3D Printed Cooling Tube Manifolds for Electric Vehicles
Inhaltsübersicht
Introduction: Revolutionizing EV Thermal Management with 3D Printed Cooling Manifolds
The relentless acceleration of the electric vehicle (EV) market is driving unprecedented innovation across the automotive supply chain. Engineers and procurement managers face the constant challenge of developing vehicles that are not only efficient and powerful but also reliable and cost-effective to manufacture. Central to achieving these goals is effective thermal management. The battery pack, electric motors, and power electronics in an EV generate significant heat during operation. Managing this heat effectively is crucial for ensuring optimal performance, maximizing battery lifespan, preventing component degradation, and guaranteeing passenger safety. Traditional cooling systems, often constrained by conventional manufacturing methods like casting, extrusion, and welding, struggle to meet the increasingly complex demands of modern EV architectures. This is where Additive Fertigung von Metall (AM), oder 3D-Druck, emerges as a transformative technology.
Metal 3D printing allows for the creation of highly complex, optimized, and integrated components that were previously impossible or prohibitively expensive to produce. For EV thermal management, this translates into the ability to design and manufacture advanced cooling tube manifolds with intricate internal channels, optimized flow paths, and consolidated functionalities. These aren’t just incremental improvements; they represent a fundamental shift in how we approach the design and production of critical automotive fluid systems. By leveraging the design freedom offered by AM, engineers can create manifolds that are lighter, package more efficiently within the constrained spaces of an EV chassis, and deliver superior cooling performance compared to their traditionally manufactured counterparts.
This blog post delves into the specifics of using metal 3D printing to produce EV cooling tube manifolds, focusing on the benefits, materials, design considerations, and supplier selection process. We will explore why this technology is rapidly becoming a preferred solution for leading automotive manufacturers and Tier 1 suppliers seeking a competitive edge. Furthermore, we will highlight how partnering with an experienced 3D-Druck von Metall provider like Met3dp, with its advanced powder manufacturing and printing capabilities, can de-risk adoption and accelerate the integration of this cutting-edge technology into your EV production workflow. Whether you are an engineer designing next-generation battery cooling systems or a procurement manager sourcing high-performance automotive components, understanding the potential of 3D printed cooling manifolds is essential for staying ahead in the dynamic EV landscape.
What is an EV Cooling Tube Manifold and Its Critical Function?
An EV cooling tube manifold is a critical component within the vehicle’s thermal management system, acting as the central hub for distributing coolant fluid to various heat-generating sources and routing it back to the heat exchanger (radiator or chiller). Its primary function is to ensure that key components, particularly the battery pack and elements of the powertrain (like the motor and inverter), operate within their optimal temperature range.
Key Functions and Importance:
- Coolant Distribution: The manifold receives coolant from the pump and precisely distributes it through a network of tubes or channels to specific areas requiring cooling. This might involve directing flow to individual battery modules, sections of the motor housing, or power electronics cooling plates.
- Temperature Regulation: By ensuring consistent and adequate coolant flow, the manifold plays a direct role in regulating the temperature of critical components. Maintaining stable temperatures is vital for:
- Battery Performance & Longevity: Extreme temperatures (both hot and cold) drastically reduce battery capacity, lifespan, and charging speed. Effective cooling prevents overheating during high-load situations (fast charging, aggressive driving) and potentially heating in cold climates.
- Powertrain Efficiency: Electric motors and power electronics operate most efficiently within specific temperature windows. Overheating can lead to reduced power output, decreased efficiency, and potential damage.
- Sicherheit: Preventing thermal runaway in battery packs is a paramount safety concern. Robust cooling systems, orchestrated by the manifold, are a key line of defense.
- Fluid Collection and Return: After absorbing heat, the coolant flows back through the manifold, which directs it towards the radiator or chiller to dissipate the heat before the cycle repeats.
- System Integration: Manifolds often serve as integration points for sensors (temperature, pressure), valves, and connectors, simplifying the overall assembly and reducing potential leak points compared to systems with numerous separate fittings and hoses.
Typical Location and Design:
Cooling manifolds are typically located strategically within the vehicle, often close to the battery pack or integrated into the powertrain assembly to minimize tubing length and pressure drop. Their design can vary significantly based on the specific EV architecture:
- Battery Cooling: Manifolds might be integrated directly into the battery pack enclosure, distributing coolant to cold plates situated beneath or between battery modules.
- Powertrain Cooling: Separate or integrated manifolds may serve the electric motor(s) and power electronics (inverter, converter).
- Integrated Systems: Increasingly, designs aim for integrated thermal management systems where a single, complex manifold might serve multiple components.
Traditionally, these manifolds were often assemblies of bent tubes, cast housings, and multiple connectors. However, the complex packaging constraints and the need for optimized flow paths in modern EVs often make these traditional approaches bulky, heavy, and less efficient. This complexity and the critical nature of their function make EV cooling tube manifolds prime candidates for the advanced capabilities offered by metal additive manufacturing. Procurement managers looking for reliable automotive fluid systems suppliers und electric vehicle component manufacturers are increasingly turning to AM specialists to meet these demanding requirements.
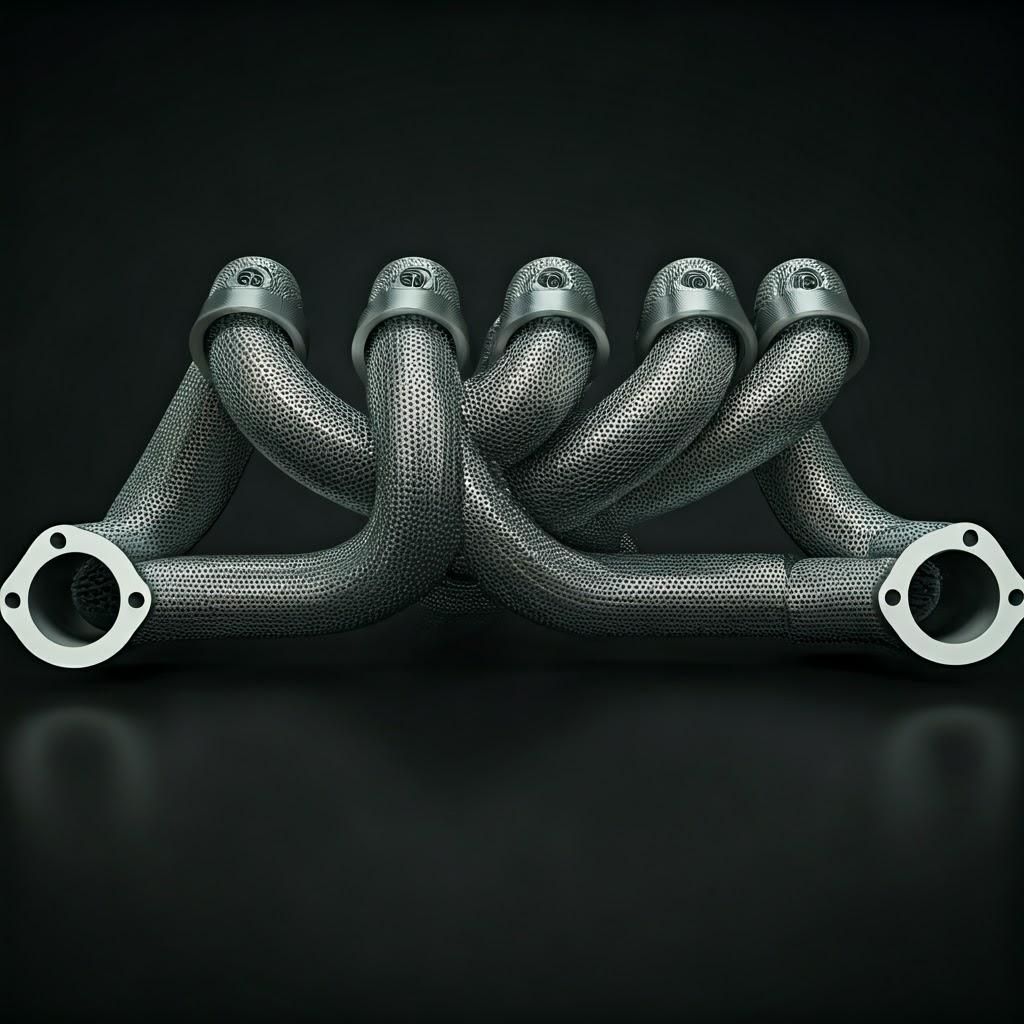
Why Choose Metal 3D Printing for EV Cooling Manifold Production?
While traditional manufacturing methods like casting, machining, and tube bending have long served the automotive industry, metal additive manufacturing offers compelling advantages specifically tailored to the challenges of producing high-performance EV cooling tube manifolds. These benefits address key industry drivers such as performance optimization, lightweighting, packaging efficiency, and accelerated development cycles.
Key Advantages of Metal AM for Cooling Manifolds:
- Unerreichte Gestaltungsfreiheit und Komplexität:
- Intricate Internal Channels: AM allows the creation of complex, non-linear internal cooling channels optimized for fluid dynamics (e.g., minimizing pressure drop, maximizing surface area for heat exchange) that are impossible to achieve with drilling or casting. Features like internal fins, turbulators, or variable channel diameters can be directly printed.
- Organic Shapes & Topology Optimization: Engineers can use topology optimization and generative design tools to create lightweight yet strong manifolds that perfectly conform to available packaging space within the vehicle chassis. This results in organic, load-path-optimized structures.
- Teil Konsolidierung: Multiple components (e.g., brackets, sensor bosses, inlet/outlet ports, multiple tubes) can be integrated into a single, monolithic 3D printed manifold. This drastically reduces part count, assembly time, weight, and potential leak points associated with joints and seals.
- Verbesserte Leistung:
- Optimized Flow Distribution: The design freedom enables precise control over coolant flow to different areas, ensuring uniform temperature distribution across battery modules or targeted cooling for specific hotspots in the motor or inverter.
- Improved Thermal Efficiency: Complex internal geometries and the ability to use high-thermal-conductivity materials (like Copper alloys) more effectively lead to more efficient heat transfer compared to bulkier, less optimized traditional designs.
- Gewichtsreduzierung:
- Material Reduction: Topology optimization removes material from non-critical areas, resulting in significantly lighter components compared to cast or machined parts designed with traditional constraints. Weight reduction is paramount in EVs to maximize range and performance.
- Use of Lightweight Alloys: AM facilitates the use of high-strength, lightweight alloys like AlSi10Mg, further contributing to overall vehicle weight reduction.
- Accelerated Development & Prototyping:
- Schnelle Iteration: New manifold designs can be printed and tested in days or weeks, compared to the months often required for tooling development in casting or complex fixtures for fabrication. This accelerates the design validation and overall vehicle development timeline.
- Beseitigung von Werkzeugen: AM is a tool-less process, eliminating the significant cost and lead time associated with creating molds, dies, or complex jigs. This is particularly advantageous for low-to-mid volume production runs or customized designs.
- Supply Chain Flexibility & On-Demand Production:
- Reduzierte Bestände: Parts can be printed on demand, reducing the need for large inventories of specialized manifolds.
- Verteilte Fertigung: Production can potentially be decentralized, improving supply chain resilience. Wholesale buyers and distributors benefit from faster access to customized or low-volume, high-performance parts without traditional tooling barriers.
Comparison: Traditional vs. Additive Manufacturing for EV Cooling Manifolds
Merkmal | Traditional Manufacturing (Casting, Fabrication) | Additive Fertigung von Metallen (z. B. LPBF) | Vorteil von AM |
---|---|---|---|
Entwurfskomplexität | Limited internal channels, simpler geometries | Highly complex internal channels, organic shapes | Optimized flow, better packaging, part consolidation |
Teil Konsolidierung | Difficult, often requires multiple parts/assembly | Easily integrates multiple features into one part | Reduced weight, fewer leak points, simplified assembly |
Gewichtsreduzierung | Constrained by process limitations | Enables topology optimization, significant potential | Improved vehicle range and performance |
Materielle Verwendung | Can be wasteful (machining), process-limited | Optimized material usage, near-net shape | Reduced material cost and waste |
Werkzeugbau | Required (molds, dies, jigs) – costly & slow | Tool-less | Faster time-to-first-part, economical for low/mid volumes, rapid iteration |
Vorlaufzeit (Proto) | Wochen bis Monate | Tage bis Wochen | Accelerated development cycles |
Leistung | Good, but often compromised by manufacturability | Potentially superior due to design optimization | Enhanced thermal efficiency, uniform cooling |
Ideales Volumen | High Volume | Low-to-Mid Volume, Complex Parts, Prototypes | Flexibility for niche vehicles, performance upgrades, initial production ramps |
In Blätter exportieren
Companies like Met3dp, specializing in advanced metal powders and industrial printing systems like Selective Electron Beam Melting (SEBM) and Laser Powder Bed Fusion (LPBF), are enabling automotive manufacturers and Tier 1 automotive suppliers to harness these advantages effectively. Their expertise ensures that the potential of AM is translated into reliable, high-performance components ready for demanding EV applications.
Material Selection: Optimizing Performance with CuCrZr and AlSi10Mg Powders
Choosing the right material is paramount to the success of any 3D printed component, especially for a functionally critical part like an EV cooling tube manifold. The material must possess the necessary thermal conductivity, mechanical strength, corrosion resistance, and compatibility with automotive coolants, all while being processable via metal additive manufacturing. For EV cooling applications, two materials stand out due to their excellent balance of properties: Copper-Chromium-Zirconium (CuCrZr) und Aluminum-Silicon-Magnesium (AlSi10Mg).
1. Copper-Chromium-Zirconium (CuCrZr): The High-Performance Choice
- Überblick: CuCrZr is a precipitation-hardenable copper alloy renowned for its exceptional combination of high thermal and electrical conductivity, good mechanical strength (especially at elevated temperatures), and resistance to softening.
- Key Properties & Benefits for EV Cooling Manifolds:
- Ausgezeichnete Wärmeleitfähigkeit: Copper alloys inherently offer superior thermal conductivity compared to aluminum or steels (~300-380 W/m·K for printed and heat-treated CuCrZr). This allows for highly efficient heat transfer, enabling smaller or more effective cooling systems.
- Good Mechanical Strength: While not as strong as steels, heat-treated CuCrZr provides sufficient strength and fatigue resistance for the pressures and vibrations encountered in automotive coolant systems. It maintains strength well at moderately elevated temperatures found in powertrain cooling loops.
- Korrosionsbeständigkeit: Exhibits good resistance to corrosion from typical automotive coolant mixtures (glycol/water).
- Stress Relaxation Resistance: Important for maintaining clamping forces and sealing integrity over the vehicle’s lifetime.
- Why Use AM for CuCrZr? Manufacturing complex CuCrZr parts traditionally is challenging. AM allows the intricate geometries needed for manifolds to be realized directly, leveraging the material’s high conductivity in optimized designs.
- Herausforderungen: Printing copper alloys can be more challenging than aluminum or steel due to copper’s high reflectivity and thermal conductivity, requiring optimized process parameters (laser power, scan speed) often found in specialized systems. Post-processing, including specific heat treatments (solutionizing and aging), is crucial to achieve the desired mechanical properties.
2. Aluminum-Silicon-Magnesium (AlSi10Mg): The Lightweight Workhorse
- Überblick: AlSi10Mg is one of the most common and well-understood aluminum alloys used in additive manufacturing. It’s essentially a casting alloy adapted for AM processes like Laser Powder Bed Fusion (LPBF).
- Key Properties & Benefits for EV Cooling Manifolds:
- Ausgezeichnetes Verhältnis von Stärke zu Gewicht: Aluminum alloys are significantly lighter than copper or steel, contributing directly to vehicle lightweighting and improved range. AlSi10Mg offers good mechanical properties after heat treatment.
- Gute Wärmeleitfähigkeit: While lower than CuCrZr, AlSi10Mg still possesses good thermal conductivity (typically 100-130 W/m·K after stress relief), sufficient for many battery and some powertrain cooling applications.
- Excellent Processability: AlSi10Mg is relatively easy to process using LPBF systems, with well-established parameters leading to dense, reliable parts.
- Korrosionsbeständigkeit: Generally good resistance to automotive coolants, similar to cast aluminum components commonly used in engines and cooling systems.
- Kosten-Nutzen-Verhältnis: Aluminum powder is typically less expensive than specialized copper alloys, and processing is often faster, making it a more cost-effective solution for many applications.
- Why Use AM for AlSi10Mg? AM unlocks the ability to create highly complex, lightweight AlSi10Mg manifolds that would be difficult or impossible to cast or machine, maximizing the benefits of this versatile alloy.
- Herausforderungen: Requires appropriate heat treatment (typically stress relief or T6) to achieve optimal mechanical properties and dimensional stability. While processable, ensuring leak-tight integrity in thin-walled, complex structures still requires careful process control.
Material Property Comparison:
Eigentum | CuCrZr (Heat Treated) | AlSi10Mg (Heat Treated – T6) | Einheit | Significance for EV Cooling Manifold |
---|---|---|---|---|
Dichte | ~8.9 | ~2.67 | g/cm³ | AlSi10Mg offers significant lightweighting potential. |
Wärmeleitfähigkeit | ~300 – 380 | ~120 – 140 | W/m-K | CuCrZr enables higher thermal efficiency or more compact designs. |
Streckgrenze (Rp0,2) | ~350 – 450 | ~230 – 280 | MPa | Both offer sufficient strength; CuCrZr better at higher temps. |
Tensile Strength (Rm) | ~450 – 550 | ~330 – 400 | MPa | Measures resistance to breaking under tension. |
Elongation at Break (A) | ~10 – 20 | ~3 – 10 | % | Indicates ductility; important for fatigue and impact resistance. |
Processability (LPBF) | More Challenging | Well-Established | – | Affects print success rate, parameter development, and cost. |
Relative Kosten | Höher | Unter | – | Significant factor in component and overall vehicle cost. |
In Blätter exportieren
Der Met3dp-Vorteil:
Choosing the right material powder is as critical as selecting the alloy itself. The quality, sphericity, particle size distribution, and purity of the metal powder directly impact the final part’s density, mechanical properties, and surface finish. Met3dp, leveraging its fortschrittliche Systeme zur Pulverherstellung including gas atomization and Plasma Rotating Electrode Process (PREP) technologies, produces high-quality spherical metal powders optimized for AM processes. Their portfolio includes standard alloys like AlSi10Mg and extends to specialized materials, potentially including copper alloys suitable for demanding applications like CuCrZr. Partnering with a provider like Met3dp, who controls both the hochwertige Metallpulver and possesses deep expertise in printing process parameters, ensures that the selected material delivers its maximum performance potential in the final 3D printed EV cooling manifold. They act as a reliable automotive material supplier specializing in powders specifically designed for additive manufacturing, ensuring consistency and quality for wholesale buyers and large-scale production needs. Sources and related content
Design for Additive Manufacturing (DfAM) for Optimal Cooling Manifold Performance
Simply replicating a traditionally designed manifold using 3D printing often fails to capture the full potential of additive manufacturing. To truly leverage the benefits of complexity, part consolidation, and lightweighting, engineers must embrace Design für additive Fertigung (DfAM) principles. DfAM is not just about making a part printable; it’s about fundamentally rethinking the design approach to maximize performance, minimize cost, and exploit the unique capabilities of the AM process. For EV cooling tube manifolds, applying DfAM is crucial for achieving superior thermal performance and efficient packaging.
Key DfAM Considerations for EV Cooling Manifolds:
- Optimierung der Fließwege:
- CFD-Driven Design: Utilize Computational Fluid Dynamics (CFD) simulation early and often in the design process. This allows engineers to model coolant flow, identify potential stagnation zones or areas of high-pressure drop, and iteratively refine internal channel geometry for optimal thermal performance and minimal pumping losses.
- Smooth Bends & Transitions: Avoid sharp corners in internal channels. Use generous radii for bends and smooth transitions between different channel cross-sections to minimize flow separation and pressure drop. AM easily accommodates complex, curved pathways.
- Variable Cross-Sections: Design channels that vary in size or shape along their length to control flow velocity and optimize heat transfer where it’s needed most.
- Interne Merkmale: Incorporate features like internal fins, dimples, or lattice structures directly into the channel walls to enhance turbulence and increase the surface area for heat exchange, boosting thermal efficiency without significantly increasing overall size.
- Part Consolidation & Feature Integration:
- Combine Multiple Functions: Identify opportunities to merge adjacent components (brackets, mounting points, sensor housings, connectors) into the single manifold structure. This reduces part count, assembly labor, weight, and potential leak paths.
- Integrated Mounts & Interfaces: Design mounting bosses, flanges, and connection ports directly into the manifold body, ensuring they are robust and positioned optimally. Consider the accessibility needed for fasteners during vehicle assembly.
- Strategien zur Gewichtsreduzierung:
- Topologie-Optimierung: Employ software tools to identify and remove material from areas not critical to the manifold’s structural integrity or fluid-handling function. This often results in organic, bone-like structures that are significantly lighter than solid designs but maintain the required stiffness and strength.
- Gitterförmige Strukturen: For non-load-bearing sections or internal supports, consider using lattice structures to reduce material usage and weight while maintaining geometric stability.
- Thin-Walled Design: Leverage AM’s ability to create strong, thin-walled structures. However, ensure wall thickness is sufficient to withstand operating pressures, resist damage during handling/installation, and allow for reliable printing without defects (minimum printable wall thickness depends on the machine, material, and orientation).
- Manufacturability Considerations (Process-Specific):
- Selbsttragende Winkel: Design overhangs and internal channels with angles relative to the build plate that minimize the need for support structures (typically >45 degrees from horizontal for LPBF). Extensive internal supports can be very difficult or impossible to remove completely.
- Entfernung von Puder: Critically important for manifolds. Ensure internal channels have adequate access points or escape holes for removing unfused metal powder after printing. Design channels to avoid sharp corners or dead ends where powder can become trapped. Consider flow-through designs where possible.
- Orientation Strategy: Plan the part’s orientation on the build plate early in the design phase. Orientation affects surface finish on different faces, support requirements, residual stress accumulation, and potentially mechanical properties due to anisotropy.
- Minimale Featuregröße: Be aware of the minimum printable feature size (holes, wall thickness, ribs) achievable with the chosen AM process and material.
DfAM Workflow Example:
- Definieren Sie Anforderungen: Specify operating pressures, flow rates, temperature targets, packaging constraints, mounting points, and material choice.
- Initial Concept & Simulation: Develop an initial design concept, possibly based on a traditional layout, and run CFD analysis to establish a baseline performance.
- Topology Optimization/Generative Design: Use optimization tools, inputting load cases (pressure, vibration, mounting forces) and design space constraints, to generate a lightweight, structurally efficient form.
- Detailed Design & Channel Refinement: Refine the optimized geometry, focusing on smoothing internal flow paths, integrating features, and ensuring DfAM manufacturability (powder removal, support minimization). Re-run CFD to verify performance improvements.
- Printability Check & Support Strategy: Perform a final check for minimum wall thickness, self-supporting angles, and trapped volumes. Plan the support structure strategy.
- Prototyping & Testing: Print prototypes for validation, including flow testing, pressure testing, and fit checks. Iterate as needed.
Implementing DfAM requires a shift in mindset and often collaboration between design engineers and AM specialists. Companies like Met3dp, with extensive experience in various Druckverfahren and materials like AlSi10Mg and CuCrZr, can provide invaluable DfAM guidance. Their engineers understand the nuances of powder bed fusion processes and can help optimize manifold designs not just for performance but also for efficient and reliable additive production, ensuring that procurement managers receive parts that meet stringent automotive requirements.
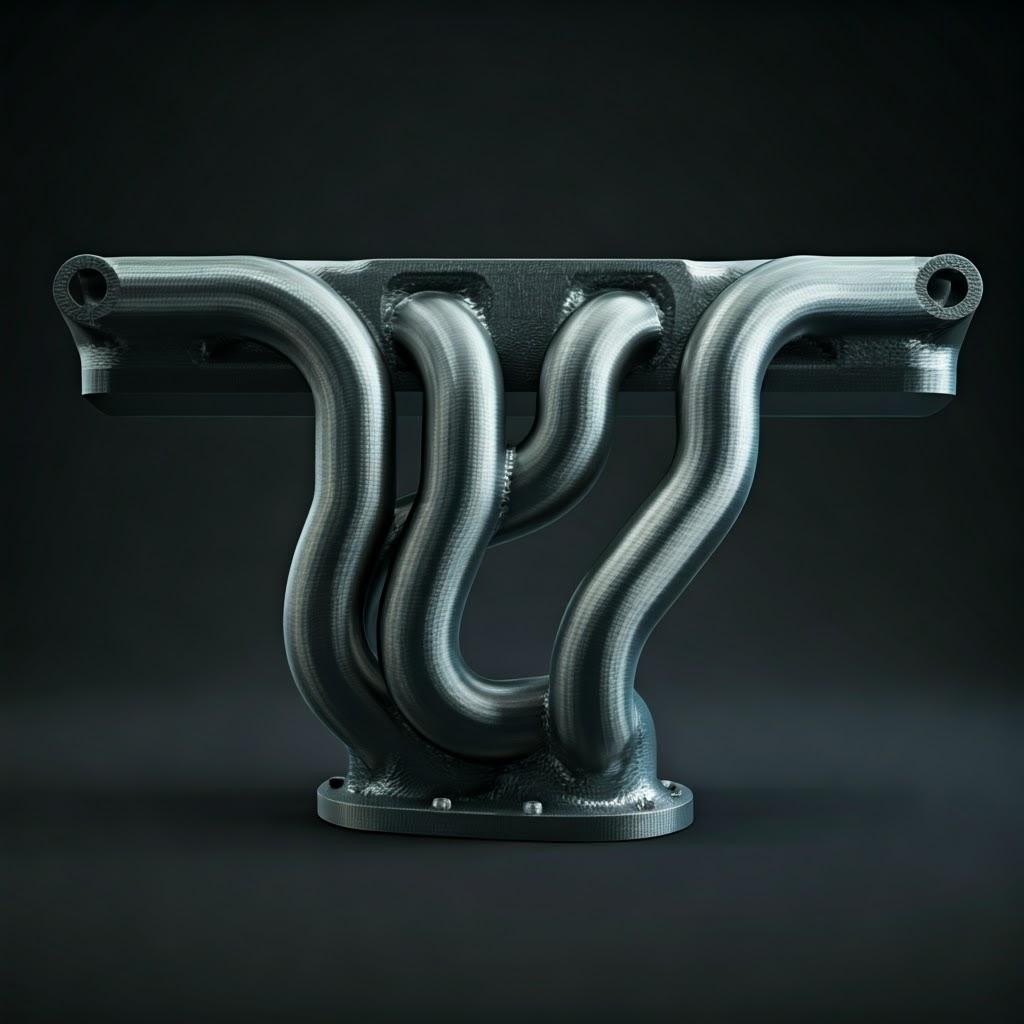
Achieving Precision: Tolerance, Surface Finish, and Dimensional Accuracy in 3D Printed Manifolds
While metal 3D printing offers incredible design freedom, understanding and managing the achievable levels of precision – encompassing dimensional tolerances, surface finish, and overall accuracy – is crucial for functional components like EV cooling manifolds. These factors directly impact sealing integrity, assembly fit, and potentially fluid dynamics within the manifold. Engineers and procurement specialists need realistic expectations and must specify requirements clearly.
Abmessungstoleranzen:
- As-Built Tolerances: Metal AM processes like Laser Powder Bed Fusion (LPBF) and Selective Electron Beam Melting (SEBM) typically achieve general dimensional tolerances in the range of ±0.1 mm to ±0.3 mm or ±0.2% of the dimension, whichever is larger. This is often comparable to investment casting. For general manifold bodies, tolerances adhering to ISO 2768-m (mittel) oder manchmal ISO 2768-f (fein) might be achievable directly from the printer for non-critical features.
- Faktoren, die die Verträglichkeit beeinflussen: Achievable tolerances depend on several factors:
- Größe und Geometrie des Teils: Larger parts or complex geometries are more prone to thermal distortion during printing, potentially widening tolerances.
- Material: Different materials exhibit varying levels of shrinkage and stress accumulation.
- Kalibrierung und Zustand der Maschine: Well-maintained and accurately calibrated printers yield better results.
- Orientierung aufbauen: The orientation on the build plate affects thermal gradients and support needs, influencing final dimensions.
- Wärmemanagement: The efficiency of heat dissipation during the build process impacts stress and warping.
- Tighter Tolerances via Post-Processing: For critical interfaces, such as sealing faces, connector ports, or precision mounting points, as-built tolerances are often insufficient. Post-process CNC machining is typically employed to achieve tighter tolerances, often reaching ±0.02 mm to ±0.05 mm or better, comparable to standard machining practices. It’s essential to incorporate machining allowances into the DfAM phase for these features.
Oberflächengüte (Rauhigkeit):
- As-Built Surface Roughness: The surface finish of as-printed metal parts is inherently rougher than machined surfaces due to the layer-by-layer fusion of powder particles. Typical as-built surface roughness (Ra) for LPBF parts ranges from 6 µm bis 20 µm (240 µin bis 800 µin), depending on:
- Orientierung: Upward-facing surfaces are generally smoother than downward-facing surfaces (which require supports) or vertical walls (which show layer lines).
- Material & Powder Size: Finer metal powders generally lead to smoother finishes.
- Prozessparameter: Laser power, scan speed, and layer thickness influence melt pool dynamics and resulting roughness.
- Interne Kanäle: The roughness of internal channels is particularly important for fluid flow and pressure drop. As-built internal surfaces tend to be rougher, especially if supports were needed or powder removal was challenging.
- Verbesserung der Oberflächengüte: Various post-processing techniques can significantly improve surface finish:
- Abrasives Strahlen (Perlen-/Sandstrahlen): Common for achieving a uniform matte finish and removing semi-sintered particles. Ra values around 3 µm to 8 µm are typical.
- Taumeln/Gleitschleifen: Effective for smoothing external surfaces and deburring edges, especially for batches of smaller parts.
- Micro-Machining / Polishing: Can achieve very smooth finishes (Ra < 1 µm) on specific surfaces, often required for sealing faces.
- Elektrochemisches Polieren: Can smooth both internal and external surfaces, reducing roughness significantly.
Maßgenauigkeit und Qualitätskontrolle:
- Sources of Inaccuracy: Deviations from the intended geometry can arise from residual stresses causing warping (especially after removal from the build plate), incomplete support leading to sagging, or inaccuracies in the original CAD model or machine calibration.
- Sicherstellung der Genauigkeit: Reputable AM service providers employ rigorous quality control measures:
- Prozess-Simulation: Simulating the build process beforehand can predict potential distortion and allow for compensation in the build file or adjustments to the support strategy.
- Prozessüberwachung: In-situ monitoring of the melt pool and layer deposition can help detect potential anomalies during the build.
- Strict Powder Handling: Ensuring consistent metal powder quality (Met3dp Products are produced using advanced atomization for high sphericity and flowability) is fundamental.
- Controlled Post-Processing: Careful execution of stress relief heat treatment and support removal is critical to prevent distortion.
- Metrologie und Inspektion: Final parts undergo dimensional inspection using CMMs (Coordinate Measuring Machines), 3D scanning, or traditional metrology tools to verify compliance with specified tolerances.
Specification Table Example:
Merkmal | Typical As-Built (LPBF) | Typical Post-Processed (Machining/Polishing) | Anmerkungen |
---|---|---|---|
General Tolerance (Linear) | ±0.1 to ±0.3 mm / ±0.2% | N/A (Defined by feature) | ISO 2768-m often achievable |
Critical Tolerance (Linear) | N/A (Usually insufficient) | ±0.02 to ±0.05 mm (or tighter) | Requires CNC machining; allowance needed in design |
Oberflächenrauhigkeit (Ra) | 6 µm to 20 µm | 0.8 µm to 6 µm (Machined/Blasted) < 0.8 µm (Polished) | Varies significantly with orientation and finishing |
Leak Tightness | Process Dependent | Verified by Testing | Requires dense printing & post-process testing (e.g., pressure decay) |
In Blätter exportieren
Partnering with an experienced provider like Met3dp ensures access to state-of-the-art equipment with industry-leading accuracy and reliability. Their focus on process control, from powder quality management through advanced printing systems to meticulous post-processing and inspection, gives engineers and procurement managers confidence in receiving dimensionally accurate, high-precision EV cooling manifolds that meet demanding automotive specifications.
Essential Post-Processing Steps for Functional EV Cooling Manifolds
A metal 3D printed part, upon removal from the printer, is rarely ready for its final application, especially for demanding uses like automotive fluid handling. A series of crucial post-processing steps are required to relieve internal stresses, remove support structures, achieve the required dimensional tolerances and surface finish, and ensure the part is clean and leak-free. Neglecting or improperly executing these steps can compromise the manifold’s performance, reliability, and longevity.
Common Post-Processing Workflow for AM Manifolds:
- Stressabbau Wärmebehandlung:
- Zweck: This is arguably the most critical step. The rapid heating and cooling inherent in powder bed fusion processes create significant residual stresses within the printed part. These stresses can cause distortion (warping) during or after removal from the build plate, or even lead to cracking over time. Stress relief involves heating the part (while often still attached to the build plate) to a specific temperature below the material’s aging or transformation temperature, holding it for a set duration, and then slowly cooling it.
- Material Specifics:
- AlSi10Mg: Typically stress-relieved at around 250-300°C for 1-2 hours. This stabilizes the part before support removal. Further heat treatments (like T6 – solutionizing and aging) might be applied later to enhance mechanical properties, though this can sometimes affect dimensions slightly.
- CuCrZr: Requires a more complex, multi-stage heat treatment. Typically involves solution annealing (at ~950-1000°C) followed by rapid quenching, and then precipitation hardening (aging at ~450-500°C) to achieve the desired high strength and conductivity. Stress relief might be performed before or integrated within this cycle.
- Wichtigkeit: Performing stress relief correctly is vital for dimensional stability and preventing premature failure.
- Entnahme von der Bauplatte:
- Methoden: Parts are typically removed from the build plate using wire EDM (Electrical Discharge Machining), sawing, or sometimes manual breaking if designed with specific weak points. Care must be taken not to damage the part during this process.
- Entfernung der Stützstruktur:
- Zweck: Support structures are necessary during printing to anchor the part and support overhanging features, preventing collapse and distortion. These must be removed afterward.
- Methoden: Dies kann sehr arbeitsintensiv sein. Zu den Methoden gehören:
- Manuelle Entfernung: Breaking or cutting away supports using hand tools (pliers, cutters, grinders). Requires skill to avoid damaging the part surface.
- CNC-Bearbeitung: Milling or grinding away support structures, often used for more robust supports or critical surface interfaces.
- Elektrochemische Bearbeitung (ECM): Can sometimes be used for support removal, particularly for internal supports if access allows.
- Herausforderungen: Removing supports from complex internal channels can be extremely difficult and requires careful DfAM planning (minimizing internal supports, designing access points).
- Cleaning and Powder Removal:
- Zweck: Thoroughly removing all unfused metal powder, especially from internal channels, is critical. Trapped powder can impede coolant flow, potentially dislodge and damage downstream components (like pump impellers), and prevent effective leak testing or surface treatments.
- Methoden: Compressed air, specialized vacuum systems, vibration, and sometimes ultrasonic cleaning baths are used. Design features like strategically placed drain/access holes are essential. Inspection with borescopes may be necessary for complex internal passages.
- Machining for Critical Tolerances & Features:
- Zweck: As discussed previously, achieving tight tolerances on sealing surfaces, connector interfaces, sensor ports, and mounting points typically requires secondary machining operations.
- Methoden: CNC milling or turning is used to machine specific features to final dimensions and required surface finishes.
- Oberflächenveredelung:
- Zweck: To achieve the desired surface roughness for functional reasons (e.g., improved flow in channels, better sealing) or aesthetics, and to remove any remaining support nibs or minor imperfections.
- Methoden: Abrasive blasting (bead, sand, shot), tumbling/vibratory finishing, polishing, or electrochemical polishing, depending on the requirements.
- Dichtheitsprüfung:
- Zweck: Absolutely essential for any fluid-carrying component. This verifies the integrity of the manifold walls and all joints/interfaces.
- Methoden:
- Pressure Decay Test: Pressurizing the manifold with air or inert gas to a specified pressure, sealing it, and monitoring for any pressure drop over time indicates a leak.
- Helium Leak Detection: A more sensitive method using helium as a tracer gas and a mass spectrometer to detect even minute leaks. Often required for high-integrity applications.
- Submersion Test: Pressurizing the part and submerging it in water or another liquid to visually check for bubbles.
- Final Cleaning & Inspection:
- Zweck: Ensure the part is free from machining fluids, debris, or contaminants before packaging and shipment. Final dimensional and visual inspection confirms all requirements are met.
The complexity and criticality of these post-processing steps highlight the importance of working with a full-service additive manufacturing provider. Companies like Met3dp offer comprehensive solutions that span not only advanced printing but also the necessary downstream processes. Their integrated approach ensures that each step, from heat treatment tailored to specific alloys like CuCrZr or AlSi10Mg to meticulous cleaning and final inspection, is performed correctly, delivering functional, reliable EV cooling manifolds ready for assembly. Procurement managers sourcing B2B additive manufacturing partners should verify the supplier’s in-house or validated third-party capabilities for all required post-processing stages.
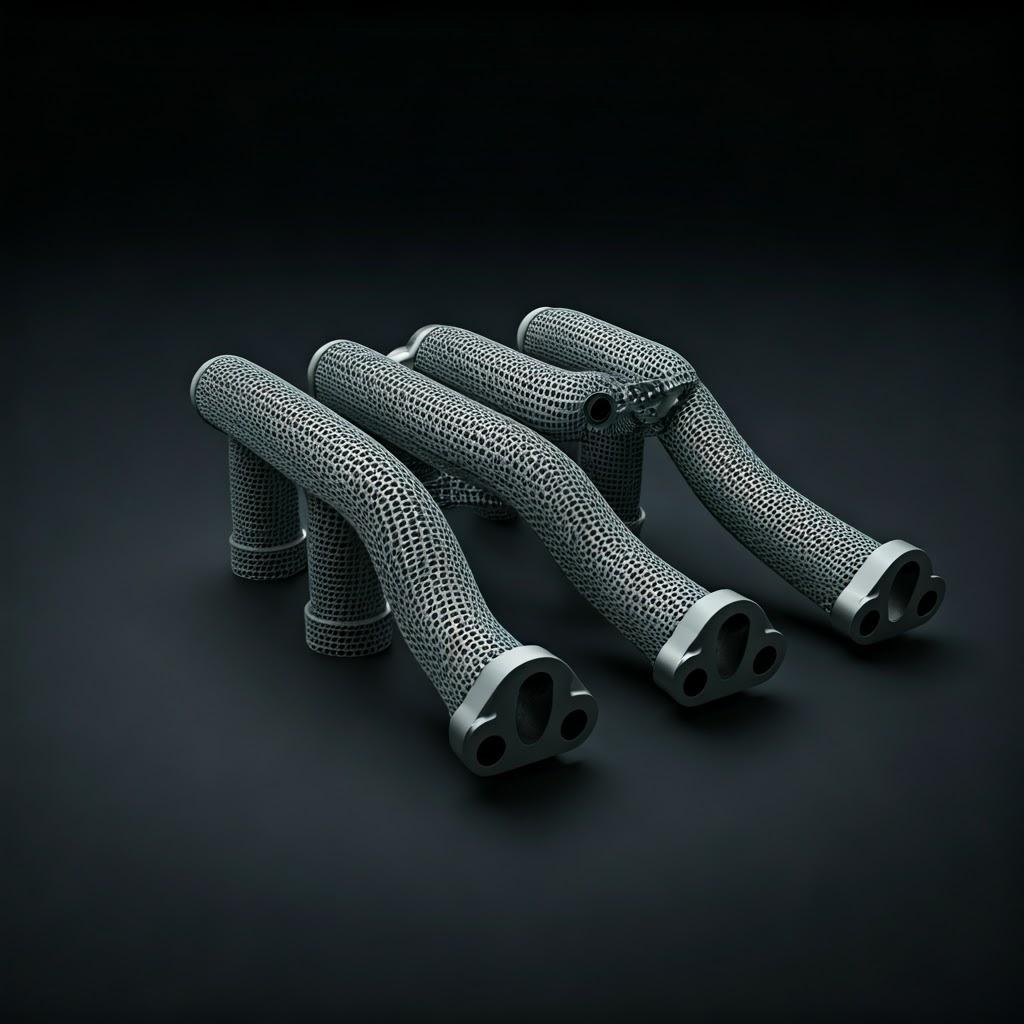
Overcoming Common Challenges in Metal 3D Printing for EV Cooling Components
While metal additive manufacturing offers significant advantages for producing EV cooling manifolds, it’s not without its challenges. Understanding these potential hurdles and the strategies to mitigate them is crucial for successful implementation, ensuring consistent quality and reliable performance. Automotive engineers and procurement managers should be aware of these factors when evaluating AM as a production solution.
Gemeinsame Herausforderungen und Abhilfestrategien:
- Residual Stress, Distortion, and Cracking:
- Herausforderung: The rapid heating/cooling cycles inherent in LPBF/SEBM generate internal stresses. These stresses can cause the part to warp or distort, especially after removal from the build plate, or even lead to cracks, particularly in complex geometries or crack-sensitive materials like some copper or high-strength aluminum alloys.
- Milderung:
- Build Simulation: Using simulation software to predict stress accumulation and distortion, allowing for pre-compensation in the CAD model or optimization of build orientation and support structures.
- Optimierte Scan-Strategien: Using specific laser/electron beam scanning patterns (e.g., island scanning, checkerboard patterns) to distribute heat more evenly and reduce localized stress peaks.
- Robuste Stützstrukturen: Well-designed supports help anchor the part firmly during printing, resisting deformation forces.
- Wärmemanagement: Utilizing heated build platforms (common in SEBM, less so but available in some LPBF) can reduce thermal gradients.
- Prompt Stress Relief: Performing stress relief heat treatment immediately after printing, often before removing the part from the build plate, is critical for stabilizing the component.
- Material Selection & Parameter Tuning: Choosing alloys with lower crack sensitivity where possible, and meticulously tuning process parameters (power, speed, layer thickness) for the specific material and geometry. Met3dp’s deep materials science expertise and control over hochwertige Metallpulver are key assets here.
- Pulverentfernung aus internen Kanälen:
- Herausforderung: Complex manifold designs often feature intricate, winding internal channels. Ensuring all unfused metal powder is removed from these passages after printing is vital but can be difficult. Trapped powder obstructs flow and risks contaminating the cooling system.
- Milderung:
- DfAM für die Pulverentfernung: Designing channels with sufficient diameter, smooth bends, and strategically placed access/drain holes specifically for powder evacuation. Avoiding dead-end cavities.
- Optimierte Ausrichtung: Orienting the part during build setup to facilitate powder drainage via gravity.
- Gründliche Reinigungsverfahren: Employing multi-step cleaning processes involving vibration, compressed air, potentially ultrasonic cleaning, and sometimes flushing with appropriate fluids.
- Inspektion: Using borescopes or other methods to visually inspect internal channels for remaining powder.
- Achieving Leak-Proof Integrity:
- Herausforderung: Manifolds must be completely leak-proof under operating pressures. Achieving full density (no porosity) and preventing micro-cracks, especially in thin walls or complex joints created during part consolidation, is essential.
- Milderung:
- Optimierte Prozessparameter: Using carefully developed and validated printing parameters to achieve >99.5% density in the printed material. Met3dp’s focus on industry-leading accuracy and reliability addresses this directly.
- Qualitätskontrolle des Pulvers: Using high-quality, spherical powder with controlled particle size distribution and low oxygen content minimizes porosity defects.
- Geeignete Wanddicke: Designing walls thick enough to ensure structural integrity and provide a margin against potential localized porosity.
- Strenge Dichtheitsprüfungen: Implementing mandatory pressure decay or helium leak testing on 100% of manufactured manifolds as a final quality check.
- Support Structure Strategy and Removal:
- Herausforderung: Designing effective support structures that prevent deformation but are also removable without damaging the part, especially from internal areas or delicate features, requires expertise. Support removal can be time-consuming and costly.
- Milderung:
- DfAM für die Minimierung der Unterstützung: Designing parts with self-supporting angles (>45°) wherever possible.
- Smart Support Generation: Using advanced software to generate supports that are strong where needed but have weaker connection points for easier removal (e.g., conical supports, perforated supports).
- Careful Manual Removal / Machining: Employing skilled technicians or precise machining operations for support removal.
- Considering Support-Free Processes (Where Applicable): Exploring processes that inherently require fewer supports, though these may have other trade-offs.
- Konsistenz und Reproduzierbarkeit:
- Herausforderung: Ensuring that every manifold produced meets the same dimensional accuracy, material properties, and performance standards, especially for serial production.
- Milderung:
- Robustes Qualitätsmanagementsystem (QMS): Implementing a comprehensive QMS (e.g., aligned with ISO 9001 or IATF 16949 principles for automotive suppliers) covering powder management, machine calibration, process monitoring, post-processing control, and final inspection.
- Process Monitoring & Control: Utilizing machines with in-situ monitoring capabilities and maintaining tight control over all process parameters.
- Standardisierte Verfahren: Having well-documented procedures for every step from design review to final shipment.
- Regular Maintenance & Calibration: Ensuring all printing and testing equipment is regularly maintained and calibrated.
Successfully navigating these challenges requires a combination of design expertise (DfAM), material science knowledge, precise process control, and rigorous quality assurance. Partnering with an established metal AM provider like Met3dp, which combines jahrzehntelanges kollektives Fachwissen with advanced equipment and high-quality material production, significantly de-risks the adoption of 3D printing for critical EV components. Their focus on delivering branchenführendes Druckvolumen, Genauigkeit und Zuverlässigkeit provides the necessary foundation for tackling these challenges head-on.
Selecting the Right Metal AM Partner: Criteria for Choosing Your EV Manifold Supplier
The success of implementing 3D printed EV cooling manifolds depends heavily on the capabilities and expertise of your chosen additive manufacturing partner. This is not merely a transactional purchase; it’s a technical partnership requiring collaboration, trust, and a deep understanding of both the AM process and the demanding requirements of the automotive industry. Selecting the right supplier is critical for mitigating risks, ensuring quality, and realizing the full benefits of the technology. Procurement managers and engineering teams should evaluate potential B2B additive manufacturing partners based on a comprehensive set of criteria:
Key Evaluation Criteria for Metal AM Suppliers:
- Automotive & Application Experience:
- Does the supplier have demonstrable experience working with automotive clients (OEMs or Tier 1 suppliers)?
- Do they understand the specific challenges of thermal management components and fluid handling systems?
- Can they provide case studies or examples of similar projects (e.g., heat exchangers, fluid manifolds, complex housings)?
- Material Expertise & Powder Quality:
- Do they have proven expertise in processing the required materials (e.g., AlSi10Mg, CuCrZr)? Have they developed robust, validated parameters?
- What is their process for qualifying and controlling metal powder quality? Do they source from reputable suppliers or, ideally, manufacture their own high-quality powders? (Met3dp’s in-house powder production using advanced gas atomization and PREP ensures high sphericity, flowability, and purity – crucial for consistent part quality).
- Can they provide material datasheets based on printed and appropriately post-processed specimens?
- Technology & Equipment:
- What types of metal AM technologies do they offer (LPBF, SEBM, etc.)? Do these align with the project requirements?
- What is the build volume, accuracy, and feature resolution capability of their machines?
- Do they invest in modern, well-maintained equipment from reputable manufacturers?
- What are their in-house capabilities for essential post-processing (heat treatment, machining, finishing, cleaning, leak testing)?
- Quality Management System (QMS) & Certifications:
- Is the supplier ISO 9001 certified? While full IATF 16949 certification may be rare for pure AM service bureaus, do their processes align with its principles (traceability, process control, corrective actions)?
- What are their procedures for process monitoring, part inspection (metrology equipment like CMMs, scanners), and quality documentation?
- How do they ensure traceability from raw powder batch to final shipped part?
- Engineering & DfAM Support:
- Do they offer design consultation and DfAM support to help optimize parts for additive manufacturing?
- Can their engineers collaborate effectively with your design team?
- Do they utilize simulation tools for predicting build outcomes (stress, distortion)?
- Capacity & Scalability:
- Can they accommodate your required volumes, from initial prototypes to potential low- or mid-volume series production?
- Do they have sufficient machine capacity and skilled personnel to meet agreed-upon lead times?
- Project Management & Communication:
- Do they provide clear communication channels and responsive project management?
- Are their quoting processes transparent and detailed?
- Standort und Logistik:
- Consider the supplier’s location relative to your facilities for shipping times and costs, though global specialists are common.
Met3dp exemplifies many of these essential qualities. As a company specializing in both 3D-Druck-Ausrüstung und Hochleistungsmetallpulver, Met3dp possesses end-to-end expertise. Their focus on industrial applications in sectors like aerospace, medical, and automotive means they understand the need for branchenführendes Druckvolumen, Genauigkeit und Zuverlässigkeit. Mit jahrzehntelanges kollektives Fachwissen, advanced powder manufacturing, and a commitment to comprehensive solutions, they position themselves as a strong potential partner. Exploring their capabilities further on their Über uns page can provide deeper insight into their company philosophy and experience. Choosing a partner like Met3dp, who truly understands the intricacies of metal AM from powder to finished part, significantly increases the likelihood of success for your EV cooling manifold project.
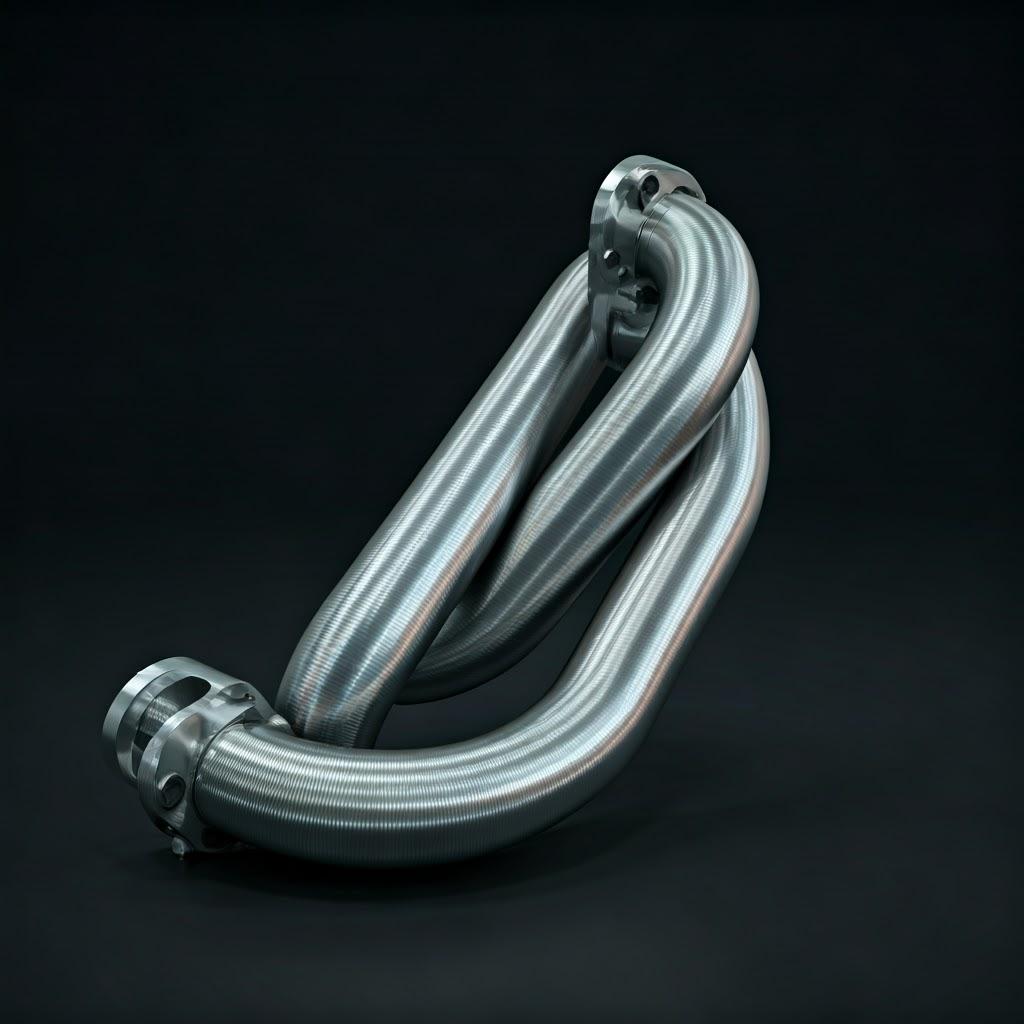
Understanding Cost Drivers and Lead Times for 3D Printed Manifolds
While performance benefits are compelling, cost and lead time remain critical considerations for adopting any manufacturing technology in the automotive sector. Understanding the factors that influence the price and production timeline of 3D printed EV cooling manifolds is essential for accurate budgeting, project planning, and comparing AM against traditional methods.
Die wichtigsten Kostentreiber:
- Materialart und -verbrauch:
- Pulverkosten: Metal powders vary significantly in price. High-performance alloys like CuCrZr are generally more expensive than standard AlSi10Mg. The cost is directly related to the weight of the powder consumed, which includes the part itself and any support structures.
- Recycling-Effizienz: The ability to safely recycle and reuse unfused powder affects overall material cost efficiency.
- Part Volume & Build Height:
- Maschinenzeit: The primary driver is often the time required to print the part(s). This is influenced by the total volume of material being fused and, critically, the height of the part in the build chamber (as printing is layer by layer). Taller parts take longer.
- Build Plate Utilization: Printing multiple parts simultaneously on a single build plate (nesting) significantly reduces the cost per part by distributing the machine setup and operation time. Maximizing build density is key for bulk order 3D printing economics.
- Part Complexity & Design:
- Unterstützende Strukturen: Complex geometries with numerous overhangs require extensive support structures. These consume extra material and add significant time and labor for removal during post-processing. DfAM efforts to minimize supports directly impact cost.
- Wanddicke: Very thin walls might require slower printing speeds or specialized parameters, potentially increasing time.
- Nachbearbeitungsanforderungen:
- Wärmebehandlung: Required cycles (stress relief, aging) add time and cost (furnace time, energy). Complex cycles for alloys like CuCrZr cost more than simple stress relief for AlSi10Mg.
- Support Removal & Machining: Labor-intensive steps like manual support removal or precision CNC machining of multiple features add significant cost.
- Oberflächenveredelung: Techniques like polishing or electrochemical polishing add cost compared to standard bead blasting.
- Testing & Inspection: Rigorous testing (leak testing, dimensional inspection with CMM) adds to the final cost but is essential for quality assurance.
- Bestellmenge:
- Amortization: Setup costs (build preparation, machine setup) are amortized over the number of parts in a batch. Larger quantities generally lead to a lower price per part.
- Mengenrabatte: Suppliers often offer tiered pricing for larger wholesale orders.
Typische Vorlaufzeiten:
Lead times for 3D printed manifolds can vary considerably based on complexity, quantity, material, post-processing needs, and the supplier’s current capacity.
- Prototypen: For single parts or very small batches, lead times are typically measured in days to a few weeks. This includes printing, basic post-processing (stress relief, support removal, basic finish), and shipping. The rapid turnaround for prototypes is a major advantage of AM.
- Low-to-Mid Volume Production: For batch production (tens to hundreds of parts), lead times extend to several weeks or even a couple of months. This longer duration accounts for optimized build nesting, scheduling machine time, managing the workflow through various post-processing stages (which can create bottlenecks), and comprehensive quality control procedures.
- Faktoren, die die Vorlaufzeit beeinflussen:
- Verfügbarkeit der Maschine: Current workload and scheduling at the supplier.
- Post-Processing Complexity: Extensive machining or specialized finishing adds significant time.
- Materialverfügbarkeit: Ensuring sufficient qualified powder is on hand.
- Validation Requirements: If specific testing or customer approvals are needed at various stages.
It’s crucial to discuss specific cost and lead time expectations with potential suppliers early in the engagement, providing detailed CAD models and specifications to enable accurate quoting. While AM might have a higher piece price than high-volume casting for simple parts, its ability to create complex, consolidated, high-performance manifolds with minimal tooling investment can offer a compelling total cost of ownership, especially when factoring in accelerated development and improved vehicle performance.
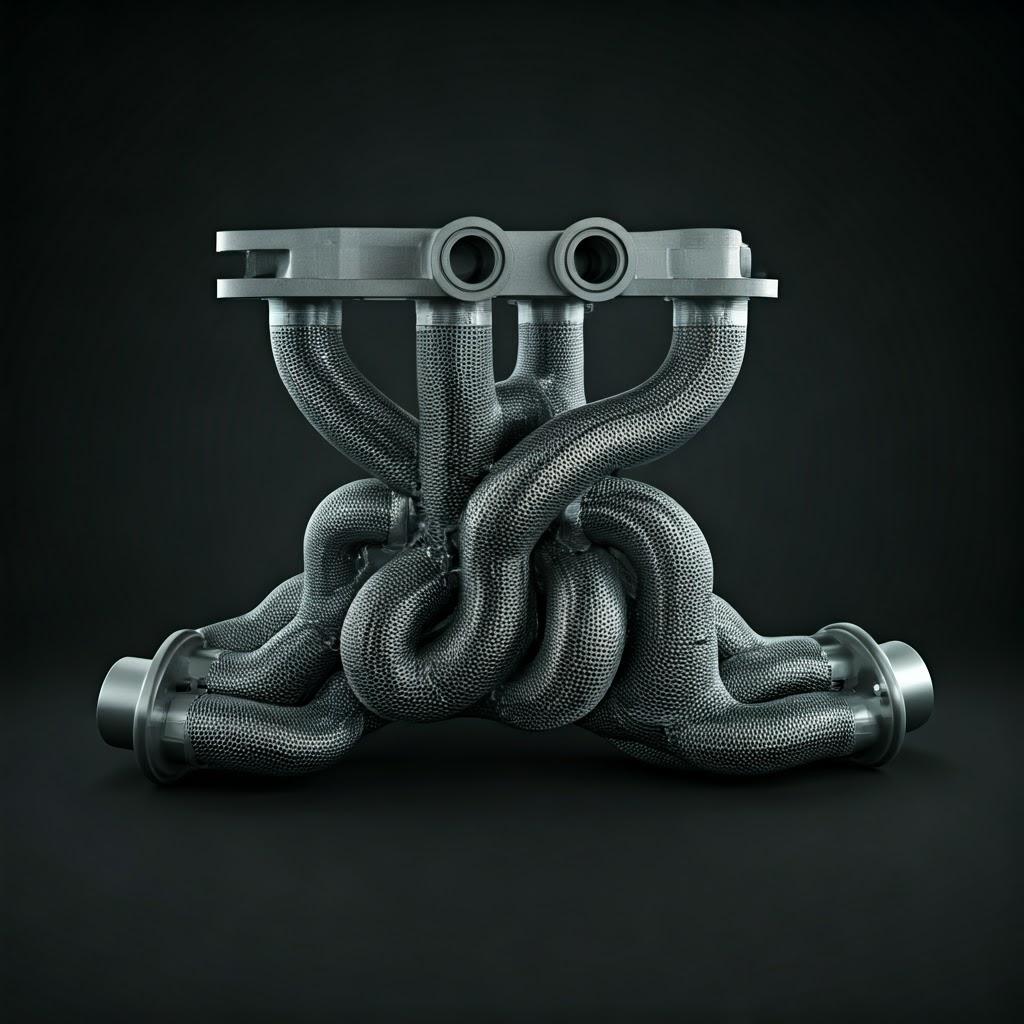
Frequently Asked Questions (FAQ) about 3D Printed EV Cooling Manifolds
Here are answers to some common questions engineers and procurement managers have about using metal additive manufacturing for EV cooling manifolds:
1. Are 3D printed metal manifolds as reliable and durable as traditionally manufactured ones (e.g., cast aluminum)?
Yes, when designed, printed, and post-processed correctly using appropriate materials and stringent quality control. Metal AM can produce fully dense parts (>99.5%) with mechanical properties comparable or even superior (in some aspects like yield strength) to equivalent cast materials after heat treatment. Key factors ensuring reliability include:
- Proper DfAM to avoid stress concentrations.
- Using high-quality, contamination-free metal powders.
- Optimized and validated printing parameters to ensure density and fusion.
- Appropriate stress relief and other heat treatments.
- Thorough cleaning and removal of internal powder.
- Rigorous leak testing and dimensional verification. Partnering with an experienced supplier like Met3dp, focused on industrial quality, is crucial for achieving reliability equivalent to or exceeding traditional methods, especially for complex geometries where AM excels.
2. What are the typical operating pressure and temperature limits for 3D printed manifolds (e.g., in AlSi10Mg or CuCrZr)?
The limits depend heavily on the specific design (wall thickness, geometry), the chosen material, and the post-processing (heat treatment state).
- Pressure: Properly designed and manufactured manifolds can typically handle the pressures found in automotive coolant systems, which are often in the range of 1.5 to 3 bar (approx. 20-45 psi), with safety factors applied. Burst pressure testing during validation confirms the design limits, which can often far exceed standard operating pressures.
- Temperatur:
- AlSi10Mg: Suitable for typical battery cooling loops (often operating below 60-80°C). Its properties degrade at higher temperatures, making it less ideal for direct, high-temperature powertrain components without careful design consideration.
- CuCrZr: Maintains good strength and excellent thermal conductivity at higher temperatures (up to ~300°C or more, depending on the specific condition and exposure time), making it suitable for more demanding powertrain or power electronics cooling applications. Always consult material datasheets for the specific AM material and condition, and validate the design through simulation and physical testing under worst-case operating conditions.
3. How does the cost of 3D printed manifolds compare to casting or fabrication, especially at higher volumes?
- Prototypes & Low Volumes: AM is almost always more cost-effective and significantly faster because it eliminates tooling costs (molds, dies, jigs) which can run into tens or hundreds of thousands of dollars.
- Mid Volumes (Hundreds to Low Thousands): AM can remain competitive, especially if the design complexity is high, part consolidation offers significant assembly savings, or lightweighting/performance benefits provide substantial value. The break-even point depends heavily on the part’s specifics.
- High Volumes (Tens of Thousands+): For simpler designs that are easily castable or fabricated, traditional methods usually become more cost-effective per piece due to economies of scale. However, if the performance gains or packaging advantages enabled nur by AM are critical, it might still be considered. It’s not just about piece price; consider the total cost of ownership, including development time, assembly labor, weight savings impact on vehicle range, and potential performance improvements.
4. Can internal channels be made smooth enough for efficient fluid flow?
As-printed internal surfaces are rougher than machined ones (typically Ra 10-20 µm). However, several factors mitigate this:
- DfAM: Designing for smooth bends and avoiding sharp transitions minimizes flow disruption despite roughness.
- Maßstab: For typical manifold channel diameters, the relative roughness may have a manageable impact on pressure drop.
- Nachbearbeiten: Techniques like abrasive flow machining or electrochemical polishing can significantly smooth internal channels if extremely low pressure drop or specific flow characteristics are required, albeit at an additional cost. CFD analysis during the design phase helps predict the impact of expected roughness and optimize the design accordingly.
Conclusion: Driving the Future of EV Performance with Advanced Metal AM Solutions
The transition to electric mobility demands continuous innovation in every aspect of vehicle design and manufacturing. Effective thermal management is non-negotiable for ensuring the performance, longevity, and safety of electric vehicles. As we’ve explored, metal additive manufacturing offers a powerful solution for producing EV cooling tube manifolds that push beyond the limitations of traditional methods.
By leveraging the unparalleled design freedom of AM, engineers can create highly optimized, complex, and consolidated manifolds using advanced materials like high-conductivity CuCrZr or lightweight AlSi10Mg. The results are components that offer superior thermal performance, fit into tighter spaces, reduce overall vehicle weight, and simplify assembly – all critical factors in the competitive EV market. Key takeaways include:
- Performance Boost: AM enables intricate internal channels and optimized geometries for enhanced cooling efficiency.
- Gestaltungsfreiheit: Facilitates part consolidation, lightweighting through topology optimization, and adaptation to complex packaging constraints.
- Material Advantage: Allows effective use of high-performance materials tailored to specific thermal and mechanical needs.
- Accelerated Innovation: Radically reduces lead times for prototypes and enables faster design iteration cycles.
However, realizing these benefits requires careful consideration of Design for Additive Manufacturing (DfAM) principles, meticulous execution of essential post-processing steps (like heat treatment and leak testing), and robust quality control. Overcoming challenges related to residual stress, powder removal, and achieving precision demands expertise and advanced capabilities.
This underscores the importance of selecting the right manufacturing partner. Companies like Met3dp, operating at the forefront of metal powder production and additive manufacturing systems, provide the integrated expertise necessary to navigate the complexities of AM. Their commitment to producing high-quality powders and delivering industry-leading printing solutions empowers automotive manufacturers and suppliers to confidently adopt this transformative technology.
Metal 3D printing is no longer just a prototyping tool; it is a viable and increasingly advantageous solution for producing critical, high-performance components for the electric vehicles shaping our future. By embracing metal AM for components like cooling tube manifolds, the automotive industry can accelerate innovation, enhance vehicle performance, and drive the transition towards sustainable mobility.
Ready to explore how metal additive manufacturing can revolutionize your EV thermal management systems? Kontaktieren Sie Met3dp noch heute to discuss your application requirements and discover how our advanced powders and printing solutions can power your organization’s additive manufacturing goals.
Teilen auf
Facebook
Twitter
LinkedIn
WhatsApp
E-Mail
MET3DP Technology Co., LTD ist ein führender Anbieter von additiven Fertigungslösungen mit Hauptsitz in Qingdao, China. Unser Unternehmen ist spezialisiert auf 3D-Druckgeräte und Hochleistungsmetallpulver für industrielle Anwendungen.
Fragen Sie an, um den besten Preis und eine maßgeschneiderte Lösung für Ihr Unternehmen zu erhalten!
Verwandte Artikel
Über Met3DP
Aktuelles Update
Unser Produkt
KONTAKT US
Haben Sie Fragen? Senden Sie uns jetzt eine Nachricht! Wir werden Ihre Anfrage mit einem ganzen Team nach Erhalt Ihrer Nachricht bearbeiten.
Holen Sie sich Metal3DP's
Produkt-Broschüre
Erhalten Sie die neuesten Produkte und Preislisten
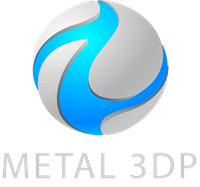
Metallpulver für 3D-Druck und additive Fertigung
UNTERNEHMEN
PRODUKT
cONTACT INFO
- Qingdao Stadt, Shandong, China
- [email protected]
- [email protected]
- +86 19116340731