Inductively Coupled Plasma (ICP)
Table of Contents
Imagine a miniature sun, blazing with intense heat but safely contained within a sophisticated instrument. This fiery heart of science isn’t science fiction; it’s the reality of inductively coupled plasma (ICP). ICP technology plays a crucial role in various scientific and industrial fields, and today, we’ll delve into its fascinating world.
What is Inductively Coupled Plasma (ICP)?
ICP, also known as Transformer Coupled Plasma (TCP), is a unique type of plasma source. But what exactly is plasma? Imagine a state of matter even hotter than gas, where atoms are stripped of some or all their electrons, creating a sea of charged particles. In ICP, this high-temperature plasma is generated through a clever trick: electromagnetic induction.
Here’s the magic behind it:
- A rapidly oscillating electric current flows through a cooled induction coil.
- This current creates a rapidly changing magnetic field around the coil.
- This magnetic field, in turn, induces an electric current within a flowing stream of gas, typically argon.
- The induced current heats the gas to incredibly high temperatures, exceeding 6,000 degrees Celsius, transforming it into plasma.
Think of it this way: Imagine swirling a metal whisk rapidly within a strong magnet. The whisk heats up due to the induced current, just like the gas in the ICP torch.
This high-temperature plasma offers a powerful tool for various analytical techniques. But before we explore its applications, let’s understand the different types of ICP and their characteristics.
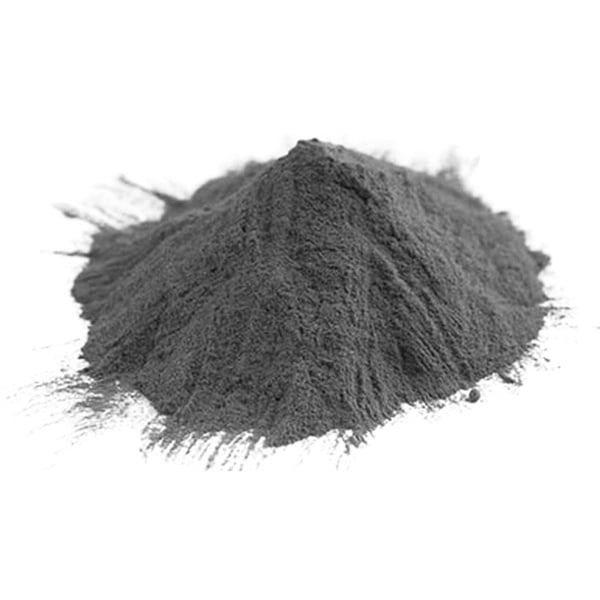
Types of ICP
The world of ICP isn’t a one-size-fits-all situation. Here’s a breakdown of the most common types:
- Inductively Coupled Plasma Optical Emission Spectroscopy (ICP-OES): This workhorse technique uses the excited state of elements within the plasma. As these elements return to their ground state, they emit light at specific wavelengths. By analyzing this light, scientists can identify and quantify the elemental composition of a sample.
- Inductively Coupled Plasma Mass Spectrometry (ICP-MS): Here, the hot plasma efficiently ionizes elements in the sample. These ions are then separated and measured based on their mass-to-charge ratio by a mass spectrometer. ICP-MS offers exceptional sensitivity, allowing detection of trace elements present in minute quantities.
- Inductively Coupled Plasma Reactive Ion Etching (ICP-RIE): This technique utilizes the highly reactive plasma to etch patterns onto various materials. The plasma can precisely remove material layer by layer, making it a valuable tool in microelectronics fabrication.
Choosing the Right ICP: Selecting the appropriate ICP type depends on your analytical needs. ICP-OES excels at elemental identification and quantification, while ICP-MS shines in detecting trace elements. ICP-RIE, on the other hand, is your go-to for precise material etching.
Applications of ICP
ICP technology isn’t just a scientific showstopper; it has a wide range of practical applications across various industries. Let’s explore some key areas where ICP plays a vital role:
Environmental Monitoring: ICP is a powerful tool for analyzing environmental samples like soil, water, and air. It can detect and quantify trace elements like heavy metals, pollutants, and even nutrients, providing crucial insights into environmental health.
Material Science: From analyzing the composition of alloys and semiconductors to identifying impurities in advanced materials, ICP is a workhorse in material science research and development. It helps ensure material quality and optimize material properties for specific applications.
Forensics and Trace Evidence Analysis: ICP-MS, with its exceptional sensitivity, plays a critical role in forensic science. It can analyze minute traces of elements left behind at crime scenes, helping investigators link suspects to evidence and identify the source of materials.
Food and Pharmaceutical Analysis: Ensuring food safety and the quality of pharmaceutical products is paramount. ICP helps analyze the elemental composition of food and drugs, detecting contaminants, verifying the presence of essential elements, and ensuring product safety and efficacy.
Biological Research: ICP offers valuable insights into biological processes. It can analyze the elemental composition of tissues, fluids, and even single cells, helping researchers understand the role of trace elements in various biological functions.
These are just a few examples, and the applications of ICP continue to expand as scientists unlock its full potential.
Metal Powders for ICP Analysis
Now, let’s delve into the world of metal powders specifically used for ICP analysis. These carefully designed powders offer several advantages:
- Improved Accuracy: Metal powders with high purity and homogeneous composition minimize measurement errors, leading to more reliable results.
- Enhanced Sensitivity: Fine and uniform metal powders dissolve more efficiently within the plasma, maximizing the number of atoms available for analysis, which translates to better detection limits for trace elements.
Metal Powders for ICP Analysis
Here’s a closer look at some specific metal powder models commonly used in ICP analysis, along with their key characteristics:
1. High-Purity Titanium Powder (Grade 2):
- Description: This metal powder boasts a minimum titanium content of 99.2% with low levels of impurities like iron, oxygen, and nitrogen.
- Advantages: Excellent choice for analyzing titanium alloys and identifying trace elements due to its high purity. Fine particle size ensures efficient dissolution in the plasma, enhancing sensitivity.
- Disadvantages: May be slightly more expensive compared to some other metal powders.
2. Iron Powder (Reduced):
- Description: This iron powder undergoes a reduction process to minimize oxide content, typically below 1%.
- Advantages: Cost-effective option for analyzing iron and steel samples. Good sensitivity for detecting major elements in iron-based materials.
- Disadvantages: Higher oxygen content compared to high-purity iron powders might affect accuracy for trace element analysis.
3. Copper Powder (Electrolytic):
- Description: Produced through an electrolytic refining process, this copper powder boasts high purity exceeding 99.5% copper content.
- Advantages: Excellent for analyzing copper alloys and identifying trace elements due to its minimal impurities. Fine and uniform particle size enhances dissolution efficiency.
- Disadvantages: Slightly higher cost compared to some other metal powder options.
4. Nickel Powder (Spherical):
- Description: This unique nickel powder features spherical particles for improved flowability and packing density.
- Advantages: Spherical shape minimizes surface area, reducing potential oxidation during storage. Offers good packing characteristics for sample preparation, leading to more consistent results.
- Disadvantages: May be slightly more expensive compared to irregularly shaped nickel powders.
5. Aluminum Powder (High Purity):
- Description: This aluminum powder offers a minimum aluminum content of 99.7%, ideal for analyzing aluminum alloys and composites.
- Advantages: High purity minimizes interference from impurities during analysis. Fine particle size ensures efficient dissolution for improved sensitivity.
- Disadvantages: Aluminum is highly reactive and requires careful handling to prevent oxidation, which can affect analysis accuracy.
6. Cobalt Powder (Carbonyl):
- Description: Produced via the carbonyl process, this cobalt powder boasts exceptional purity exceeding 99.9%.
- Advantages: Unmatched purity makes it ideal for analyzing high-purity cobalt materials and detecting trace elements at very low concentrations.
- Disadvantages: The high purity and production process often translate to a higher cost compared to other metal powders.
7. Tin Powder (Reduced):
- Description: This tin powder undergoes a reduction process to minimize oxide content, typically below 2%.
- Advantages: Cost-effective option for analyzing tin alloys and solders. Good sensitivity for detecting major elements in tin-based materials.
- Disadvantages: Higher oxygen content compared to high-purity tin powders might affect accuracy for trace element analysis.
8. Molybdenum Powder (Reduced):
- Description: This molybdenum powder undergoes a reduction process to minimize oxide content, typically below 1%.
- Advantages: Relatively cost-effective choice for analyzing molybdenum alloys and steels. Good sensitivity for detecting major elements in molybdenum-containing materials.
- Disadvantages: May not be suitable for high-precision analysis of trace elements due to potential interference from oxygen.
9. Silver Powder (High Purity):
- Description: This silver powder boasts a minimum silver content exceeding 99.9%, making it ideal for analyzing high-purity silver materials and jewelry.
- Advantages: Exceptional purity minimizes interference from impurities for accurate analysis. Fine particle size ensures efficient dissolution in the plasma.
- Disadvantages: High purity and production process often result in a higher cost compared to other metal powders.
10. Mixed Metal Calibration Standards:
- Description: These pre-mixed powders combine various elements in known concentrations, serving as reference materials for ICP calibration.
- Advantages: Essential for ensuring the accuracy and consistency of ICP measurements. Available in various compositions to suit specific analytical needs.
- Disadvantages: May be slightly more expensive compared to single-element metal powders.
Choosing the Right Metal Powder:
Selecting the most suitable metal powder for your ICP analysis depends on several factors:
- The elements you’re analyzing: Ensure the powder’s composition minimizes spectral interference from the matrix.
- Desired level of accuracy: High-purity powders offer better accuracy for trace element analysis.
- Cost considerations: Balance the cost of the powder with the required level of accuracy and sensitivity for your application.
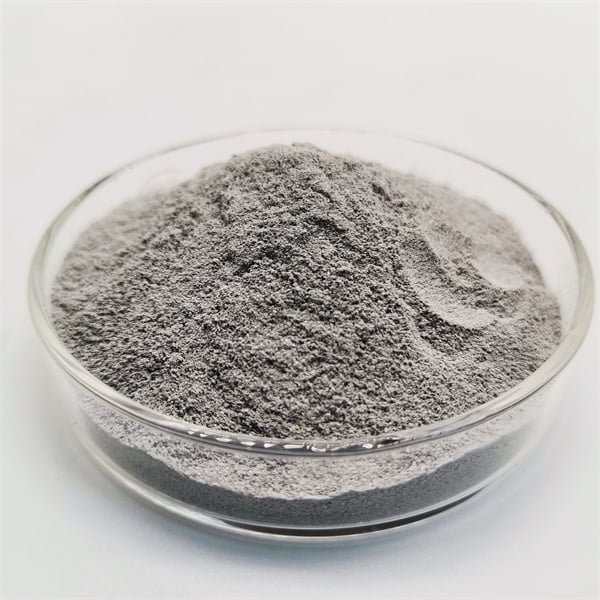
Advantages and Limitations of ICP
ICP technology offers a powerful analytical toolkit, but like any tool, it has its own set of advantages and limitations. Understanding these aspects is crucial for maximizing its effectiveness and interpreting results accurately.
Advantages of ICP
- High Sensitivity: ICP excels at detecting trace elements present in minute quantities. This makes it ideal for analyzing environmental samples, high-purity materials, and biological tissues.
- Wide Elemental Range: ICP can analyze a vast range of elements from the lightest (lithium) to the heaviest (uranium). This versatility makes it a valuable tool for diverse analytical applications.
- Fast Analysis Times: ICP analyses are typically completed within minutes, making it a time-efficient technique for routine analysis.
- Minimal Sample Preparation: ICP analysis often requires minimal sample preparation compared to other techniques, reducing analysis time and potential errors.
- Multi-Element Analysis: ICP can simultaneously analyze multiple elements within a single sample, providing a comprehensive elemental profile.
- Excellent Detection Limits: ICP boasts exceptional detection limits, allowing scientists to identify trace elements present in very low concentrations.
These advantages make ICP a highly versatile and powerful analytical tool across various scientific disciplines.
Limitations of ICP
While ICP offers significant benefits, it’s important to acknowledge its limitations to ensure proper interpretation of results:
- Interference: Spectral overlap from certain elements can interfere with the analysis of other elements present in the sample. Careful selection of analytical conditions and background correction techniques are crucial to mitigate this issue.
- Limited Speciation Capabilities: ICP primarily identifies the total elemental content, not the specific chemical form (oxidation state) of the element. Additional techniques might be required for speciation analysis.
- Cost: ICP instruments are relatively expensive compared to some simpler analytical techniques. However, the versatility and efficiency of ICP often justify the initial investment for high-throughput analysis needs.
- Sample Introduction: ICP analysis typically requires the sample to be dissolved in a liquid solvent. This might not be suitable for analyzing certain types of samples like solids or organic materials.
By understanding these limitations, scientists can choose the most appropriate analytical techniques for their specific needs and interpret ICP results with proper caution.
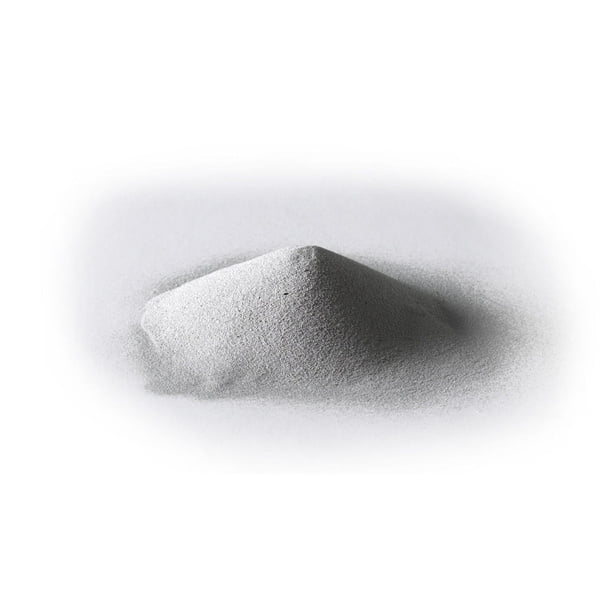
ICP vs. Other Analytical Techniques
When faced with an analytical challenge, ICP isn’t always the only option. Here’s a brief comparison of ICP with some other commonly used techniques:
- Atomic Absorption Spectroscopy (AAS): AAS shares some similarities with ICP but offers lower detection limits for certain elements. However, AAS is a single-element technique, while ICP can analyze multiple elements simultaneously.
- X-Ray Fluorescence (XRF): XRF is a non-destructive technique that excels at analyzing the elemental composition of solid samples. However, XRF generally has lower sensitivity for trace elements compared to ICP.
- Mass Spectrometry (MS): MS offers exceptional sensitivity and versatility, similar to ICP-MS. However, MS instruments are typically more expensive and require more specialized expertise compared to ICP.
The choice between ICP and other techniques depends on the specific analytical needs, including the elements of interest, required sensitivity, sample type, and budget constraints.
The Evolving Landscape of ICP
ICP technology is constantly evolving, with advancements in instrumentation, methodology, and data analysis capabilities. Here are some exciting trends shaping the future of ICP:
- Miniaturization of ICP instruments: Portable and handheld ICP devices are being developed, offering greater flexibility for on-site analysis in diverse environments.
- Improved Detection Limits: Advancements in technology are pushing detection limits even lower, allowing scientists to identify elements present in vanishingly small quantities.
- Automated Sample Preparation Systems: Automated systems are streamlining sample preparation workflows, making ICP analysis faster and more efficient.
- Development of New Applications: Researchers are constantly exploring new applications for ICP, expanding its reach into various scientific fields.
These advancements promise to solidify ICP’s position as a vital analytical tool for the foreseeable future.
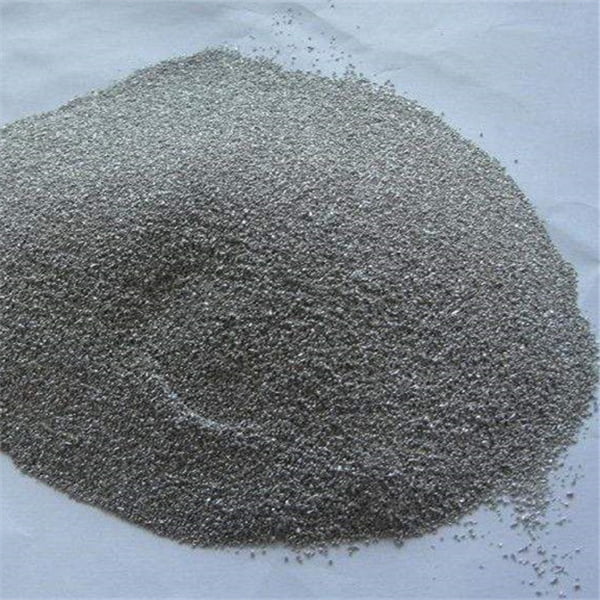
FAQ
Q: What safety precautions should be taken when working with ICP?
A: ICP systems operate at high temperatures and pressures. Here are some crucial safety precautions:
- Always wear appropriate personal protective equipment (PPE): This includes safety glasses, gloves, and lab coat to protect yourself from potential splashes or hot materials.
- Maintain proper ventilation: ICP instruments generate fumes, so ensure adequate ventilation within the laboratory space.
- Be aware of electrical hazards: ICP systems use high voltage electricity. Only trained personnel should operate the instrument and follow proper safety protocols.
- Handle hazardous materials with care: Many samples analyzed by ICP might contain hazardous elements. Proper disposal procedures must be followed to minimize environmental and health risks.
Q: How often does ICP equipment require calibration?
A: The frequency of ICP calibration depends on several factors, including the specific instrument, analytical requirements, and usage patterns. Generally, calibration is recommended:
- At regular intervals: This could be daily, weekly, or monthly, depending on the instrument and usage frequency.
- After maintenance procedures: Any maintenance or repairs performed on the instrument might necessitate recalibration to ensure accuracy.
- When switching between different sample types: Switching from analyzing one type of material to another might require recalibration to account for potential matrix effects.
Consulting the instrument manufacturer’s recommendations and following established laboratory protocols are crucial for determining the appropriate calibration frequency for your ICP system.
Q: How can I interpret the results of an ICP analysis?
A: ICP analysis reports typically include a table listing the elements detected in the sample and their corresponding concentrations. However, proper interpretation requires additional considerations:
- Detection limits: The report should specify the detection limits for each element. Elements reported below the detection limit might be present but not quantifiable.
- Matrix effects: The presence of other elements in the sample can interfere with the analysis of certain elements. Background correction techniques are often employed to minimize these interferences.
- Quality control data: The analysis report should include data from quality control samples to assess the accuracy and precision of the measurements.
Consulting a qualified analyst or chemist experienced with ICP is recommended for comprehensive interpretation of ICP results, especially when dealing with complex samples or critical analytical data.
Q: Are there any online resources for learning more about ICP?
A: Absolutely! Here are some helpful resources to delve deeper into the world of ICP:
These resources can provide valuable insights into the theory, operation, and applications of ICP technology.
Share On
Facebook
Twitter
LinkedIn
WhatsApp
Email
MET3DP Technology Co., LTD is a leading provider of additive manufacturing solutions headquartered in Qingdao, China. Our company specializes in 3D printing equipment and high-performance metal powders for industrial applications.
Inquiry to get best price and customized Solution for your business!
Related Articles
November 14, 2024
No Comments
November 14, 2024
No Comments
About Met3DP
Play Video
Recent Update
Our Product
CONTACT US
Any questions? Send us message now! We’ll serve your request with a whole team after receiving your message.
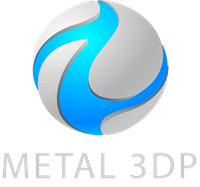
Metal Powders for 3D Printing and Additive Manufacturing
COMPANY
PRODUCT
cONTACT INFO
- Qingdao City, Shandong, China
- [email protected]
- [email protected]
- +86 19116340731