Performing a validated stress relief heat treatment cycle before removing parts from the build plate is crucial.
- Support Removal Difficulties and Surface Damage:
- Cause: Supports need to be strong enough to prevent warping but easy enough to remove without excessive force or damaging the framework surface, especially near delicate clasps or margins. Poorly designed supports (too dense, wrong contact type, inaccessible locations) make removal laborious and risky.
- Mitigation Strategies:
- Intelligent Support Design (DfAM/Software): Utilizing minimal contact points (cone, perforated), angling supports for tool access, placing them on non-critical surfaces whenever possible.
- Appropriate Removal Tools: Using precise cutting tools (fine burs, discs, potentially wire EDM).
- Skilled Technicians: Experienced technicians are essential for careful and efficient support removal and subsequent surface finishing.
- Optymalizacja procesów: Some parameter adjustments can influence the strength of the bond between the support and the part.
- Surface Porosity or Imperfections:
- Cause:
- Gas Porosity: Trapped gas (e.g., Argon from the build chamber, or dissolved gases in the powder) forming small spherical pores during solidification.
- Lack-of-Fusion Porosity: Insufficient energy density leading to incomplete melting between layers or scan tracks, resulting in irregular-shaped voids. Can be caused by incorrect parameters, poor powder quality, or inconsistent energy delivery.
- Chropowatość powierzchni: As discussed earlier, inherent roughness and partially sintered particles adhering to down-facing or heavily supported surfaces.
- Mitigation Strategies:
- Validated Process Parameters: Ensuring sufficient energy density for full melting without overheating (which can increase gas porosity).
- High-Quality Powder: Using powder with high sphericity, controlled PSD, good flowability, and low gas content (e.g., from specialized suppliers like Met3dp) minimizes risks. Proper powder handling and storage are vital. Met3dp’s commitment to advanced powder production techniques like gas atomization ensures optimal powder characteristics.
- Controlled Atmosphere: Maintaining a high-purity inert gas environment (Argon) in the build chamber.
- Optimized Orientation & Supports: Minimizing down-facing surfaces where possible.
- Appropriate Post-Processing: Sandblasting removes loose particles; significant porosity often requires part rejection. Hot Isostatic Pressing (HIP) can close internal pores but is rarely used for dental frameworks due to cost and potential distortion.
- Cause:
- Powder Quality Management and Contamination:
- Cause: Metal powder quality can degrade over time with handling and reuse (exposure to atmosphere, slight changes in PSD due to sieving, potential for cross-contamination if multiple materials are used on the same machine without thorough cleaning). Contaminated or degraded powder leads to printing defects and inconsistent properties.
- Mitigation Strategies:
- Strict Powder Handling Protocols: Storing powder in sealed containers with inert gas or low humidity, minimizing exposure time.
- Regular Sieving: Removing oversized particles or spatters.
- Powder Lot Traceability & Testing: Monitoring powder properties (chemistry, PSD, flowability) over its lifecycle. Implementing a powder refresh strategy (mixing used powder with virgin powder).
- Dedicated Equipment (Ideal): Using machines dedicated to specific materials (especially biocompatible ones like CoCr or Ti) prevents cross-contamination. Thorough cleaning protocols if changing materials.
- Sourcing from Reliable Suppliers: Partnering with powder manufacturers like Met3dp who have robust quality control throughout their production process provides a strong starting point.
- Achieving Consistent Passive Fit:
- Cause: Despite AM’s high accuracy potential, minor deviations can still occur due to thermal effects, slight variations in powder layers, or post-processing steps. Ensuring consistent passive fit, especially for multi-unit implant cases requiring near-zero strain, remains a high-level challenge.
- Mitigation Strategies:
- Precision Across Workflow: High-accuracy scanning, meticulous digital design, calibrated high-resolution printers, validated parameters, controlled heat treatment, and precision machining (if needed) are all essential links in the chain.
- Verification Jigs: Using verification jigs to confirm implant interface positions before finalizing framework design.
- Multi-Part Frameworks (Sectioning): For very long-span or complex implant cases, sometimes designing the framework in sections that are printed and then post-joined (e.g., laser welding) can help manage cumulative tolerances, although this adds complexity.
- Robust Quality Control: Implementing rigorous dimensional checks (CMM, 3D scanning) on final parts.
Successfully navigating these challenges requires a combination of advanced technology, process expertise, material science understanding, and stringent quality control. Dental framework manufacturers and suppliers who invest in high-quality equipment (like Met3dp’s printers), premium materials, skilled personnel, and validated processes are best positioned to consistently overcome these hurdles and deliver superior CoCr dental frameworks that meet the demanding requirements of the dental industry.
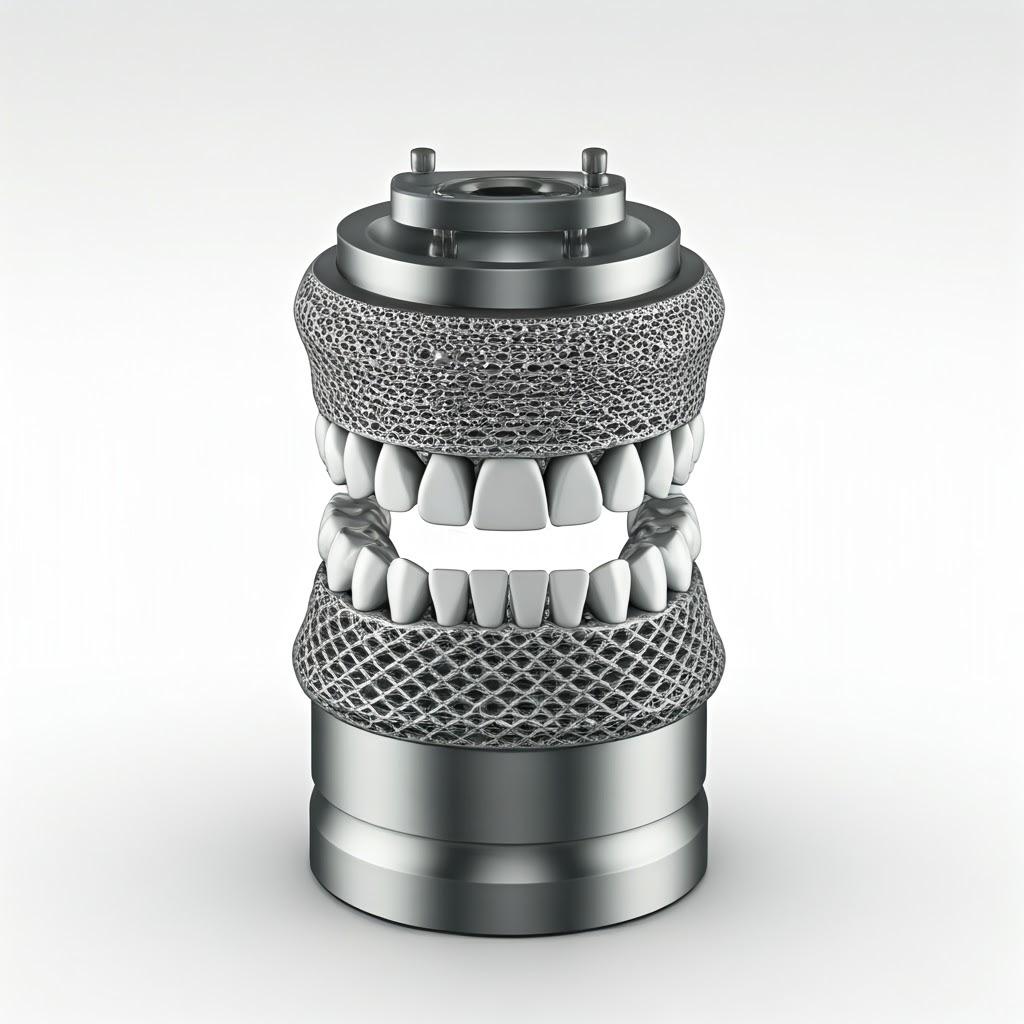
Supplier Selection: Choosing the Right Metal 3D Printing Service Provider for Dental Frameworks
Selecting the right partner for manufacturing 3D printed Cobalt-Chrome dental frameworks is a critical decision for dental laboratories, clinics, and wholesale dental distributors. The quality, accuracy, and reliability of the final product depend heavily on the capabilities, expertise, and quality systems of the chosen additive manufacturing service provider or contract manufacturer. Given the critical nature of dental devices, a thorough evaluation process is essential, going beyond just price considerations. Procurement managers and lab owners should look for partners who demonstrate excellence across several key areas.
Key Criteria for Evaluating Dental AM Suppliers:
- Dental-Specific Expertise and Experience:
- Importance: Dentistry has unique requirements regarding fit, function, biocompatibility, and aesthetics. A generic metal 3D printing service may not understand the nuances of dental anatomy, terminology, or clinical workflows.
- What to Look For: Proven track record in producing dental frameworks (RPDs, C&B substructures, implant bars). Staff with dental technology or biomedical engineering backgrounds. Understanding of dental CAD/CAM software and workflows. Case studies or examples of successful dental projects. Knowledge of specific challenges like achieving passive fit or optimizing porcelain bonding surfaces.
- Certifications and Quality Management System (QMS):
- Importance: Demonstrates a commitment to quality, safety, and regulatory compliance, crucial for medical/dental devices.
- What to Look For:
- Norma ISO 13485: Essential. This international standard specifies requirements for a QMS where an organization needs to demonstrate its ability to provide medical devices and related services that consistently 1 meet customer and applicable regulatory requirements. 2 Certification to ISO 13485 is a strong indicator of a reliable supplier for dental components. 1. medium.com medium.com 2. www.sec.gov www.sec.gov
- ISO 9001: A general standard for quality management systems, good to have but less specific than ISO 13485 for medical applications.
- Material Certifications: Evidence that the CoCr alloys used meet relevant standards (e.g., ASTM F75 or ISO 22674 for dental alloys).
- Material Quality Control and Traceability:
- Importance: Guarantees the use of appropriate, high-quality, biocompatible materials and allows for tracking in case of issues.
- What to Look For:
- Validated Powder Sources: Use of CoCrMo/CoCrW powders specifically designed and validated for dental AM from reputable manufacturers (demonstrating an understanding of powder’s critical role). Companies like Met3dp, specializing in high-performance metal powders produced via advanced methods like gas atomization, exemplify the type of quality focus required.
- Powder Batch Traceability: Systems to track which powder batch was used for which build and which parts.
- Powder Handling Procedures: Strict protocols for storage, handling, sieving, reuse, and testing of powders to prevent contamination and ensure consistency. Documentation of powder lifecycle management.
- Material Certificates: Ability to provide certificates of conformity (CoC) for the material used in specific batches upon request.
- Printer Technology and Process Validation:
- Importance: The quality and calibration of the SLM/DMLS machines directly impact part accuracy, density, and surface finish. Validated processes ensure repeatability.
- What to Look For:
- Industrial-Grade Equipment: Use of reputable, well-maintained SLM/DMLS systems known for stability and accuracy (e.g., systems capable of meeting the precision needs outlined earlier). Companies like Met3dp leverage their deep expertise by manufacturing their own industry-leading printers known for volume, accuracy, and reliability.
- Regular Calibration: Documented procedures for machine calibration (laser power, scanner accuracy, platform leveling).
- Validated Process Parameters: Established and validated parameter sets specifically for the CoCr alloys being used for dental applications, ensuring optimal density and properties.
- Environmental Controls: Build chambers with controlled inert atmospheres (Argon purity monitoring).
- Comprehensive In-House Post-Processing Capabilities:
- Importance: As detailed previously, post-processing is critical. A supplier offering integrated services ensures better control, consistency, and potentially faster turnaround.
- What to Look For:
- Validated Heat Treatment: Properly calibrated furnaces with controlled atmospheres and documented stress relief cycles specific to dental CoCr alloys.
- Skilled Finishing: Experienced technicians for support removal, surface finishing (blasting, polishing), and fitting.
- Precision Machining (if needed): In-house CNC machining capabilities for critical implant interfaces or margins.
- Obróbka powierzchni: Capability for specific treatments like oxidation firing for PFM applications.
- Capacity, Lead Times, and Responsiveness:
- Importance: Ability to meet required production volumes and delivery schedules is crucial for dental labs and distributors managing case workflows.
- What to Look For: Sufficient machine capacity, realistic and clearly communicated lead times (from file acceptance to shipping), responsive customer service and technical support for queries or issues. Flexibility to handle urgent cases (potentially at a premium).
- Technical Support and Design Consultation (DfAM):
- Importance: A valuable partner can assist with optimizing designs for additive manufacturing.
- What to Look For: Willingness to review designs, provide DfAM feedback, advise on optimal orientation and support strategies, and collaborate to solve potential manufacturing challenges. Access to knowledgeable engineers or technicians.
- Reputation and References:
- Importance: Past performance is often a good indicator of future reliability.
- What to Look For: Testimonials, case studies, references from other dental labs or industry partners. Positive standing within the dental manufacturing B2B network. Long-term relationships with clients. Explore the company’s history and expertise – for example, understanding a company’s background, like the information available on the Met3dp About Us page, can provide insights into their experience and commitment to the additive manufacturing field.
Evaluation Table Summary:
Criterion | Kluczowe czynniki | Why It Matters for Dental Frameworks |
---|---|---|
Dental Expertise | Industry knowledge, application experience, understanding clinical needs | Ensures functional, clinically relevant designs and solutions |
Certifications (ISO 13485) | QMS specifically for medical devices | Guarantees safety, quality, regulatory compliance |
Material Control | Validated powders, traceability, handling protocols, CoC availability | Ensures biocompatibility, correct properties, consistency |
Technology & Validation | Industrial printers, calibration, validated parameters, environmental control | Ensures accuracy, density, repeatability, optimal mechanical properties |
Przetwarzanie końcowe | In-house heat treatment, finishing, machining capabilities | Ensures final part quality, stability, function, faster turnaround |
Capacity & Lead Time | Production volume capability, reliable delivery schedules, responsiveness | Meets business needs, ensures timely case completion |
Technical Support & DfAM | Design review, optimization advice, problem-solving collaboration | Improves manufacturability, potentially reduces cost, enhances quality |
Reputation & References | Testimonials, case studies, industry standing | Builds trust and confidence in the supplier’s capabilities |
Export to Sheets
By systematically evaluating potential suppliers against these criteria, dental businesses can forge strong partnerships with capable metal AM providers who consistently deliver high-quality, precise, and reliable Cobalt-Chrome dental frameworks, ultimately contributing to better patient outcomes and enhancing their own operational efficiency.
Cost Analysis and Turnaround: Understanding Pricing and Lead Times for 3D Printed Dental Frameworks
While the clinical and technical benefits of 3D printed CoCr dental frameworks are clear, understanding the economic aspects – cost structure and production timelines – is crucial for business planning and decision-making in dental labs and procurement departments. Pricing for AM parts is influenced by a different set of factors compared to traditional casting, and lead times can offer significant advantages, particularly for complex cases.
Factors Influencing the Cost of 3D Printed CoCr Frameworks:
- Part Geometry and Volume (Bounding Box):
- Uderzenie: Larger or more complex parts occupy more space in the build chamber and generally require more material and longer print times. The overall height of the part on the build plate is a major driver of print time.
- Rozważania: Pricing is often related to the volume of the part’s bounding box (the smallest cuboid that encloses the part and its supports) as this represents machine utilization space. Complex designs requiring intricate supports also increase cost.
- Material Consumption:
- Uderzenie: The actual weight or volume of CoCrMo or CoCrW powder solidified to create the part and its support structures directly contributes to cost. CoCr alloys are relatively expensive precious/semi-precious metal powders.
- Rozważania: Efficient design (DfAM) aiming for lightweighting while maintaining strength, and minimizing support volume, helps control material costs. Powder cost per kilogram is a key input for suppliers. Wholesale dental framework pricing may reflect economies of scale in powder purchasing.
- Machine Print Time:
- Uderzenie: SLM/DMLS is a layer-by-layer process, and machine time is a significant cost factor, often charged per hour. Print time depends on the part height, total volume to be sintered, chosen layer thickness, and laser scanning parameters.
- Rozważania: Nesting multiple parts efficiently within a single build maximizes machine utilization and can reduce the per-part cost. Thicker layers print faster but reduce resolution and surface finish.
- Support Structure Requirements:
- Uderzenie: Supports consume material, add to print time, and require manual labor for removal and subsequent surface finishing. More extensive or complex supports directly increase cost.
- Rozważania: DfAM principles focused on self-supporting designs and optimizing orientation can significantly reduce support needs and associated costs.
- Post-Processing Intensity:
- Uderzenie: This is often a major cost component, sometimes equalling or exceeding the printing cost itself. Each step adds labor, equipment time, and consumable costs.
- Rozważania:
- Obróbka cieplna: Essential but requires furnace time and controlled atmosphere.
- Support Removal & Finishing: Labor-intensive, especially for complex geometries or high polish requirements.
- CNC Machining: Adds significant cost due to machine time, programming, and fixturing, but may be necessary for implant interfaces.
- Required Surface Finish: Achieving a mirror polish is far more time-consuming than a standard sandblasted finish.
- Quality Control and Inspection:
- Uderzenie: Basic visual inspection is standard. More rigorous requirements, such as CMM measurements for critical dimensions or 3D scanning for full geometry verification, add to the cost.
- Rozważania: The level of required documentation (e.g., detailed inspection reports, material certs) can also influence price.
- Order Quantity and Setup:
- Uderzenie: There’s typically a setup cost associated with preparing a build file, loading the machine, and initiating a print run. Spreading this setup cost over a larger number of identical or similar parts reduces the per-unit price.
- Rozważania: Significant price breaks are often available for higher volume orders, relevant for wholesale dental suppliers or large labs ordering in bulk. Rush orders typically incur premium charges.
Cost Factor Summary Table:
Cost Driver | Primary Influence | How to Potentially Mitigate |
---|---|---|
Part Volume/Size | Machine space utilization, print time | Optimized orientation, efficient nesting |
Złożoność części | Design difficulty, support needs, finishing effort | DfAM for manufacturability, simplify non-critical features |
Material Consumed | Powder cost (CoCr alloy), part weight, support volume | DfAM for lightweighting, minimize supports |
Machine Print Time | Part height, total sintered volume, layer thickness | Efficient nesting, optimize parameters (balance speed/quality) |
Struktury wsparcia | Material usage, print time addition, removal labor | DfAM for self-supporting angles, optimize support design |
Przetwarzanie końcowe | Heat treatment, labor (removal, finishing), machining needs | Design for easier finishing, specify only necessary finish levels |
Quality Requirements | Inspection level (visual, CMM, scan), documentation needs | Define clear, appropriate QA specifications |
Wielkość zamówienia | Setup cost amortization, potential material/process efficiencies | Place larger orders for better unit pricing (wholesale benefits) |
Export to Sheets
Lead Time Considerations:
- Typical Turnaround: For standard CoCr dental frameworks, lead times from file submission to shipment can range from 3 to 7 business days, depending on complexity, quantity, required post-processing, and the service provider’s workload. Simpler substructures might be faster, while complex RPDs or implant bars requiring machining could take longer. (Note: Current date is April 22, 2025; these are general estimates and subject to change based on market conditions.)
- Comparison to Casting: While a single casting might theoretically be faster if the wax-up is ready, the overall AM workflow (digital design -> print -> post-process) is often more predictable and can be significantly faster for multiple units printed simultaneously or for complex cases that would require multiple casting/joining steps traditionally. The digital nature eliminates physical model shipping time in many workflows.
- Factors Influencing Lead Time: Part complexity, number of units, nesting efficiency, required post-processing steps (especially heat treatment and extensive polishing/machining), machine availability, and shipping logistics.
Understanding these cost factors and lead time dynamics allows dental businesses to accurately budget for 3D printed frameworks, compare quotes effectively, and manage expectations for case completion. While the per-unit cost might sometimes appear higher than traditional casting at first glance, considering reduced chair-side adjustment time, improved fit, potentially longer lifespan, and faster turnaround for complex cases often reveals a favorable total cost of ownership and significant clinical advantages.
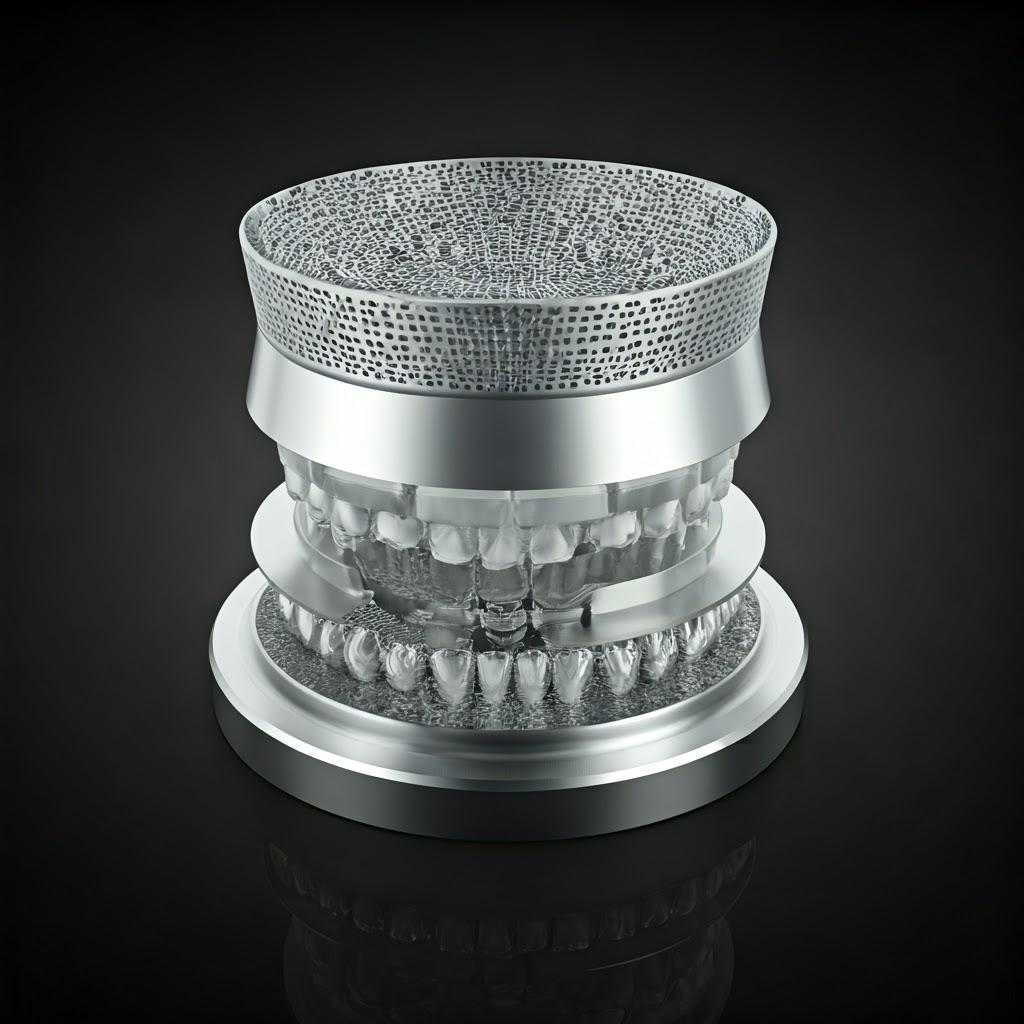
Frequently Asked Questions (FAQ) about 3D Printed CoCr Dental Frameworks
Here are answers to some common questions that dental professionals, lab owners, and procurement managers may have regarding 3D printed Cobalt-Chrome dental frameworks:
- Is 3D printed Cobalt-Chrome (CoCrMo/CoCrW) biocompatible and safe for long-term use in the mouth?
- Odpowiedź: Yes, absolutely. CoCr alloys, specifically those conforming to standards like ASTM F75 or ISO 22674, have a long history of safe use in both medical implants (like hip replacements) and dental restorations. When processed correctly using validated additive manufacturing parameters and high-purity powders, 3D printed CoCr frameworks exhibit excellent biocompatibility. Reputable manufacturers conduct testing (based on ISO 10993 standards) to verify factors like cytotoxicity, sensitization, and ion release, ensuring the final components are safe for intraoral use. Always ensure your supplier uses certified materials and ideally operates under an ISO 13485 quality management system.
- How does the strength and durability of 3D printed CoCr frameworks compare to traditionally cast frameworks?
- Odpowiedź: 3D printed CoCr frameworks generally exhibit mechanical properties (like yield strength, ultimate tensile strength, and hardness) that are comparable to or even exceed those of cast CoCr frameworks. The rapid solidification during SLM/DMLS creates a very fine-grained microstructure, which can enhance strength. Furthermore, AM can achieve near-full density (>99.5%), minimizing the internal porosity that can sometimes weaken cast parts. With proper design (DfAM) and mandatory post-print stress relief heat treatment, 3D printed frameworks are highly durable and suitable for demanding long-term applications like RPDs and long-span bridges.
- Can existing dental CAD files (.STL format) designed for casting or milling be used directly for 3D printing?
- Odpowiedź: Often, yes, but typically require review and potential modification. Most dental CAD software exports designs in .STL format, which is the standard input for AM processes. However, a design optimized for casting (e.g., thicker connectors, specific sprue points assumed) may not be ideal for 3D printing. It might require adjustments based on DfAM principles – checking minimum wall thicknesses, optimizing orientation, adding supports, and potentially modifying features to leverage AM’s capabilities or avoid printing issues. Collaboration with the AM service provider during the build preparation phase is recommended to ensure the file is suitable and optimized for printing.
- What is the typical clinical lifespan of a 3D printed CoCr dental framework?
- Odpowiedź: When properly designed, manufactured using quality materials and processes, and integrated into a well-made final restoration (RPD, crown, bridge), 3D printed CoCr frameworks are expected to have a clinical lifespan similar to or potentially exceeding that of high-quality cast frameworks. Their high strength, corrosion resistance, excellent fit (reducing stress and potential leakage), and material homogeneity contribute to long-term stability and durability. Like any dental restoration, the lifespan also depends on factors like patient oral hygiene, biting forces, and regular dental check-ups. Decades of successful clinical use can be reasonably expected.
- Are there different grades or types of CoCrMo/CoCrW powder available from suppliers for dental applications?
- Odpowiedź: Yes, while most dental CoCr powders fall under the general CoCrMo or CoCrW classifications (often adhering to standards like ASTM F75), there can be minor variations in composition offered by different powder manufacturers. Some may offer slight tweaks in Molybdenum, Tungsten, Carbon, or Nitrogen content, potentially marketed as offering slightly enhanced strength, hardness, or porcelain bonding characteristics. More significantly, powder quality itself varies greatly between suppliers regarding particle size distribution, sphericity, flowability, purity, and gas content. Choosing a supplier like Met3dp, known for using advanced atomization techniques (Gas Atomization, PREP) and stringent quality control, ensures access to high-performance powders optimized specifically for demanding applications like dental additive manufacturing. It’s crucial to work with an AM provider who uses powders validated for dental use and can provide documentation regarding its properties and compliance.
Conclusion: Embracing Additive Manufacturing for Superior Cobalt-Chrome Dental Frameworks
The integration of metal additive manufacturing into restorative dentistry marks a significant leap forward, fundamentally changing how high-performance Cobalt-Chrome dental frameworks are produced. As we’ve explored, the move from traditional lost-wax casting to 3D printing technologies like Selective Laser Melting (SLM) offers a cascade of compelling advantages that benefit dental laboratories, clinicians, wholesale suppliers, and ultimately, patients seeking durable and precise restorations.
The ability to translate intricate digital designs directly into metal with micron-level accuracy revolutionizes fit, particularly for complex RPDs and multi-unit implant-supported structures where achieving passive fit is paramount. This precision minimizes chair-side adjustments, saving valuable clinical time and improving patient comfort. Furthermore, the design freedom inherent in AM empowers dental technicians to create optimized frameworks with anatomically contoured clasps, ideal connector dimensions, and uniform thicknesses previously constrained by casting limitations.
Leveraging high-quality CoCrMo and CoCrW powders, specifically engineered for additive manufacturing with controlled sphericity, particle size, and purity, ensures the resulting frameworks exhibit exceptional mechanical properties – often matching or exceeding cast components – along with the proven biocompatibility and corrosion resistance expected of dental Cobalt-Chrome. The digital workflow enhances efficiency, reduces material waste, and provides unparalleled consistency and repeatability, crucial factors for high-volume dental labs and B2B dental framework distributors aiming for predictable quality.
While challenges related to design optimization (DfAM), meticulous post-processing (especially heat treatment), and rigorous quality control exist, they are readily overcome through expertise, investment in quality systems, and strong partnerships. Choosing the right AM service provider – one with dental-specific knowledge, essential certifications like ISO 13485, robust material controls, validated processes, and comprehensive post-processing capabilities – is key to harnessing the full potential of this technology.
The future of digital dentistry clearly points towards increased adoption of additive manufacturing. As the technology continues to mature, with ongoing advancements in materials, machinery speed, and process automation, the production of dental frameworks will become even more efficient and accessible.
For dental laboratories seeking to enhance precision and efficiency, prosthodontists demanding the highest quality restorations, and dental procurement managers evaluating next-generation manufacturing solutions, metal 3D printing of Cobalt-Chrome frameworks presents an undeniable value proposition. It represents a convergence of material science, digital technology, and advanced manufacturing that elevates the standard of care.
We encourage you to explore how metal additive manufacturing can transform your dental framework production. Partnering with a knowledgeable and experienced provider is the first step. Companies like Met3dp, with deep expertise spanning advanced metal AM systems, high-performance powders, and application development, stand ready to assist businesses in navigating and implementing this powerful technology to achieve their manufacturing goals and deliver superior dental solutions.
Udostępnij
Facebook
Twitter
LinkedIn
WhatsApp
E-mail
MET3DP Technology Co., LTD jest wiodącym dostawcą rozwiązań w zakresie produkcji addytywnej z siedzibą w Qingdao w Chinach. Nasza firma specjalizuje się w sprzęcie do druku 3D i wysokowydajnych proszkach metali do zastosowań przemysłowych.
Zapytaj o najlepszą cenę i spersonalizowane rozwiązanie dla Twojej firmy!
Powiązane artykuły
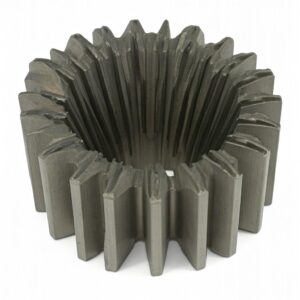
High-Performance Nozzle Vane Segments: Revolutionizing Turbine Efficiency with Metal 3D Printing
Czytaj więcej "
May 13, 2025
Brak komentarzy
Informacje o Met3DP
Ostatnia aktualizacja
Nasz produkt
KONTAKT
Masz pytania? Wyślij nam wiadomość teraz! Po otrzymaniu wiadomości obsłużymy Twoją prośbę całym zespołem.
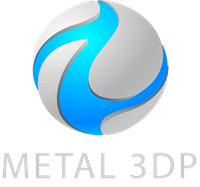
Proszki metali do druku 3D i produkcji addytywnej
PRODUKT
cONTACT INFO
- Miasto Qingdao, Shandong, Chiny
- [email protected]
- [email protected]
- +86 19116340731