Maskiner för smältning med elektronstråle
Innehållsförteckning
Översikt över electron beam melting machine
Electron beam melting (EBM) is an additive manufacturing technology used to fuse metal powders into fully dense parts layer-by-layer using a high power electron beam under vacuum conditions. EBM machines offer unmatched build speeds and mechanical properties unachievable with other metal 3D printing methods.
Key attributes of EBM technology include:
Table 1: Overview of Electron Beam Melting Technology
Attribut | Beskrivning |
---|---|
Värmekälla | High intensity electron beam |
Miljö | High vacuum |
Råmaterial | Metal powder bed |
Beam Control | Electromagnetic lenses and coils |
Build Mode | Layer-by-layer metal powder fusion |
Tillämpningar | Aerospace, medical, automotive, tooling |
By leveraging precise beam focusing and rapid scanning, EBM fuses conductive materials like titanium, nickel alloys, tool steels, and refractory metals into fully dense components with superior properties exceeded only by wrought products.
The controlled vacuum environment prevents contamination while the intelligent energy delivery and high preheat temperatures minimize residual stresses leading to warp or cracks.
Understanding these core principles helps illustrate why EBM delivers exceptional mechanical performance tailor-made for the most demanding industry applications.
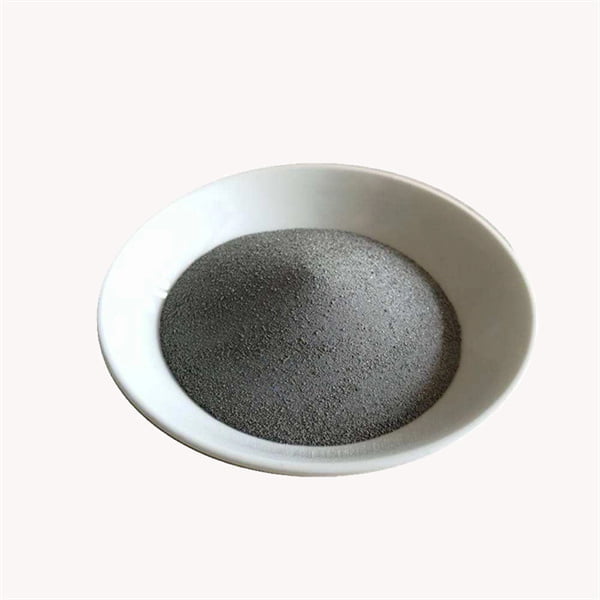
Types of Electron Beam Melting Systems
Funktion | Beskrivning | Impact on System Use |
---|---|---|
Electron Gun Configuration | Electron beam melting systems utilize an electron gun to generate a high-energy beam that selectively melts metal powder. There are two main configurations for electron guns: * Sidewinder: The electron beam source is positioned horizontally alongside the build chamber. This design allows for a larger build chamber volume but may limit access for post-processing. * Top Gun: The electron beam source is situated directly above the build chamber. This configuration offers easier access for part removal and monitoring but may restrict the maximum build envelope. | The selection of gun configuration depends on the priority between build volume and accessibility. Sidewinders are ideal for high-throughput production of larger parts, while Top Guns are better suited for research and development applications where frequent part monitoring and manipulation are necessary. |
Beam Melting Mode | EBM systems can operate in two primary beam melting modes: * Raster Scan: The electron beam rapidly scans across the powder bed in a predetermined pattern, melting designated areas layer-by-layer. This method is efficient for simple geometries but can create stair-stepping artifacts on curved surfaces. * Vector Scan: The electron beam follows a defined vector path to melt the powder. This method offers greater control over melt pool geometry and surface finish, particularly for intricate features. However, vector scanning can be slower than raster scanning for simple geometries. | The choice between raster and vector scan modes depends on the desired balance between speed, resolution, and surface quality. Raster scanning is preferred for high-volume production of parts with basic geometries, while vector scanning is more suitable for complex parts demanding high accuracy and surface finish. |
Vacuum System Design | Electron beam melting necessitates a high vacuum environment (typically below 10^-4mbar) to prevent oxidation of the molten metal and ensure beam stability. There are two common vacuum system designs: * Diffusion Pump: This traditional design utilizes diffusion pumps to achieve the required vacuum level. Diffusion pumps are reliable and well-established but require routine maintenance and can be relatively slow to reach optimal vacuum levels. * Cryopumping: This newer technology employs cryopumps to capture gas molecules through extreme cooling. Cryopumps offer faster pump-down times and reduced maintenance needs compared to diffusion pumps. However, they have a higher initial investment cost and may require additional plumbing for liquid nitrogen supply. | The selection of vacuum system design hinges on factors like budget, maintenance requirements, and desired cycle times. Diffusion pumps are a cost-effective choice for established production environments, while cryopumping offers advantages for high-throughput applications or research settings prioritizing fast turnaround times. |
System för pulvertillförsel | The even distribution of metal powder across the build platform is critical for successful EBM builds. Two primary powder delivery systems are employed: * Blade Coating: A blade or rake spreads a thin layer of powder over the build platform after each melting cycle. Blade coating is a simple and reliable method but can cause inconsistencies in powder density due to variations in blade pressure and angle. * Recoil Spreading: A high-pressure gas stream disrupts the powder bed, causing the powder particles to recoil and deposit a fresh layer. Recoil spreading offers more uniform powder distribution compared to blade coating but requires careful optimization of gas pressure to avoid disturbing previously melted layers. | The powder delivery system selection impacts layer quality and repeatability. Blade coating is suitable for low-volume production or applications where powder density is less critical. Recoil spreading is preferred for high-precision parts demanding consistent powder distribution. |
Fundamentals of Electron Beam Melting Process
Etapp | Beskrivning | Fördelar | Överväganden |
---|---|---|---|
Pulverberedning | Metal powders used in EBM are meticulously produced to ensure optimal flowability, particle size distribution, and chemical purity. Particle size plays a crucial role, as excessively fine powders can hinder layer spreading, while overly coarse powders can lead to balling and poor fusion. Strict control over oxygen, nitrogen, and other elements is essential to minimize porosity and achieve the desired mechanical properties. | * Consistent material properties throughout the build * Reduced risk of defects like porosity and cracking | * High upfront cost of high-quality metal powders * Need for specialized handling and storage due to potential reactivity |
Vakuumkammare | Electron Beam Melting necessitates a high vacuum environment (typically between 10⁻⁴ and 10⁻⁹ Pa) to prevent oxidation and contamination of the molten metal. This is achieved by powerful vacuum pumps that extract air molecules from the chamber. The absence of oxygen allows for processing of reactive metals like titanium and tantalum. | * Minimizes interaction between molten metal and atmospheric gases * Enables production of high-density parts with excellent mechanical properties | * Complex and expensive vacuum pumping systems required * Additional considerations for moisture control to prevent spatter and inconsistencies |
Electron Beam Generation | At the heart of EBM lies an electron gun, which emits a focused beam of high-energy electrons. An electric field heats a filament (cathode), causing it to release electrons. These electrons are accelerated by a high voltage towards an anode and further concentrated by electromagnetic coils. The resulting beam boasts exceptional power density, capable of melting even refractory metals with high melting points. | * Precise control over melt pool size and depth * Deep penetration for complex geometries with internal features | * Requires high voltage and sophisticated beam control systems * Maintenance and calibration of the electron gun are crucial |
Powder Delivery and Spreading | A blade or roller mechanism meticulously spreads a thin layer of metal powder across the build platform. This layer thickness, typically ranging from 30 to 100 microns, is predetermined by the desired resolution and part geometry. | * Ensures uniform layer thickness for consistent material properties * Enables creation of intricate features | * Precise control of powder spreading mechanism is critical * Powder flowability and particle size distribution can impact spreading efficiency |
Selective Melting | The electron beam, guided by computer-controlled scanning mirrors, selectively melts specific regions of the powder bed according to a sliced 3D model. The unmelted powder acts as support for overhanging structures, eliminating the need for external support structures common in other additive manufacturing techniques. | * High precision and accuracy in building complex geometries * Capability to create internal features and channels | * Careful scan path planning is necessary to optimize melting and minimize residual stress |
Layer-by-Layer Building | Following the melting of a single layer, a fresh layer of powder is deposited on top. The electron beam then selectively melts designated areas within this new layer, fusing it to the previously melted layer. This process continues iteratively until the entire 3D object is complete. | * Creates complex geometries in a step-wise manner * Enables efficient use of material, minimizing waste | * Build time can be longer compared to some other AM processes due to the layer-by-layer approach |
Heat Management and Build Platform | Thermal management plays a vital role in EBM. The build platform is typically preheated to a temperature slightly below the melting point of the powder to improve flowability and promote bonding. A water-cooled copper crucible further aids in heat dissipation and prevents warping of the final part. | * Minimizes thermal stresses and residual stress build-up * Contributes to dimensional accuracy and part quality | * Careful control of preheating temperature and cooling rates is necessary |
Part Removal and Post-Processing | Once the build process is complete, the chamber is brought back to atmospheric pressure. The built part remains adhered to the build platform and needs to be separated. Support structures, if any, are carefully removed using mechanical techniques. Depending on the application, post-processing steps like heat treatment, hot isostatic pressing, or machining may be employed to achieve the desired surface finish and mechanical properties. | * Minimizes post-processing steps due to high density and near-net-shape capabilities * Enables creation of functional parts | * Removal process requires care to avoid damaging delicate features * Heat treatment or other post-processing steps may add to overall processing time |
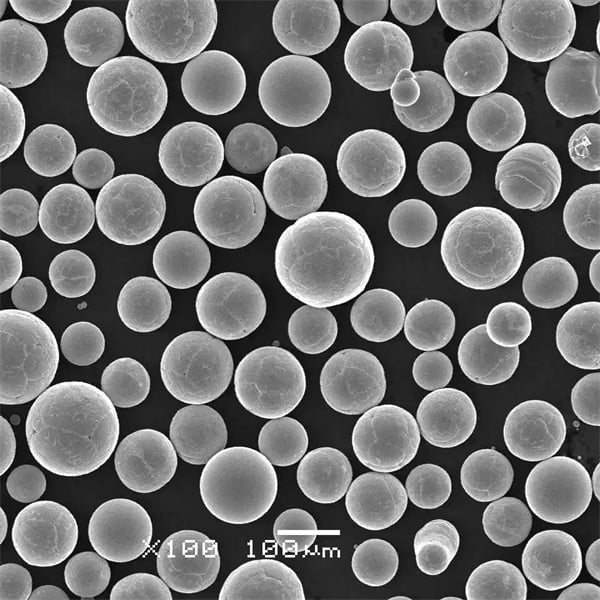
Key Specifications of electron beam melting machine
Specifikation | Beskrivning | Impact on Part Quality and Production |
---|---|---|
Beam Power (kW) | This refers to the total power output of the electron beam. | Higher beam power translates to faster melting speeds and increased throughput. It also allows for processing of thicker layers and denser materials. However, excessive power can lead to overheating and warping of the part. |
Bygg kuvertet (mm) | This defines the maximum dimensions (length, width, height) of a part that can be manufactured in a single build. | A larger build envelope offers greater design flexibility for complex geometries. However, it also increases the machine footprint, powder consumption, and overall build time. |
Vacuum Level (Pa) | The pressure within the EBM chamber, crucial for preventing oxidation and contamination during the melting process. | A high vacuum (lower Pa value) ensures minimal interaction between the molten metal and atmospheric gases, resulting in parts with superior mechanical properties and high density. However, achieving and maintaining a deeper vacuum requires more powerful pumps, adding to the machine cost and complexity. |
Skiktets tjocklek (μm) | The thickness of each individual layer of metal powder spread and melted during the build process. | Thinner layers offer finer detail and smoother surface finishes. However, they require more build time due to the increased number of layers needed. Conversely, thicker layers can be faster to build but may result in a rougher surface and potential internal porosity. |
Materialkompatibilitet | The range of metal powders that the EBM system can process. | A wider material compatibility allows for the creation of parts from diverse alloys with specific properties suited for various applications. However, some materials have higher melting points or require specialized handling, which might limit machine options. |
In-Situ Monitoring | The ability to monitor the melting process in real-time using cameras or other sensors. | In-situ monitoring allows for early detection of defects like spatter or layer cracking, enabling corrective actions to be taken during the build. This can significantly improve yield and reduce the risk of part failure. |
Melt Pool Geometry Control | The level of precision with which the electron beam can be focused and manipulated to control the shape of the molten metal pool. | Precise melt pool control minimizes heat-affected zones and residual stresses, leading to parts with enhanced mechanical performance and fatigue resistance. This is particularly important for applications with high-stress requirements. |
Powder Recoating System | The mechanism used to deliver and spread fresh metal powder across the build platform after each layer is melted. | An efficient recoating system ensures consistent powder layer thickness and minimizes the risk of contamination from residual powder particles. Blade and roller-based systems are common, each with its own advantages and limitations. |
Support Generation Software | The software used to design and generate support structures within the 3D model. | Well-designed support structures are crucial for preventing part distortion and ensuring proper heat dissipation during the build. Advanced software can optimize support placement and minimize material waste. |
Krav på efterbearbetning | The level of post-processing needed to achieve the desired final part properties. | EBM parts typically require minimal post-processing compared to other additive manufacturing techniques. However, support structure removal and surface finishing may still be necessary depending on the application. |
Economics of Electron Beam Melting Adoption
Faktor | Impact on Adoption | Förklaring |
---|---|---|
Capital Expenditure | Hög | EBM machines carry a hefty upfront cost, ranging from $500,000 to several million dollars depending on build volume and capabilities. This can be a significant barrier for smaller manufacturers or those new to additive manufacturing. |
Operational Costs | Mixed | While EBM offers advantages like reduced material waste and near-net-shape manufacturing, it also incurs costs for powder material, energy consumption (due to the high-powered electron beam), and potential post-processing like support removal. However, these costs can be offset by reduced machining needs and improved part performance. |
Part Complexity | Positive | EBM excels at creating complex, near-impossible geometries with internal channels and lattices. This unlocks design freedom for lightweight, high-strength components in aerospace, medical, and other industries. The ability to create intricate features can justify the higher costs associated with EBM. |
Produktionsvolym | Begränsad | EBM machines have slower build rates compared to some other additive manufacturing technologies. This makes them less suitable for high-volume production runs. However, EBM shines in applications where customization, small batch sizes, and performance are paramount. |
Materialkompatibilitet | Expanding | EBM can handle a wider range of materials than many other 3D printing processes, including reactive and refractory metals, some alloys, and even certain ceramics. This versatility opens doors for new applications in demanding environments. Continued development of compatible materials will further drive adoption. |
Labor & Expertise | Specialized | Operating and maintaining EBM machines requires skilled personnel with knowledge of vacuum systems, electron beam technology, and metal powder handling. The scarcity of such expertise can be a hurdle for some companies, impacting adoption rates. |
Return on Investment (ROI) | Long-term | The economic viability of EBM hinges on a long-term perspective. While upfront costs are high, the benefits of EBM (e.g., lighter, stronger parts, reduced waste) often translate to cost savings and performance improvements over a product’s lifecycle. |
Government Incentives | Positive | Government support for research and development in additive manufacturing, including EBM, can accelerate adoption by lowering barriers to entry and encouraging innovation. Tax breaks or grants can incentivize companies to invest in EBM technology. |
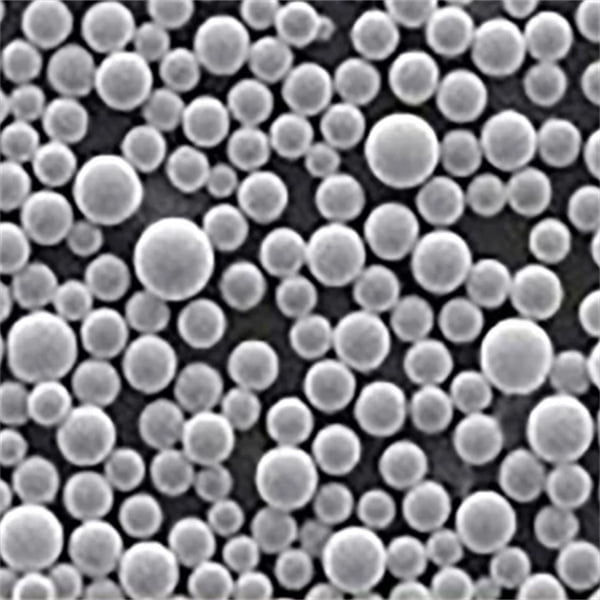
Popular Materials for EBM
Materialklass | Alloy Examples | Fastigheter | Tillämpningar |
---|---|---|---|
Titanlegeringar | Ti-6Al-4V, Ti-6Al-4V ELI | * Excellent strength-to-weight ratio * High biocompatibility * Corrosion resistant | * Aerospace components (wings, landing gear) * Medical implants (knee replacements, dental implants) * Sporting goods (golf clubs, bicycle frames) |
Nickelbaserade legeringar | Inconel 625, Inconel 718 | * Exceptional high-temperature strength * Oxidation and corrosion resistance * Good weldability | * Turbine blades and discs for jet engines * Heat exchangers for chemical processing * Impeller shafts for pumps |
Kobolt-kromlegeringar | CoCrMo, Haynes 214 | * High wear resistance * Biocompatible (limited applications) * Good hardness | * Joint replacements (hips, knees) * Cutting tools and wear parts * Dental implants (limited use due to potential chromium concerns) |
Rostfria stål | 17-4PH, 316L | * Good corrosion resistance * Moderate strength * Biocompatible (limited applications) | * Medical instruments * Chemical processing equipment * Food and beverage processing components |
Emerging Materials | * Tungsten (W) * Tantalum (Ta) * Niobium (Nb) * Molybdenum (Mo) | * Ultra-high melting points * Excellent high-temperature properties * Limited research and development | * Potential applications in aerospace, defense, and nuclear industries (due to high melting points and resistance to extreme environments) |
Benefits of Electron Beam Melting
Förmån | Beskrivning | Påverkan |
---|---|---|
Delar med hög densitet och nära nätform | EBM skapar detaljer med en densitet som överstiger 99,5% av den teoretiska densiteten hos det metallpulver som används. Detta eliminerar porositet (små luftfickor) som är vanligt förekommande i andra additiva tillverkningsmetoder, vilket resulterar i detaljer med exceptionell styrka, utmattningshållfasthet och måttnoggrannhet. | Detta gör det möjligt att skapa funktionella metallkomponenter för krävande tillämpningar inom flyg- (turbinblad, motorhus), medicin- (tandimplantat, proteser) och fordonsindustrin (lätta, högpresterande delar). |
Överlägsna mekaniska egenskaper | Den höga vakuumnivån och den exakta smältprocessen i EBM minimerar oxidation och kontaminering, vilket bevarar metallpulvrets inneboende egenskaper. Detta leder till delar med utmärkt draghållfasthet, krypmotstånd (förmåga att motstå deformation under stress vid höga temperaturer) och brottseghet. | De EBM-tillverkade komponenterna klarar stora belastningar, fungerar effektivt vid förhöjda temperaturer och motstår sprickbildning, vilket gör dem idealiska för applikationer som kräver hållbarhet och strukturell integritet i tuffa miljöer. |
Bearbetning av eldfasta och reaktiva metaller | Till skillnad från traditionella tillverkningsmetoder som begränsas av höga smältpunkter och reaktivitet, är EBM utmärkt för bearbetning av utmanande material som titanlegeringar, tantal och Inconel. Vakuummiljön förhindrar oxidation och möjliggör exakt kontroll av smältprocessen, vilket säkerställer en framgångsrik tillverkning. | Detta breddar designmöjligheterna för branscher som flyg och biomedicin, där komponenterna kräver exceptionella styrke- och viktförhållanden, biokompatibilitet (kompatibilitet med levande vävnad) och hög temperaturprestanda. |
Designfrihet för komplexa geometrier | EBM:s lager-för-lager-metod gör det möjligt att skapa invecklade interna funktioner, kanaler och gitterstrukturer som är omöjliga med konventionella tekniker. Denna designflexibilitet optimerar viktfördelningen, förbättrar värmeöverföringen och gör det möjligt att skapa komponenter med överlägsen funktionalitet. | Denna fördel revolutionerar produktdesignen i branscher som flyg- och rymdindustrin, där lätta, höghållfasta komponenter med komplexa kylkanaler är avgörande för motorns effektivitet. Det gör det också möjligt att skapa skräddarsydda medicinska implantat som passar perfekt till en patients anatomi. |
Minimala stödstrukturer | På grund av den höga hållfastheten hos EBM-tillverkade detaljer behövs minimalt med stödstrukturer under tillverkningsprocessen. Detta minskar efterbearbetningstiden och materialspillet, liksom risken för att stödstrukturerna skadar den slutliga komponenten. | Detta innebär snabbare produktionstider, lägre totalkostnader och möjlighet att skapa intrikata geometrier som skulle vara svåra eller omöjliga att stödja med traditionella metoder. |
Minskat materialspill | EBM är en additiv tillverkningsprocess, vilket innebär att materialet läggs på lager för lager för att skapa den önskade formen. Detta minimerar materialspillet jämfört med subtraktiva tekniker som maskinbearbetning, där en betydande del av råmaterialet tas bort för att uppnå den slutliga formen. | Denna fördel främjar hållbarhet och minskar produktionskostnaderna genom att maximera materialutnyttjandet. Dessutom kan oanvänt pulver från byggkammaren ofta återanvändas för efterföljande byggnationer, vilket ytterligare minimerar avfallet. |
Översikt över electron beam melting machine Leverantörer
A variety of established industrial manufacturers and specialized new entrants provide electron beam melting solutions scalable from research to high volume production across aerospace, medical, automotive, and industrial sectors.
Table 8: Leading Electron Beam Melting System Manufacturers
Leverantör | Detaljer | Target Segments |
---|---|---|
GE Additiv | Pioneered EBM technology | Flyg- och rymdindustrin, medicinteknik, fordonsindustrin |
Sciaky | Largest envelope size | Strukturer för flyg- och rymdindustrin |
Wayland tillsatsmedel | Budget metal AM platforms | Small machine shops |
JEOL | Research grade EBM systems | Universities |
Nano Dimension | Multi-material capabilities | Elektronik, försvar |
Industry leader Arcam EBM, now part of GE Additive, established early leadership thanks to patented solutions and continues dominating the established medical implant and aerospace categories.
Meanwhile, new entrants like Wayland aim to expand adoption by targeting small and medium manufacturers with economical starting platforms.
Collaborations on materials, part qualification, and machine optimization between producers, researchers, and end-user groups will ultimately expand EBM penetration across further critical applications.
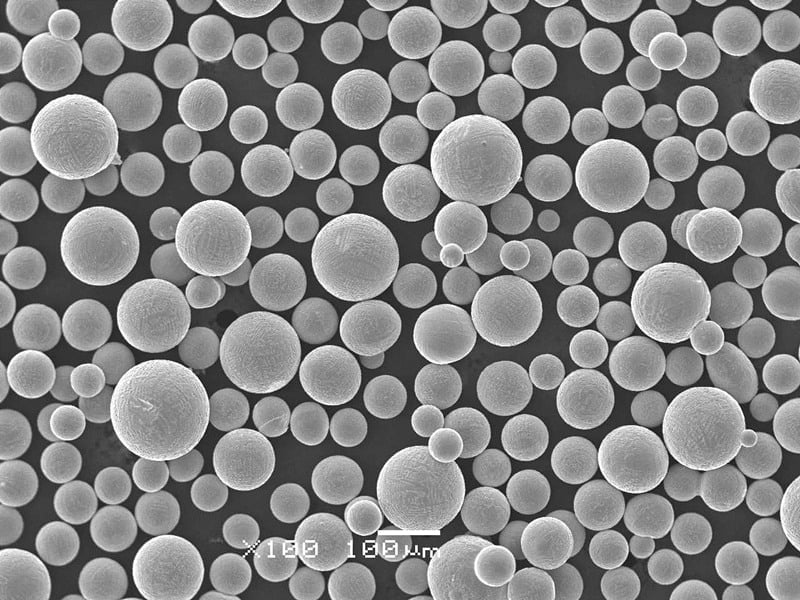
Future Outlook for Electron Beam Melting Adoption
Propelled by superior production speed capabilities plus exceptional mechanical properties impossible with other metal additive or conventional process, EBM adoption appears positioned for massive expansion across aerospace, medical device, automotive, and industrial categories over the next 5-7 years.
Broader awareness of EBM benefits beyond prototyping into full-scale production is expected to drive equipment investments as organizations leverage 3D printing to reshape supply chains.
Larger build envelopes now commercially accessible also enable consolidation of assemblies into fewer components, further optimizing inventory logistics and lead times.
However, declines in system costs combined with increased materials availability must continue improving smaller manufacturers’ access to EBM technology. Streamlining ancillary powder handling tools and post-processing workflows will also simplify adoption.
Overall, EBM sustains strong momentum to penetrate an increasingly wider range of production applications thanks to unmatched deposition rates and exceptional resultant material properties relative to alternate metal additive or legacy fabrication processes.
VANLIGA FRÅGOR
Q: What facility infrastructure is needed to support EBM?
A: Expect 500+ sq. ft for the machine itself, with more space for powder handling stations and post-processing. Concrete floor reinforcement for 12,000+ lb equipment loads is typical.
Q: How many operators per EBM machine are required?
A: One technician can support several EBM units depending on automation level and production volumes. Additional staff handles powder operations, post-processing tasks, maintenance, and engineering.
Q: What materials cannot be processed with EBM technology?
A: Non-conductive polymers cannot be processed with electron beams. But EBM accommodates practically any conductive metal alloy system otherwise manufacturable.
Q: What safety risks are associated with EBM technology?
A: High power electron beam voltages pose arc flash risks requiring proper enclosures and controls. Reactive metal powder exposure also requires protocols for fire and inhalation dangers necessitating protective equipment and training.
Q: Does EBM require any secondary heat treatment?
A: Certain alloys benefit from heat treatment to further enhance microstructures and tailor mechanical properties. However, the rapid solidification cycles and high pre-heat temperatures intrinsic to the EBM process typically eliminate these post-processing steps.
Dela på
Facebook
Twitter
LinkedIn
WhatsApp
E-post
MET3DP Technology Co, LTD är en ledande leverantör av lösningar för additiv tillverkning med huvudkontor i Qingdao, Kina. Vårt företag är specialiserat på 3D-utskriftsutrustning och högpresterande metallpulver för industriella tillämpningar.
Förfrågan för att få bästa pris och anpassad lösning för ditt företag!
Relaterade artiklar
december 18, 2024
Inga kommentarer
december 17, 2024
Inga kommentarer
Om Met3DP
Senaste uppdateringen
Vår produkt
KONTAKTA OSS
Har du några frågor? Skicka oss meddelande nu! Vi kommer att betjäna din begäran med ett helt team efter att ha fått ditt meddelande.
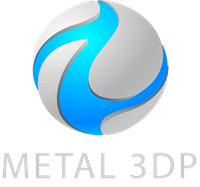
Metallpulver för 3D-printing och additiv tillverkning
FÖRETAG
PRODUKT
cONTACT INFO
- Qingdao City, Shandong, Kina
- [email protected]
- [email protected]
- +86 19116340731